Jump to a key chapter
Block Copolymers Overview
Block copolymers are an intriguing type of polymer that are vital in many engineering applications because of their versatile properties. Understanding these materials can offer insights into their usage in various fields such as medicine, electronics, and materials science.
Block Copolymer Definition
Block copolymers are macromolecules composed of two or more chemically distinct polymer blocks, which are covalently bonded. These polymers can be represented as an arrangement of blocks, where each block is a polymer chain segment. The structuring of the blocks provides a unique combination of properties depending on the interactions between the differing segments.To better understand, let’s consider:
- Structure: The arrangement of these blocks often results in a microphase separation due to the incompatibility between different blocks. This can lead to ordered structures such as spheres, cylinders, or lamellae.
- Composition: Based on the types of monomers used, block copolymers can present a wide range of characteristics like toughness, elasticity, or chemical resistance.
Block copolymers are defined as polymers made up from multiple, distinct polymer blocks connected by covalent bonds, exhibiting unique properties due to microphase separation.
Deeper Insight: The formation of distinct phases within block copolymers is primarily a result of thermodynamic forces. These forces drive the distinct blocks to self-assemble into nanoscale domains. Consequently, block copolymers have the unparalleled ability to create materials with properties that are otherwise difficult to achieve with single-phase polymers. This self-assembly process can be mathematically described by the Flory-Huggins theory, which explains the interactions between different types of polymer segments.
Block Copolymer Example
Block copolymers have practical applications in daily life and various industries. One illustrative example is the copolymer made from styrene and butadiene, also known as SBS (styrene-butadiene-styrene). This copolymer finds use in items like the soles of shoes, automotive parts, and as an additive in bitumen to increase the durability of asphalt.Consider the role of SBS in asphalt:
- - Durability: It enhances the elasticity and strength of asphalt, improving resistance to cracking and wear.
- - Performance: Roads made with SBS-modified asphalt can better withstand temperature variations and heavy traffic loads.
A common example of block copolymers is the use of polyethylene oxide and polypropylene oxide in the production of Pluronics, a series of biodegradable surfactants. These materials are utilized in drug delivery systems due to their ability to form micelles, which can encapsulate and transport pharmaceutical compounds effectively in the body.
As you study block copolymers, remember that their unique ability to self-assemble into distinct domains is what imparts them with special properties, making them highly versatile in engineering applications.
Block Copolymer Synthesis
Block copolymer synthesis is a fascinating process that allows for the design of materials with tailored properties. These materials play a significant role in fields ranging from nanotechnology to pharmaceuticals. To appreciate this process, let's look at how block copolymers are synthesized and the challenges encountered during their creation.
Techniques of Block Copolymer Synthesis
Several techniques are employed in the synthesis of block copolymers, each offering specific advantages depending on the desired properties and applications:
- Living Anionic Polymerization: This method provides a controlled approach to synthesizing block copolymers by using a 'living' chain end, allowing for precise control over molecular weight and block length.
- Living Cationic Polymerization: Similar to anionic polymerization, cationic polymerization involves using active centers that do not terminate without the deliberate addition of a terminating agent. This method is suitable for synthesizing polymers with specific arrangement and properties.
- Radical Polymerization: A popular method due to its simplicity and versatility. It allows for the creation of block copolymers using a wider range of monomers, although with less control over polydispersity.
- Ring-Opening Metathesis Polymerization (ROMP): This technique is favored for synthesizing block copolymers with cyclic monomers. ROMP provides a high level of control over the polymer architecture.
Deep Dive: An interesting aspect of block copolymer synthesis is the utilization of reversible-deactivation radical polymerization (RDRP) techniques, such as RAFT (Reversible Addition-Fragmentation Chain Transfer) and ATRP (Atom Transfer Radical Polymerization). RDRP offers outstanding control over polymer architecture and polydispersity, enabling the synthesis of complex block structures that are essential in advanced material design.
Challenges in Block Copolymer Synthesis
Synthesis of block copolymers poses several challenges that need to be addressed to effectively harness their full potential:
- Monomer Reactivity: Disparities in reactivity between different monomers may lead to non-uniform block lengths, which can affect the polymer's physical properties.
- Control Over Molecular Weight: Achieving precise control over the molecular weight is crucial, as even minor deviations can result in significant changes in the performance and application of the copolymer.
- Purity: High purity of monomers and catalysts is essential to avoid unwanted side reactions that could affect the final polymer structure.
- Process Scalability: Translating lab-scale synthesis to industrial-scale production while maintaining polymer quality and consistency is a common hurdle.
Understanding the synthesis process thoroughly can enhance the ability to manipulate block copolymer structures, leading to innovative applications in technology and medicine.
Block Copolymer Self Assembly
The self-assembly of block copolymers is a fascinating process that creates ordered structures due to the interaction of different polymer blocks. This concept is fundamental in advanced material science and engineering applications.
