Jump to a key chapter
Biochemical Processes Overview
Understanding biochemical processes is fundamental to comprehending how living organisms function at the molecular level. These processes are responsible for converting food into energy, synthesizing complex molecules, and breaking down wastes.
Importance of Biochemical Processes
Biochemical processes play a critical role in the support of life. Almost every function within a living organism relies on biochemical reactions, including:
- Metabolism: This includes both catabolic and anabolic reactions, which break down nutrients and build complex molecules, respectively.
- Cell Signaling: This involves biochemical pathways that transmit signals from one part of the cell to another or to other cells.
- Gene Expression: Biochemical processes determine how genes are turned on or off in response to different environmental cues.
Key Components of Biochemical Reactions
Biochemical reactions generally involve a substrate and an enzyme, which acts as a catalyst to increase the reaction rate. Crucial factors include:
- Substrates: The molecules upon which enzymes act.
- Enzymes: Proteins that significantly speed up chemical reactions.
- Cofactors: Non-protein molecules that assist enzyme function.
Biochemical Process: A series of structural, chemical, and functional changes occurring in living organisms and cells that make life possible.
Consider cellular respiration, a biochemical process where glucose and oxygen yield carbon dioxide, water, and energy in the form of ATP. The simplified chemical equation is: \[C_6H_{12}O_6 + 6O_2 \rightarrow 6CO_2 + 6H_2O + Energy (ATP)\]
Enzymes are not consumed in the biochemical reactions they catalyze; they remain unchanged after the reaction.
Biochemical processes are not only intricately complex but also incredibly precise. Consider the concept of feedback inhibition, where the end product of a reaction inhibits the enzyme's activity earlier in the pathway. This mechanism ensures that cells do not produce more of a substance than is necessary. For example, in the synthesis of amino acids or nucleotides, feedback inhibition prevents the overaccumulation of end products by regulating enzymes through the binding of these end products.
Biochemical Process Engineering Concepts
Biochemical process engineering involves the integration of biological and chemical processes to develop efficient technologies for producing goods such as pharmaceuticals, biofuels, and food. This field utilizes knowledge of enzymes, cell cultures, and bioreactors to facilitate these processes on an industrial scale.
Key Biochemical Engineering Principles
Several fundamental principles are crucial in biochemical engineering:
- Enzyme Kinetics: Understanding the rates at which enzymes catalyze reactions and how they are affected by factors such as temperature and pH.
- Mass and Energy Balances: Applying laws of conservation to predict the inputs and outputs of biochemical reactors.
- Bioreactor Design: Designing vessels that provide suitable conditions for microbial and enzyme activity, including temperature control, aeration, and mixing.
- Downstream Processing: Techniques for product recovery and purification, including filtration, centrifugation, and chromatography.
In an industrial setting, the production of insulin often involves genetically engineered bacteria. First, the bacteria are grown in bioreactors where they express the insulin gene. After fermentation, the insulin is harvested through a series of purification steps. This example illustrates the integration of genetic engineering, bioreactor design, and downstream processing.
Bioprocess engineering is not limited to natural organisms. New fields like synthetic biology are using engineered organisms to create new materials and chemicals.
The principle of scale-up is a key challenge in biochemical engineering. Although a process can be optimized at the laboratory scale, scaling it up to industrial quantities introduces complexities such as maintaining uniform conditions across a larger volume. Parameters like nutrient distribution, oxygen transfer, and heat removal can vary significantly with scale. Engineers often use a step-wise approach, moving from lab to pilot, and then to full-scale to iteratively optimize processes. Advanced computational models and simulations help predict challenges before physical trials.
Applications in Biochemical Process Engineering
Biochemical process engineering has widespread applications across multiple industries:
- Pharmaceuticals: Production of antibiotics, vaccines, and therapeutic proteins, often involving fermentation and cell culture technologies.
- Biofuels: Conversion of biomass into ethanol and biodiesel through enzymatic hydrolysis and microbial fermentation.
- Food and Beverages: Improving processes such as fermentation for products like beer, cheese, and yoghurt.
- Environmental Engineering: Waste treatment using microbial degradation to reduce pollution and recycle resources.
Engineered microbes are also being explored for bioremediation, where they help clean up oil spills and heavy metal contamination by naturally degrading toxic substances.
Biochemical Process of Fermentation
Fermentation is a metabolic process that converts sugar to acids, gases, or alcohol. It involves the action of microorganisms such as yeasts and bacteria. In biochemical terms, fermentation is a method for energy production when cellular respiration cannot proceed efficiently due to lack of oxygen.
