Jump to a key chapter
Wind Energy Applications in Engineering
Wind energy has rapidly become a cornerstone in the pursuit of renewable energy sources. As an engineering student, you have the opportunity to familiarize yourself with its diverse applications. Understanding how wind energy systems function, exploring various techniques, and delving into the underlying theories are crucial components of your study.
How Wind Energy Applications Work in Engineering Systems
Wind energy systems harness the natural movement of air to generate electricity. In essence, these systems convert kinetic energy from the wind into mechanical energy, which is subsequently transformed into electrical energy. This process involves several key components:
- Turbines: Wind turbines capture wind's kinetic energy. Their blades rotate, driving the shaft connected to a generator.
- Generator: Converts mechanical energy from the turbine into electrical energy.
- Transformer: Steps up the generated electricity to a higher voltage for efficient transmission over power lines.
Kinetic Energy: The energy an object possesses due to its motion, defined in physics as \( KE = \frac{1}{2}mv^2 \), where \( m \) is mass and \( v \) is velocity.
The efficiency of wind turbines improves significantly at higher altitudes where wind speeds are stronger.
Technical Techniques of Wind Energy Applications
In the realm of engineering, multiple techniques are employed to enhance the use of wind energy. A successful application requires optimizing turbine design and site selection:
- Blade Design: Modern turbines feature aerodynamically optimized blades to maximize energy capture. The blade's shape is crucial for balancing lift and drag forces.
- Siting: Geographic location plays an important role. Engineers often use wind maps to determine high wind speed areas.
- Control Systems: Advanced control mechanisms adjust the blade position and generator operations to optimize output and safety.
Simulation tools such as Computational Fluid Dynamics (CFD) allow for detailed analyses of airflow over turbine blades, enhancing both design simplicity and efficiency. CFD models solve Navier-Stokes equations to accurately depict fluid flow behaviors, a cornerstone concept in thermodynamic and fluid mechanics fields. Understanding these equations and their applications extends beyond just wind energy, offering insights into various engineering challenges.
Wind Energy Explained: Theory, Design, and Application
The theory of wind energy involves understanding how fluid dynamics and physics principles contribute to energy extraction. Designing efficient systems requires a grasp of several complex concepts:
- Betz Limit: A theoretical limit dictating the maximum efficiency of a wind turbine, stating that no turbine can capture more than 59.3% of the kinetic energy in wind.
- Aerodynamics: Key to blade design, involves principles like lift ( lift = \( L = Cl \frac{1}{2} \rho U^2 A \)) and drag.
- Material Science: Advanced materials like carbon fiber and composites are used for lightweight, strong blades.
Consider a wind turbine in a region with an average wind speed of 10 m/s. Using the power equation, \( P = \frac{1}{2} \rho A v^3 \), you can estimate the power output by calculating \( P \) with \( \rho \) as air density and \( A \) as the area swept by the blades.
Practical Exercises on Wind Energy in Engineering
Engaging in practical exercises is crucial for solidifying your understanding of wind energy applications. Here are some exercises you might find valuable:
- Create a Wind Map: Use available meteorological data to map average wind speeds in your region.
- Design a Blade Model: Using CAD software, design a turbine blade considering aerodynamic principles.
- Simulation: Employ software to simulate wind flow over different terrains to determine potential wind farm sites.
- Build a Mini Turbine: Assemble a small-scale model using common materials, measuring its energy output under various wind conditions.
Practical experiences often provide more insight than theoretical study alone. Always aim to engage both aspects for a comprehensive understanding.
Examples of Wind Energy Applications in Engineering Projects
The impact of wind energy on engineering projects is both profound and widespread, offering sustainable solutions across multiple sectors. Understanding these applications reveals the versatility of wind technologies, critical for your journey in engineering.
Various Applications of Wind Energy
Wind energy applications include diverse implementations that harness wind power to meet energy demands. A glance at their widespread usage will illuminate their significance in engineering.
- Electrical Power Generation: Large-scale wind farms convert wind energy into electricity, providing power to entire regions.
- Offshore Wind Farms: Take advantage of stronger, more consistent winds at sea, often yielding higher energy returns.
- Remote Power Solutions: Small wind turbines cater to off-grid locations, powering homes in isolated areas.
- Agricultural Applications: Wind turbines are used to operate water pumps for irrigation and other farming needs.
Consider the application of a small wind turbine system in a rural area, which provides electricity to homes without access to the central power grid. By using an average wind speed of 5 m/s, a small turbine with a rotor diameter of 2 meters can yield a power output calculated using \( P = 0.5 \times \rho \times A \times v^3 \), where \( v \) is the wind speed.