Mechanism of Block Copolymer Self Assembly
The self-assembly of block copolymers is driven by thermodynamic interactions, which cause phase separation within the polymer. This separation depends on factors such as block length and compatibility between blocks. The assembly process involves:
- Microphase Separation: As copolymers cool from a melt, incompatible blocks segregate, creating distinct domains.
- Thermodynamics: The Flory-Huggins parameter, \(\boldsymbol{\text{{\textit{χ}}}}\text{{N}}\), quantifies interactions, where \(\text{{\textit{χ}}}\) is the interaction parameter and \(\text{{N}}\) is the degree of polymerization.
Consider a diblock copolymer consisting of polystyrene (PS) and polyisoprene (PI). When \(\frac{N_{\text{PS}}}{N} \) is around 0.5, lamellar structures form. If conditions change to favor PI, cylindrical or spherical structures might arise. Such structural versatility is crucial in nanotechnology applications.
For an even deeper understanding of block copolymer self-assembly, explore how external fields like electric or magnetic fields can influence the orientation and order of polymer domains. These fields can guide microphase separation, making custom-designed materials suitable for applications in electronics where alignment of motifs is crucial.
Applications of Block Copolymer Self Assembly
Block copolymer self-assembly has a wide range of applications due to the variety of structures it can form. These applications include:
Field | Application | Structure Utilized |
Electronics | Photonic crystals | Cylinder structures |
Medicine | Drug delivery systems | Micelles |
Energy | Battery separators | Lamellae |
Understanding the self-assembly of block copolymers can lead to innovations in creating customizable materials for a variety of high-tech applications.
Block Copolymer Properties
Block copolymers exhibit a range of distinct properties due to the combination of different polymers within their structure. These unique properties find applications in diverse fields, from industry to research.
Physical and Chemical Properties of Block Copolymers
The physical and chemical properties of block copolymers stem from the inherent characteristics of the different polymer blocks and their synergistic combination. Key properties include:
- Thermal Behavior: Block copolymers often display different glass transition temperatures (\( T_g \)) and melting temperatures (\( T_m \)) for each block, offering a broad range of thermal properties.
- Mechanical Strength: The arrangement of blocks, such as hard and soft segments, allows for excellent mechanical properties, enhancing elasticity, toughness, and impact resistance.
- Chemical Resistance: Depending on the blocks used, these copolymers can exhibit superior resistance to solvents, acids, or bases.Mathematically, these properties can be modulated by adjusting the volume fraction \( f \) of each block, impacting the copolymer’s morphology and characteristics. The equation used to calculate the volume fraction is: \[ f = \frac{V_{\text{block}}}{V_{\text{total}}} \]
A classic example illustrating the importance of these properties is the use of poly(ethylene oxide)-b-polypropylene (PEO-b-PPO) copolymers in biomedical applications. The hydrophilic PEO segment enhances biocompatibility, while the hydrophobic PPO segment contributes to stability and strength, making them ideal for drug delivery systems.
Block Copolymer Phase Diagram
Phase diagrams are essential tools in understanding the behavior of block copolymers as they map out the morphological transitions resulting from changes in parameters like temperature, composition, and pressure. The key features include:
- Order-Disorder Transition (ODT): A critical transition where the copolymer changes from a disordered melt to an ordered phase.
- Morphological Regions: The phase diagram outlines different structural arrangements (e.g., spherical, cylindrical, lamellar) depending on the volume fractions \( f_A \) and \( f_B \) of the respective blocks.
- Influence of Flory-Huggins Parameter \( \chi \): This parameter represents the interaction strength between different polymer segments and is key in predicting phase behavior.The critical formula used in conjunction with the phase diagrams is: \[\chi N \approx 10.5 - 12\]
In any phase diagram of block copolymers, note the drastic change in morphology as the \( f \) values approach 0.5, which is indicative of a lamellar structure.
Significance of Block Copolymer Micelles
The formation of micelles is one of the most significant applications of block copolymers, especially in the biomedical field. Micelles are structures formed when the copolymers self-assemble in selective solvents, with one block forming the core and the other the corona.They display unique properties, which include:
- Core-Shell Structure: This allows the encapsulation of hydrophobic drugs, enhancing solubility in aqueous environments.
- Stimuli-Responsive Behavior: Micelles can be designed to respond to external stimuli such as pH, temperature, or light, releasing their payload when needed.
- Targeted Delivery: Functionalizable corona provides options for attaching targeting ligands, enhancing delivery to specific sites.The partition coefficient \(K\) for encapsulation can be mathematically described as: \[K = \frac{C_{\text{core}}}{C_{\text{water}}} \]
In a deeper exploration of block copolymer micelles, consider their role in gene therapy. Recent advances have developed micelle systems capable of delivering genetic material into cells. These systems can protect against degradation and improve delivery efficiency, holding promise for advancing gene therapy techniques.
block copolymers - Key takeaways
- Block Copolymers: Macromolecules composed of chemically distinct polymer blocks covalently bonded, showcasing unique properties due to microphase separation.