Steps in the Biochemical Process of Fermentation
The process of fermentation can be divided into several key steps:
- Glycolysis: This is the first step where glucose is broken down into pyruvate, producing a small amount of ATP and NADH. The general equation for glycolysis is: \[ C_6H_{12}O_6 \rightarrow 2 C_3H_4O_3 + 2ATP + 2NADH \]
- Conversion of Pyruvate: Pyruvate is converted into different products via various pathways depending on the organism and conditions. For alcoholic fermentation, pyruvate is converted into ethanol and carbon dioxide. The equation is: \[ 2C_3H_4O_3 \rightarrow 2C_2H_5OH + 2CO_2 \]
- Regeneration of NAD+: The NADH generated during glycolysis is oxidized back to NAD+. This allows glycolysis to continue in the absence of oxygen.
Lactic acid fermentation, performed by muscle cells, results in lactic acid as a byproduct, causing the sensation of muscle soreness.
Fermentation can occur in different environments and organisms, leading to various end products. Notable forms include:
- Alcoholic fermentation, where yeast produces ethanol, used in brewing and winemaking.
- Lactic acid fermentation, important in dairy products and occurring in human muscle cells.
- Acetic acid fermentation, performed by bacteria to create vinegar.
Industrial Applications of Fermentation
Fermentation technologies have a wide array of industrial applications:
- Food and Beverage Industry: Fermentation is integral to the creation of products like beer, wine, yogurt, cheese, and bread. These processes depend on the activity of microbes that convert sugars into alcohol or acids.
- Pharmaceuticals: The production of antibiotics such as penicillin is carried out through fermentation. Microorganisms are engineered to produce high yields of specific drugs.
- Biofuels: Fermentation is used to produce bioethanol from biomass. This renewable energy source is gaining importance due to its low environmental impact.
An example application of fermentation is in bread-making. Yeast ferments the sugars present in the dough, producing carbon dioxide and ethanol. The carbon dioxide causes the dough to rise, creating a light and airy structure, while the ethanol vaporizes during baking.
The use of engineered microorganisms allows for precise control over fermentation processes, optimizing product yield and efficiency.
Modern fermentation techniques are advancing rapidly due to genetic engineering and systems biology. By manipulating microbial pathways, scientists can enhance yields, reduce unwanted by-products, and innovate new fermentation-based products. For instance, 'precision fermentation' is a technique that not only aims to produce food-grade products but also focuses on pharmaceuticals and specialty chemicals. This involves programming microorganisms to produce specific complex molecules that are otherwise difficult to synthesize chemically. Such advancements promise a future where fermentation helps create sustainable solutions to global challenges.
Biochemical Conversion Process Techniques
In the field of biochemical engineering, conversion processes play a vital role in transforming raw materials into valuable products through biochemical reactions. These processes harness the action of microbes and enzymes to drive chemical transformations, utilized extensively in industries such as pharmaceuticals and biofuels.
Energy and Material Conversion in Biochemical Processes
Understanding how energy and materials are converted in biochemical processes is crucial for developing efficient industrial applications. These processes often involve the transformation of substrates into products through enzymatic reactions. Here, enzymes act as catalysts to facilitate the conversion by lowering the activation energy required for a reaction.Some key aspects of energy and material conversion include:
- Substrate Utilization: The initial materials, such as sugars or starches, are consumed by microorganisms or enzymes.
- Energy Transfer: Biochemical reactions often release or store energy in the form of ATP, a primary energy carrier in cells. The equation for the conversion of ATP is: \[ ATP \rightarrow ADP + P_i + \text{Energy} \]
- Product Formation: Final products can include alcohols, acids, gases, or other chemical compounds.
Biochemical Conversion: The process by which living organisms or enzymes transform substrates into different products, usually involving energy transfer or transformation.
Consider the conversion of glucose into ethanol through fermentation, which can be summarized by the chemical equation: \[ C_6H_{12}O_6 \rightarrow 2C_2H_5OH + 2CO_2 \] This reaction not only converts material but also releases energy used by the microorganisms for growth.
In many biochemical processes, the efficiency of conversion and energy transfer can be enhanced by optimizing specific parameters such as temperature, pH, and substrate concentration. For instance, in biochemical reactors, maintaining optimal conditions for enzyme activity is crucial. A typical enzymatic reaction follows Michaelis-Menten kinetics, described by the equation: \[ v = \frac{{V_{\text{max}} [S]}}{{K_m + [S]}} \] where \( v \) is the rate of reaction, \( V_{\text{max}} \) is the maximum rate, \( [S] \) is the substrate concentration, and \( K_m \) is the Michaelis constant. Understanding these dynamics helps in designing more efficient processes for industrial applications.
Biochemical Processes in Biotechnology
Biochemical processes are integral to the field of biotechnology, which seeks to utilize biological systems or derived biomolecules for innovative solutions and sustainable technology. Biotechnology encompasses the use of cells, biological molecules, and cellular processes to develop new products and technologies.Some notable biotechnological applications include:
- Genetically Engineered Microorganisms: Used in the production of pharmaceuticals and vaccines.