Betz Limit: An important concept in wind turbine efficiency, indicating that no turbine can convert more than 59.3% of the wind's kinetic energy into mechanical energy.
The importance of offshore wind farms cannot be overstated. These projects face less land use competition and ecological impact, offering an advantage over onshore sites. Additionally, wind speeds are generally higher over water due to the absence of obstructions, which leads to increased energy output. However, the installation and maintenance of offshore wind turbines pose logistical challenges requiring robust engineering solutions. Structural materials and designs must adequately withstand harsh marine environments, including high winds, saltwater corrosion, and wave forces.
Offshore wind farms are closer to urban areas, reducing transmission distance and increasing efficiency in power delivery.
Case Studies in Engineering Projects
Exploring case studies showcases the practical utilization of wind energy in real-world engineering projects. These examples demonstrate both the successes and the challenges that come with integrating wind power:
- Horns Rev Wind Farm (Denmark): One of the world's first large-scale offshore wind farms, it serves as a model for subsequent projects worldwide.
- Shepherds Flat Wind Farm (USA): A significant example of onshore wind energy application, it combines over 300 turbines to generate an impressive 845 MW of electricity.
- Alta Wind Energy Center (USA): Highlights advancements in generating wind power with improved turbine designs and grid integration strategies.
Horns Rev Wind Farm faced numerous challenges such as harsh weather conditions and construction on the North Sea's seabed. These issues required innovative solutions, including monopile foundations, which provided stability for the turbines.
Innovations in Wind Energy Applications
Innovations continue to drive the evolution of wind energy applications, with a focus on improving efficiency, reducing costs, and widening accessibility. Notable innovations include:
- Floating Wind Turbines: Enable placement of turbines in deep ocean areas where winds are stronger.
- Advanced Materials: Development of lightweight composites increases blade efficiency and durability.
- Smart Grids: Integrate renewable energy sources while ensuring stable energy distribution.
- Energy Storage Solutions: Address the intermittent nature of wind energy by leveraging battery storage and new technologies like pumped hydroelectric storage.
Floating wind turbines represent a transformative development in wind energy, opening up new areas for power generation far from the coastlines. They use mooring systems similar to those in the oil and gas industry, allowing turbines to be stationed in water depths of over 700 meters. This helps leverage higher and more consistent wind speeds found in deep waters, significantly increasing their efficiency.
Technical Techniques of Wind Energy Applications
Understanding technical techniques in wind energy applications is essential for those interested in harnessing wind power. These techniques are crucial for optimizing the design and improving the efficiency of wind energy systems, ensuring that they meet both current and future energy demands effectively.
Tools and Materials for Wind Energy Projects
Wind energy projects require a diverse range of tools and materials to effectively convert wind into usable energy. The choice of materials and tools can significantly impact the performance and longevity of the wind turbines.
- Materials: Modern wind turbines rely on robust materials such as carbon fiber and glass-reinforced plastics for their blades, ensuring they can withstand the forces exerted by the wind.
- Structure Components: Key materials include steel for the tower and nacelle housing, giving the turbine structural integrity.
- Tools: Engineers employ various diagnostic and design tools, such as CAD software, to model and optimize the shape and placement of turbine components.
To construct a reliable wind turbine, consider the use of fiberglass for blades due to its strength-to-weight ratio, which is critical for enabling large blade designs that maximize energy capture. A simple calculation of blade material influence could start with the blade's mass related to its build-up materials, quantified by the equation \( m = \rho V \), where \( \rho \) is density and \( V \) is volume.
The advent of nano-materials marks a significant advance in wind turbine technology. These materials offer enhanced aerodynamic properties, higher efficiency, and greater durability. Recent innovations include the use of carbon nanotubes and graphene in blade coatings, which reduce wear and tear from environmental factors, thus extending the turbine's operational life. Such technological advances represent substantial engineering breakthroughs, giving engineers versatile new tools to further increase the efficiency and effectiveness of wind energy systems.
Mechanical Design in Wind Energy Systems
Mechanical design is pivotal in optimizing wind energy systems. Engineers focus on creating efficient, robust, and durable designs that can maximize power output while minimizing wear and tear.
- Turbine Blades: These are crafted with aerodynamic principles in mind to optimize lift and reduce drag, following the formula for lift force: \( L = Cl \frac{1}{2} \rho v^2 A \).
- Gearing Systems: Transmit mechanical energy from the rotor to the generator efficiently. Optimal gearing ratios are crucial and are derived through formulas such as \( P = T \omega \), where \( P \) is power, \( T \) is torque, and \( \omega \) is angular velocity.