- Block Copolymer Synthesis: Techniques like living anionic polymerization, cationic polymerization, and ROMP allow precise control over block copolymer characteristics.
- Block Copolymer Self Assembly: Driven by thermodynamic forces, leading to nanoscale domains that form structures such as spheres, cylinders, or lamellae.
- Block Copolymer Example: SBS (styrene-butadiene-styrene) used in shoes and asphalt, and Pluronics for drug delivery micelles.
- Block Copolymer Properties: Include thermal behavior, mechanical strength, and chemical resistance, influenced by the volume fraction of polymer blocks.
- Block Copolymer Phase Diagram: Used to predict morphological transitions based on parameters like temperature and composition, utilizing the Flory-Huggins Parameter.
Learn faster with the 12 flashcards about block copolymers
Sign up for free to gain access to all our flashcards.
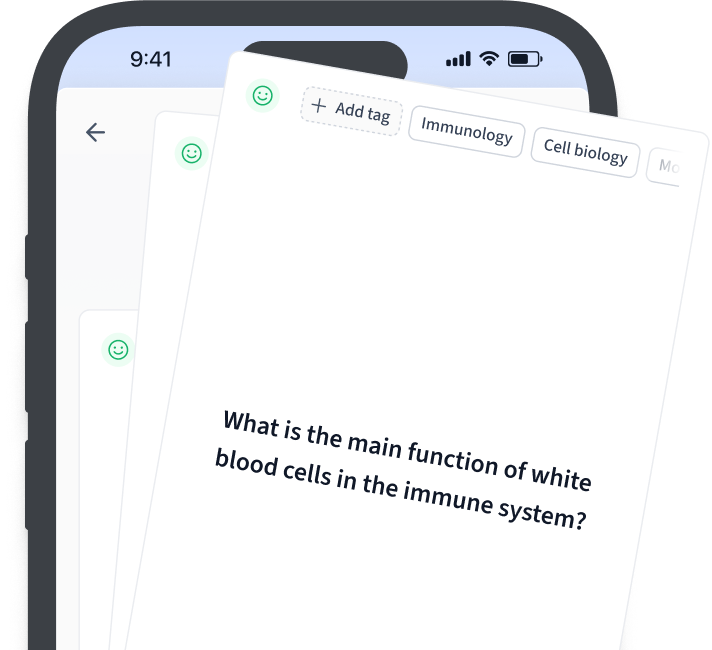
Frequently Asked Questions about block copolymers
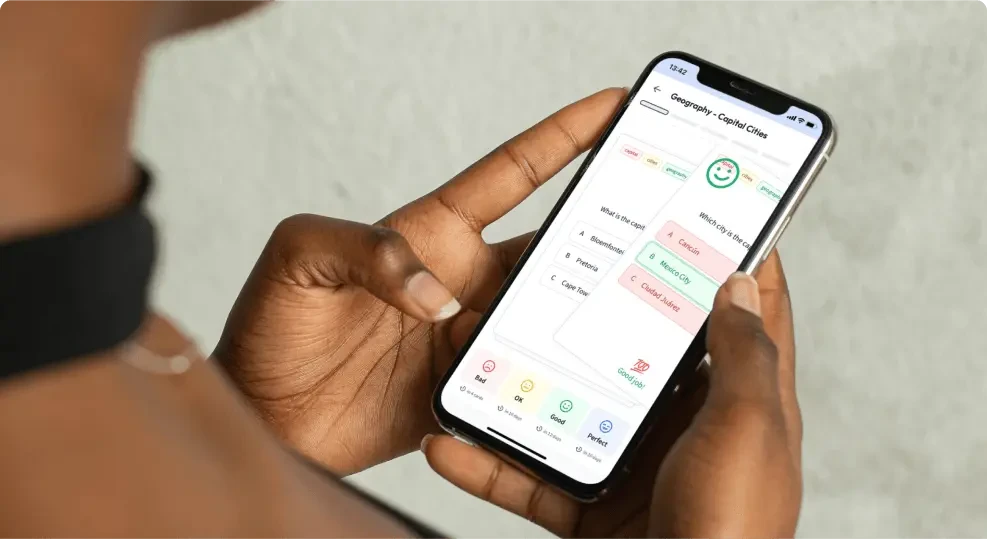
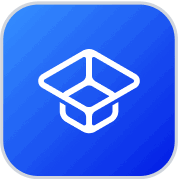
About StudySmarter
StudySmarter is a globally recognized educational technology company, offering a holistic learning platform designed for students of all ages and educational levels. Our platform provides learning support for a wide range of subjects, including STEM, Social Sciences, and Languages and also helps students to successfully master various tests and exams worldwide, such as GCSE, A Level, SAT, ACT, Abitur, and more. We offer an extensive library of learning materials, including interactive flashcards, comprehensive textbook solutions, and detailed explanations. The cutting-edge technology and tools we provide help students create their own learning materials. StudySmarter’s content is not only expert-verified but also regularly updated to ensure accuracy and relevance.
Learn more