- Bioreactors: Designed to cultivate organisms under controlled conditions for maximal yield of required products.
- Bioinformatics: The computational study of biological data to understand biochemical pathways and optimize them for industrial use.
In agricultural biotechnology, genetically modified organisms (GMOs) are developed to enhance traits such as growth rate or disease resistance. For example, Bt corn is engineered to express a bacterial toxin that deters insect pests, reducing the need for chemical pesticides.
Biotechnological processes often aim to achieve greater sustainability by using renewable resources and reducing waste production through efficient biochemical pathways.
One of the most intriguing advancements in biotechnology is synthetic biology. This involves designing and constructing new biological parts, devices, and systems, or re-designing existing natural biological systems. Synthetic biology allows for the customization of microorganisms to perform tasks like producing biodegradable plastics or even pharmaceuticals. Such reengineering relies heavily on understanding and manipulating metabolic pathways.By integrating concepts from biochemical engineering, computer science, and genetic engineering, synthetic biology holds the potential for transformative impacts across industries.
Biochemical Process of Photosynthesis
Photosynthesis is a vital biochemical process that transforms solar energy into chemical energy, fueling the life cycle on Earth. This process primarily occurs in plants, algae, and some bacteria, converting carbon dioxide and water into glucose and oxygen.
Photosynthesis as a Biochemical Conversion Process
Photosynthesis acts as a biochemical conversion mechanism by which light energy is harnessed to create chemical energy in the form of glucose. This is achieved in two stages:
- Light-dependent Reactions: Occurring in the thylakoid membranes, these reactions use light energy to produce ATP and NADPH. Water molecules are split, releasing oxygen as a byproduct.
- Calvin Cycle (Light-independent Reactions): This stage occurs in the stroma, where ATP and NADPH generated in the light-dependent reactions are used alongside carbon dioxide to synthesize glucose.
Photosynthesis: The process by which green plants and certain other organisms transform light energy into chemical energy (glucose) using sunlight, carbon dioxide, and water, producing oxygen as a byproduct.
The equation representing photosynthesis is:
6CO2 + 6H2O + light energy | → | C6H12O6 + 6O2 |
Chlorophyll, the green pigment in plants, plays a crucial role by absorbing light, which is necessary for the photosynthesis process.
The efficiency of photosynthesis can vary based on environmental conditions such as light intensity, carbon dioxide concentration, and temperature. Plants have developed mechanisms to maximize energy capture and storage. For example, C4 plants like corn and CAM plants like cacti have specialized pathways that enable them to minimize photorespiration, a competing process that can reduce photosynthesis efficiency. C4 plants create a four-carbon compound, hence the name, which helps concentrate carbon dioxide in the chloroplasts, minimizing losses. CAM plants open their stomata at night to reduce water loss and store carbon dioxide as a waxy acid, enabling continued photosynthesis during the day.
Role of Photosynthesis in Biochemical Processes
Photosynthesis is foundational to ecological and biochemical processes, contributing significantly to the oxygen and carbon cycles on Earth.
- Oxygen Production: It provides the majority of the oxygen needed by aerobic organisms for respiration.
- Carbon Fixation: Through photosynthesis, carbon dioxide is fixed from the atmosphere into organic compounds, forming the base of the food chain.
- Energy Source: The glucose produced serves as a primary energy source for plants and, indirectly, for animals and humans through the consumption of plant materials.
Without photosynthesis, the Earth's atmosphere would not contain oxygen, and the lush diversity of life we know today would not exist.
Photosynthesis in marine environments, conducted by phytoplankton, is equally crucial as terrestrial photosynthesis. These microscopic organisms contribute nearly 50% of the global photosynthesis and play a pivotal role in the oceanic carbon cycle. The process undertaken by phytoplankton not only aids in removing large amounts of atmospheric carbon dioxide but also supports marine food webs. Advanced research in photosynthesis aims to enhance crop yields through bioengineering, improving the efficiency of carbon fixation and resilience to abiotic stresses such as drought and heat. This endeavor could significantly boost agricultural productivity and help address the pressing global food demand of a growing population.
biochemical processes - Key takeaways
- Biochemical Processes: Series of structural, chemical, and functional changes that occur in living organisms, crucial for converting food into energy, synthesizing molecules, and breaking down wastes.
- Biochemical Process Engineering: The integration of biological and chemical processes to develop technologies for producing goods, utilizing knowledge of enzymes, cell cultures, and bioreactors.
- Biochemical Process of Fermentation: Metabolic process converting sugars to acids, gases, or alcohols involving microorganisms like yeast and bacteria, crucial for energy production in the absence of oxygen.