- Control Systems: Automated systems adjust blade pitch and yaw to capture the maximum wind energy safely and efficiently.
The use of variable speed generators enhances the efficiency of wind turbines by allowing them to adjust to wind speed changes, ensuring constant energy output and reducing mechanical stresses.
An intriguing aspect of mechanical design is the integration of smart materials into wind turbines. These materials respond to environmental changes, such as wind speed or temperature, by altering their properties. For example, employing piezoelectric materials in blade design can lead to automatic adjustments in blade angle, improving efficiency. By harnessing properties such as flexibility and resilience, these materials reduce maintenance needs and improve turbine adaptability to varying wind conditions, representing a cutting-edge evolution in design engineering for renewable energy systems.
Wind Energy Explained: Theory, Design, and Application
Wind energy is at the forefront of renewable energy solutions, offering vast potential for sustainable electricity generation. Understanding its theory, efficient system design, and application challenges is critical in engineering.
Core Principles of Wind Energy
The core principles of wind energy revolve around the conversion of wind’s kinetic energy into mechanical energy, and ultimately into electrical energy. Wind turbines play a central role, functioning through the aerodynamic principles of lift and drag to rotate turbine blades. This rotation drives the generator, producing electricity.Key Concepts include:
- Kinetic Energy: Can be calculated as \( KE = \frac{1}{2}mv^2 \), where \( m \) is mass and \( v \) is velocity.
- Betz Limit: The theoretical maximum efficiency of a wind turbine is 59.3%.
- Aerodynamic Forces: Lift \( L \) and drag \( D \) are critical for blade function, where lift is characterized by \( L = C_l \frac{1}{2} \rho v^2 A \).
Lift Force: A critical aerodynamic force used in the design of wind turbine blades, defined by the equation \( L = C_l \frac{1}{2} \rho v^2 A \), where \( C_l \) is the lift coefficient, \( \rho \) is air density, \( v \) is velocity, and \( A \) is blade area.
The efficiency of wind turbines can be significantly improved with precise blade angle adjustments.
Assume a site has an average wind speed of 8 m/s, calculate the power available in the wind using \( P = \frac{1}{2} \rho A v^3 \), assuming air density \( \rho \) of 1.225 kg/m³ and rotor diameter of 40 meters. Use \( A = \pi (\frac{d}{2})^2 \) for the swept area. Such calculations help engineers estimate the potential energy output of proposed wind farm sites.
Designing Efficient Wind Energy Systems
Creating efficient wind energy systems requires meticulous attention to design details, from the micro aspects of blade design to the macro perspectives of site selection.
- Blade Design: Turbine blades must be aerodynamically optimized to maximize lift and minimize drag, as seen in lift force equations.
- Energy Storage: Balancing supply-demand challenges through battery storage or other techs, like pumped hydroelectric storage.
- Site Selection: Locations are selected based on wind maps indicating sustained high speeds, considering topographical influences on wind patterns.
Applications like Computational Fluid Dynamics (CFD) have revolutionized turbine design by simulating wind interaction with turbines under various conditions. CFD uses the Navier-Stokes equations to predict complex fluid dynamics, and its advanced modeling capabilities allow engineers to optimize blade shapes and configurations for maximal efficiency.
Application Challenges in Wind Energy Engineering
Despite the promising potential of wind energy, there are several application challenges facing engineers.
- Intermittency: Wind is inherently variable, necessitating reliable energy storage solutions to ensure consistent power supply.
- Environmental Impact: The construction and operation can affect wildlife and local ecosystems.
- Logistical Issues: Transporting large turbine components and assembling them in remote or offshore locations is a major engineering challenge.
Advanced research into vertical axis wind turbines (VAWTs) presents solutions to some logistical and environmental challenges. VAWTs are less sensitive to wind direction changes, require less space, and can be installed in urban settings. Additionally, their simpler mechanical structure often leads to lower manufacturing and maintenance costs, offering a potential alternative to traditional horizontal axis designs.
wind energy applications - Key takeaways
- Wind Energy Applications: Key approaches for utilizing wind energy in engineering include power generation, offshore farms, and small turbine systems for remote areas.
- Working Mechanisms: Wind energy systems convert wind's kinetic energy into mechanical, then electrical energy, using components like turbines, generators, and transformers.
- Technical Techniques: Essential methods involve optimizing turbine blade design, site selection through wind maps, and using control systems for efficiency.
- Core Principles: Understanding involves kinetic energy calculations, the Betz limit, and aerodynamic forces like lift and drag in turbine design.
