Jump to a key chapter
Definition of Machining Dynamics
Machining dynamics is a key concept in manufacturing processes, focusing on the **interaction between machines, cutting processes, and the materials being worked on**. This field of study examines the **vibrations and forces** involved in the machining process, which can affect the surface finish, tool life, and overall efficiency of the manufacturing operation. Understanding machining dynamics is essential for improving the quality and precision of machined parts.
Basic Principles of Machining Dynamics
The study of machining dynamics involves several fundamental principles:
- Vibrations: These are oscillations that occur during the cutting process, caused by interaction between the tool and workpiece.
- Stability: It is essential to maintain a stable machining environment to prevent excessive vibrations or chatter.
- Cutting Force: Force exerted by the tool on the workpiece, influencing the cutting performance.
- Material Properties: Material's strength, hardness, and other characteristics affect dynamic behavior.
Cutting Force Coefficient is a pivotal parameter in machining dynamics. It defines the relationship between applied force and material removal rate. Mathematically, it is expressed as \[ F = K_c \cdot A \cdot v \] where \[ F \] is the cutting force, \[ K_c \] is the cutting force coefficient, \[ A \] is the cross-sectional area of cut, and \[ v \] is the cutting speed.
Consider a scenario where the cutting force coefficient \[ K_c \] is determined experimentally to be 1500 N/mm2. For a cut with a cross-sectional area \[ A = 2 \text{ mm}^2 \] and cutting speed \[ v = 200 \text{ mm/s} \], the cutting force \[ F \] can be calculated as follows: \[ F = 1500 \cdot 2 \cdot 200 = 600,000 \text{ N} \] This example illustrates how modifying any of the parameters can significantly impact the cutting force.
Machining dynamics often involves the study of Chatter, a self-excited vibration that occurs during machining and leads to poor surface finish and possible tool or machine damage. Chatter arises from various factors such as spindle speed, tool geometry, and material properties. To analyze chatter, researchers employ models like the Standby Variable Model and the Mode Coupling Model, which consider how vibrations interact with machining dynamics. One method to reduce chatter involves optimizing system parameters, such as altering spindle speed or adjusting tool stiffness, often by trial and error or dynamic response simulations. These adjustments aim to bring the system residual energy to a minimum, thereby minimizing chatter.
Reducing chatter can not only improve surface finish but also extend the life of cutting tools significantly.
Understanding Machining Dynamics
Machining dynamics involves the study of interactions between the machining system, tool, and workpiece. The analysis of these interactions is crucial to optimize machining processes, reduce unwanted vibrations, and improve the quality of the final product.
Fundamental Concepts
Key aspects of machining dynamics include:
- Vibrations: They are crucial as they determine the stability and quality of the machining process.
- Forces: Cutting forces impact tool wear, production times, and surface finish.
- Materials: The properties of the workpiece material, such as hardness and elasticity, influence machining behavior.
Machining Dynamics: This refers to the study of forces and vibrations generated during machining processes, which influence the surface finish, dimensional accuracy, and tool life.
Imagine a scenario where you are machining a titanium component. This material's high strength and hardness present challenges, resulting in significant tool wear and vibrations. By adjusting the cutting speed and feed rate, vibrations can be minimized, enhancing tool life and surface finish.
Chatter is a common phenomenon in machining dynamics, characterized by self-excited vibrations due to energy feedback from the machining process. To mitigate chatter:
- Optimize spindle speed and feed rate.
- Use high-damping tools and fixtures.
- Employ variable pitch tools for balancing forces.
Experimenting with different cutting parameters can reveal optimal conditions to minimize chatter and enhance machining efficiency.
Machining Dynamics Techniques
The study and application of machining dynamics techniques are crucial for optimizing machining processes in modern manufacturing. These techniques focus on understanding and controlling the dynamic interactions between cutting tools and workpieces.
Vibration Analysis in Machining
A significant part of machining dynamics is the analysis of vibrations. There are several techniques employed for effective vibration management:
- Tuned Mass Dampers: Used to absorb and counter undesired oscillations.
- Dynamic Absorbers: Attached to the machine to modify its vibratory response.
- Frequency Response Function (FRF): Helps in determining the stability of machining parameters.
Frequency Response Function (FRF) is a quantifiable measure of a system’s output spectrum in response to an input signal. In machining, it characterizes the structural properties of the tool and workpiece setup, usually denoted as a complex function of frequency.
Consider using a Tuned Mass Damper in a milling operation. If a tool experiences excessive vibrations at a frequency of 200 Hz, a damper can be precisely tuned to this frequency to significantly reduce unwanted oscillations, stabilizing the machining process.