- Biochemical Conversion Process: Transformation of substrates into different products through enzymatic reactions, applied in industries like pharmaceuticals and biofuels.
- Biochemical Process of Photosynthesis: Transforms solar energy into chemical energy as glucose, crucial for oxygen production, carbon fixation, and the ecological basis of food chains.
- Biochemical Processes in Biotechnology: Utilizes biological systems for innovative solutions, including applications like genetically engineered microorganisms and bioinformatics to optimize pathways for industrial use.
Learn faster with the 10 flashcards about biochemical processes
Sign up for free to gain access to all our flashcards.
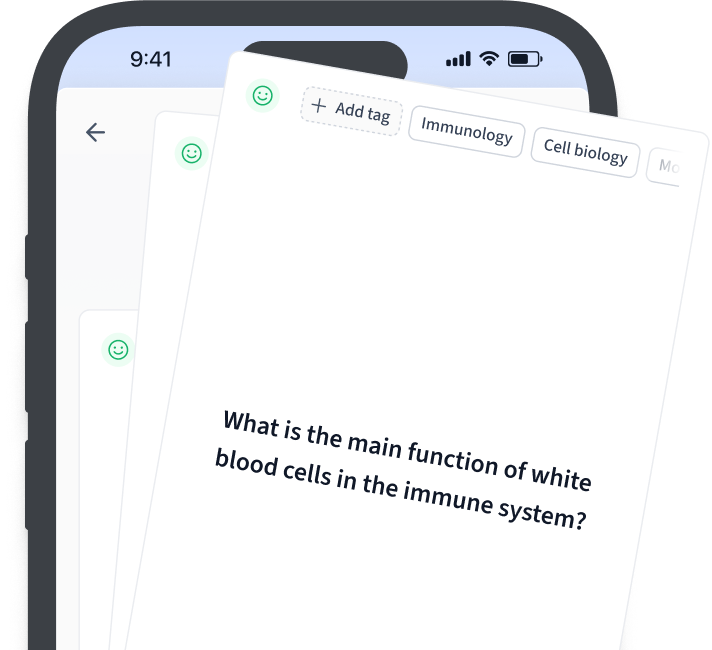
Frequently Asked Questions about biochemical processes
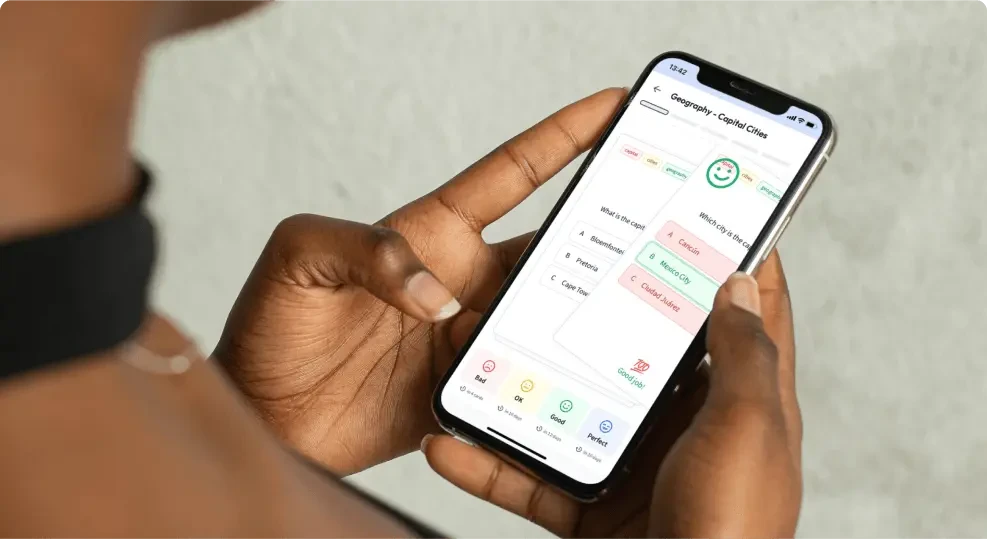
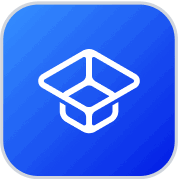
About StudySmarter
StudySmarter is a globally recognized educational technology company, offering a holistic learning platform designed for students of all ages and educational levels. Our platform provides learning support for a wide range of subjects, including STEM, Social Sciences, and Languages and also helps students to successfully master various tests and exams worldwide, such as GCSE, A Level, SAT, ACT, Abitur, and more. We offer an extensive library of learning materials, including interactive flashcards, comprehensive textbook solutions, and detailed explanations. The cutting-edge technology and tools we provide help students create their own learning materials. StudySmarter’s content is not only expert-verified but also regularly updated to ensure accuracy and relevance.
Learn more