- Theory and Design: Design incorporates physics principles such as fluid dynamics, energy storage solutions, and site selection for efficiency.
- Practical Exercises: Include creating wind maps, CAD blade design, wind flow simulations, and constructing small turbine models for educational purposes.
Learn faster with the 24 flashcards about wind energy applications
Sign up for free to gain access to all our flashcards.
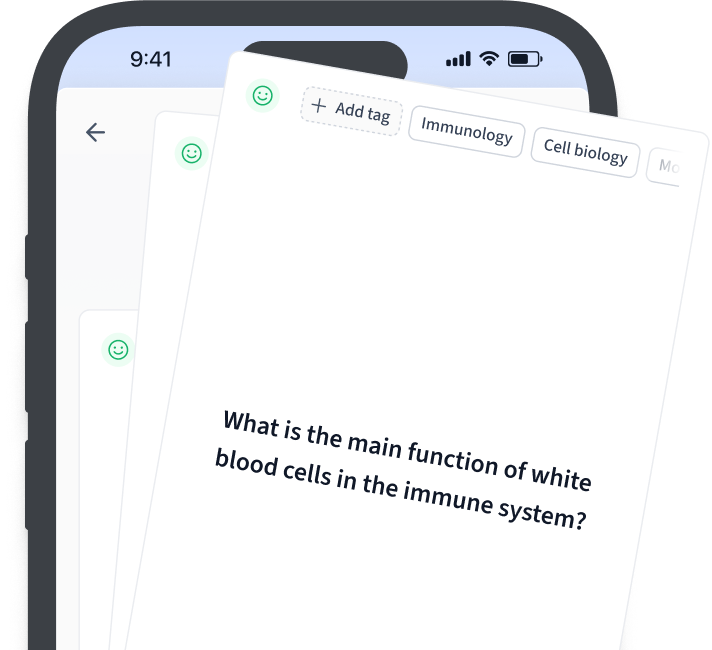
Frequently Asked Questions about wind energy applications
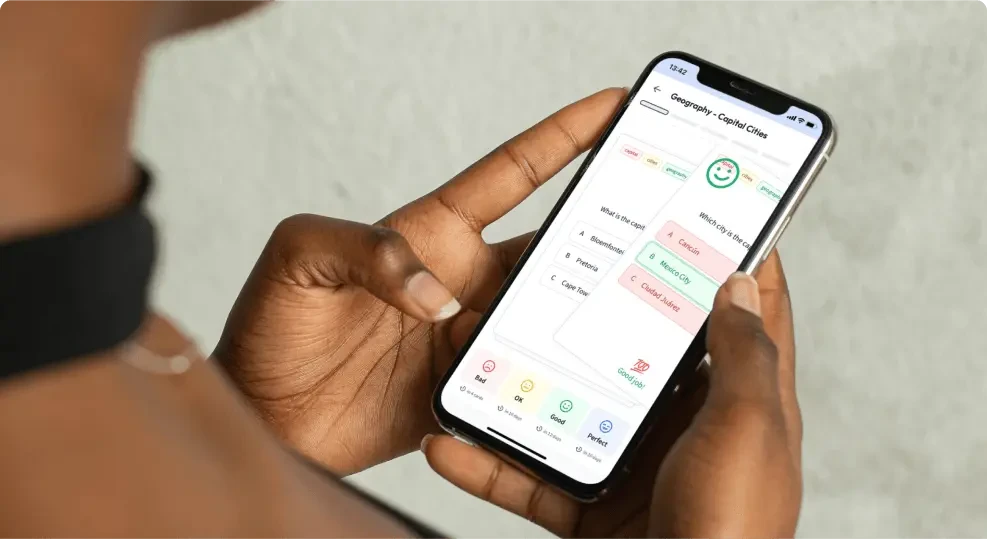
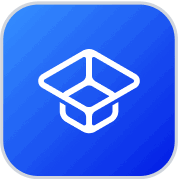
About StudySmarter
StudySmarter is a globally recognized educational technology company, offering a holistic learning platform designed for students of all ages and educational levels. Our platform provides learning support for a wide range of subjects, including STEM, Social Sciences, and Languages and also helps students to successfully master various tests and exams worldwide, such as GCSE, A Level, SAT, ACT, Abitur, and more. We offer an extensive library of learning materials, including interactive flashcards, comprehensive textbook solutions, and detailed explanations. The cutting-edge technology and tools we provide help students create their own learning materials. StudySmarter’s content is not only expert-verified but also regularly updated to ensure accuracy and relevance.
Learn more