In-depth analysis of machining dynamics involves studying mode shapes and frequencies through comprehensive models like Finite Element Analysis (FEA). By modeling the entire machining setup, including the tool, workpiece, and machine frame, you gain insights into potential resonance issues. Advanced simulations can account for different operating conditions, predicting responses under various loads and speeds. Embedding sensors into machining setups aids in real-time monitoring, providing data that feedback to algorithms for dynamic adjustment of machining parameters. The use of these sophisticated techniques offers a profound impact on improving the precision and efficiency of machining operations over extensive periods.
Regularly calibrating your equipment and updating software systems can enhance the effectiveness of vibration control measures.
Examples of Machining Dynamics
The application of machining dynamics can be understood through various examples that demonstrate how these principles improve manufacturing processes. By analyzing vibration and forces, one can optimize machining operations effectively.
Machining Dynamics Explained in Simple Terms
Machining dynamics refers to the study of the material removal process where forces and vibrations are predominant. In simpler terms, it seeks to understand how tools and workpieces interact dynamically during the machining process, ultimately influencing performance.
In machining dynamics, you often encounter terms like natural frequency, which is the frequency at which a system oscillates in the absence of external forces. The formula for calculating natural frequency \(f_n\) is given by: \[ f_n = \frac{1}{2\pi} \sqrt{\frac{k}{m}} \] where \(k\) is the stiffness of the system, and \(m\) is the mass. This equation highlights the significance of material properties on dynamic responses.Moreover, understanding modal analysis and modelling using computational tools can enhance the prediction of dynamic behavior in machining processes. Tools like MATLAB or ANSYS are often employed to simulate these dynamic interactions and validate experimental data, advancing the field's theoretical knowledge.
Consider a milling operation where you analyze tool path strategies to minimize chatter. By altering spindle speeds and implementing variable pitch cutters, you observe a reduction in vibration levels, resulting in a smoother surface finish and prolonged tool life.
Adjusting the spindle speed can move the tool away from the resonance frequency, reducing unwanted vibrations and improving machining stability.
Key Concepts in Machining Dynamics
Several key concepts form the foundation of machining dynamics:
- Chatter: Unwanted self-excited vibrations that can occur at certain frequencies.
- Stability Lobes: Graphical representations showing stable and unstable cutting zones based on speed and depth of cut.
- Damping: The mechanism of dissipating vibratory energy to mitigate oscillations.
Stability Lobes diagram represents the boundaries between stable and unstable regions in terms of speed and depth of cut during the machining process. The lobes indicate optimal processing windows for a particular setup.
Application of Machining Dynamics Techniques
Machining dynamics techniques can be applied in various manufacturing scenarios to enhance productivity and quality. Some common applications include:
- Vibration Analyzers: These tools help detect and analyze unwanted vibrations, providing real-time feedback for system adjustments.
- Tool Condition Monitoring: Implementing sensors and algorithms to track tool wear and predict maintenance schedules.
- Process Optimization: Adjusting cutting parameters based on dynamic analysis to reduce machining time without sacrificing quality.
Incorporating software solutions for monitoring dynamic parameters can result in substantial savings by avoiding downtime and extending tool life.
Real-World Examples of Machining Dynamics
Real-world implementations of machining dynamics provide insights into its practical benefits:
- Automotive Industry: Enhancing engine components production by reducing cycle times through controlled vibration and optimal cutting settings.
- Aerospace Sector: Achieving tight tolerances on critical components using stability lobe analysis to avoid chatter.
- Tool Manufacturing: Employing dynamic force modeling to design advanced cutting tools that minimize vibration and maximize lifespan.
In the aviation sector, the production of turbine blades requires extreme precision, often leveraging machining dynamics principles. Vibration control technologies, such as active dampers, are integrated into machinery to stabilize cutting processes dynamically. Additionally, the exploration of adaptive control systems, where sensors feed real-time data into control algorithms, optimizes cutting conditions instantaneously. By doing so, manufacturers not only improve quality but also significantly reduce the cost associated with tool wear and machine downtime, aligning with sustainability goals in high-stakes manufacturing environments.
machining dynamics - Key takeaways
- Machining Dynamics Definition: The study of interactions between machines, cutting processes, and materials, focusing on vibrations and forces that affect manufacturing efficiency and quality.
- Key Principles: Vibrations, stability, cutting force, and material properties are fundamental in understanding machining dynamics.
- Cutting Force Coefficient: It relates to the force level needed for material removal, defined by the formula \( F = K_c \cdot A \cdot v \).
- Chatter: A self-excited vibration that affects machining precision, which can be mitigated through speed adjustment and tool stiffness optimization.
- Machining Dynamics Techniques: Techniques such as tuned mass dampers, dynamic absorbers, and frequency response function (FRF) help control and predict vibrations.
- Application Examples: Utilizing machining dynamics in automotive and aerospace industries enhances production by reducing vibrations and optimizing conditions.
Learn faster with the 24 flashcards about machining dynamics
Sign up for free to gain access to all our flashcards.
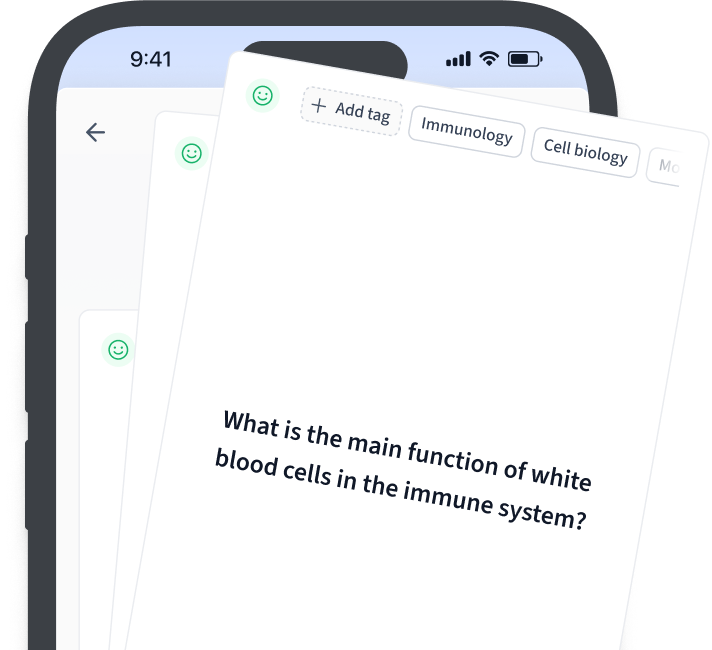
Frequently Asked Questions about machining dynamics
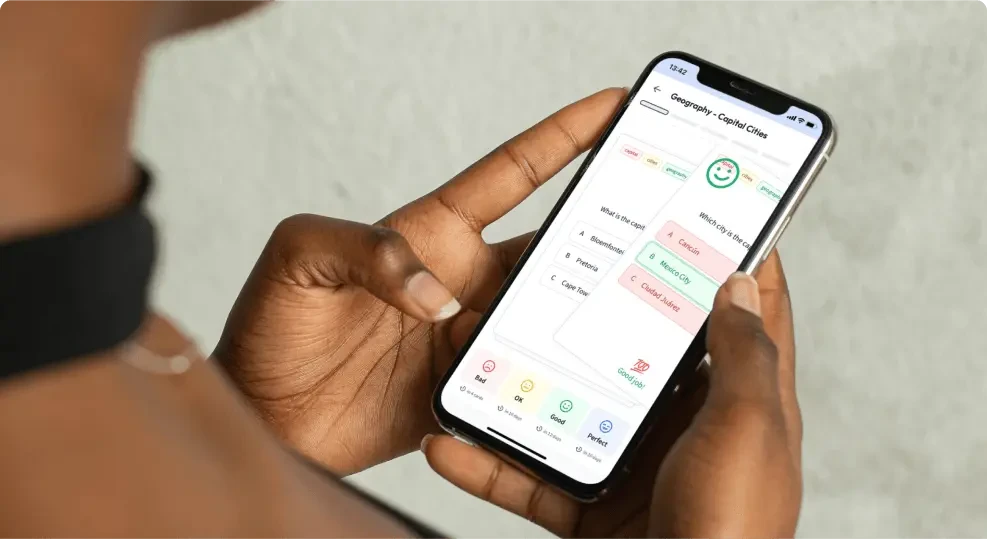
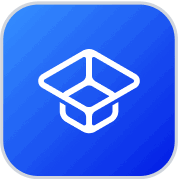
About StudySmarter
StudySmarter is a globally recognized educational technology company, offering a holistic learning platform designed for students of all ages and educational levels. Our platform provides learning support for a wide range of subjects, including STEM, Social Sciences, and Languages and also helps students to successfully master various tests and exams worldwide, such as GCSE, A Level, SAT, ACT, Abitur, and more. We offer an extensive library of learning materials, including interactive flashcards, comprehensive textbook solutions, and detailed explanations. The cutting-edge technology and tools we provide help students create their own learning materials. StudySmarter’s content is not only expert-verified but also regularly updated to ensure accuracy and relevance.
Learn more