Jump to a key chapter
What Are Carbon-Based Materials
Carbon-based materials are a class of substances that contain carbon atoms as a fundamental component. These materials are critical in various engineering applications due to their unique properties, such as high strength, conductivity, and versatility.
Definition of Carbon-Based Materials
Carbon-Based Materials are materials primarily composed of carbon atoms. They include a vast range of substances, from simple molecules like hydrocarbons to complex structures like graphene and carbon nanotubes.
Carbon-based materials are distinguished by their structural variety and diversity. These materials appear in several forms, each having distinct characteristics. The following types are commonly noted:
- Graphite: Known for its excellent electrical conductivity and lubricating properties.
- Graphene: A single layer of carbon atoms arranged in a hexagonal lattice, known for its strength and flexibility.
- Carbon Nanotubes: Cylindrical structures with remarkable electrical and mechanical properties.
- Fullerenes: Molecules made entirely of carbon, often in the form of a hollow sphere, ellipsoid, or tube.
An example of utilizing carbon-based materials is in the development of electrodes for lithium-ion batteries. Graphite is often used due to its structural stability and ability to accommodate lithium ions.
Composition and Properties of Carbon-Based Materials
The composition and properties of carbon-based materials largely depend on the bonding and arrangement of carbon atoms. Carbon atoms can form single, double, or triple bonds, leading to various structural formations that define the material's properties. Examples include:
- Sp3 Hybridization: This involves single bonds as seen in diamond, giving rise to a three-dimensional tetrahedral structure known for its hardness.
- Sp2 Hybridization: Characterized by double bonds, as observed in graphite, leading to two-dimensional planar structures with high electrical conductivity.
- Sp Hybridization: Involves triple bonds, seen in a linear arrangement as in acetylene.
Carbon's ability to catenate, or form chains with itself, allows for building complex molecules and materials.
A fascinating deep dive into carbon-based materials is the study of carbon allotropes. Allotropes are different structural forms of the same element, and carbon has several noteworthy ones, including diamond, graphite, and amorphous carbon. Each allotrope can exhibit vastly different properties; for instance, diamond is one of the hardest materials known, whereas graphite is one of the softest.Diamond's hardness is attributed to the sp3 hybridization of its carbon atoms, forming strong three-dimensional covalent bonds. In contrast, graphite's softness arises from its layers of carbon atoms held together by weaker van der Waals forces, allowing the layers to slide over each other.The modern discovery of graphene further expanded the horizons of carbon materials. Graphene, a single layer of graphite, displays extraordinary electrical, mechanical, and thermal properties, making it a topic of intense research and development. Its electrical conductivity is higher than copper, and its strength exceeds that of steel, offering potential applications in electronics, composites, and more.These unique properties make researching and utilizing carbon allotropes a major focus in materials science and engineering, promising innovative solutions for advanced technologies. Understanding the fundamental bonding and hybridization patterns enables scientists and engineers to manipulate these materials in various forms, leveraging their properties for specific applications.
Carbon-Based Anode Materials for Lithium-Ion Batteries
Lithium-ion batteries are critical components in modern technology, powering everything from smartphones to electric vehicles. At the heart of these batteries lies the anode, with carbon-based materials playing a pivotal role due to their unique characteristics. Carbon-based anodes offer several advantages in enhancing the performance and sustainability of lithium-ion batteries.
Advantages of Carbon-Based Anodes in Lithium-Ion Batteries
1. High Energy Density Carbon-based anodes, particularly those made from graphite, allow for high energy density, enabling devices to hold more charge.2. Long Cycle Life These anodes contribute to a long cycle life, meaning the battery can undergo numerous charge and discharge cycles without significant degradation.3. Good Electrical Conductivity The excellent electrical conductivity of carbon materials supports efficient charge transfer, which is crucial for rapid charging and discharging.
- Graphite’s layered structure facilitates the accommodation of lithium ions during charging.
- The reversible intercalation and de-intercalation of lithium ions are crucial for long-lasting battery life.
Intercalation is the process by which lithium ions are inserted into the layers of the graphite structure during charging.
Consider a battery-operated electric vehicle. A carbon-based anode, with its ability to support high energy densities, allows the vehicle to travel longer distances on a single charge, enhancing efficiency and convenience for users.
Challenges in Using Carbon-Based Anodes for Lithium-Ion Batteries
Despite their benefits, carbon-based anodes face several challenges: 1. Limited Capacity While efficient, the capacity of carbon-based anodes is limited compared to potential alternatives like silicon.2. Safety Concerns The formation of a solid-electrolyte interface (SEI) layer can lead to safety risks. The SEI layer stabilizes the anode but can deteriorate over time, leading to short-circuits.
- Proper management of the SEI layer is crucial to battery safety.
- High surface area of some carbon variants can increase reactivity, requiring careful consideration.
To understand the intricacies of carbon-based anodes, it's important to delve into the electrochemical reactions occurring within lithium-ion batteries:During charging, lithium ions move from the cathode to the anode through the electrolyte, embedding themselves within the carbon layers. This can be expressed mathematically by the reaction: \[ \text{LiCoO}_2 + \text{C}_n \rightarrow \text{Li}_{1-x}\text{CoO}_2 + \text{LiC}_n \] This equation shows lithium ions (Li) being transferred from lithium cobalt oxide (LiCoO2) and intercalating into the carbon (Cn).Upon discharge, this reaction reverses, releasing energy that powers the device. These reactions highlight the necessity of efficiency and stability in carbon-based anodes.Innovations like the development of hybrid carbon-silicon anodes are being explored to overcome current limitations. Combining the benefits of carbon (cycle stability) with the higher capacity of silicon promises extended battery life and enhanced performance, opening avenues for future advances in battery technology.
Future innovations in anode materials may focus on blending carbon with other materials to increase energy storage without sacrificing structural integrity.
Carbon-Based Anode Materials for Sodium Ion Batteries
Carbon-based materials play a significant role in the development of sodium-ion batteries, which are emerging as a promising alternative to lithium-ion batteries. These materials are essential due to their high conductivity, environmental abundance, and ease of fabrication, making them ideal candidates for battery anodes.
Potential of Carbon-Based Anodes in Sodium Ion Batteries
Carbon-based anodes have shown great potential in sodium-ion batteries for several reasons:
- They offer high rate capability and energy efficiency.
- Graphite, commonly used in lithium-ion batteries, is not as effective for sodium ions due to the larger size of Na+ ions compared to Li+ ions.
- Alternative carbon materials like hard carbon or carbon nanofibers can accommodate sodium ions effectively.
Intercalation refers to the reversible inclusion of sodium ions into the layers of carbon, a critical process for the functioning of sodium-ion batteries.
In practical applications, carbon-based anodes can be utilized in stationary energy storage systems where the demand for cost-effective and plentiful materials is high, providing a sustainable option compared to lithium-based systems.
Sodium is more abundant and cost-effective than lithium, which can make sodium-ion batteries a more sustainable choice in the long run.
Developments in Carbon-Based Anode Technologies for Sodium Ion Batteries
Recent advancements in carbon-based anode technologies have focused on enhancing the performance of sodium-ion batteries. Several innovations have emerged:
- Nanostructured Carbon: Modifying the surface of carbon anodes at the nanoscale to improve sodium-ion storage capacity.
- Composite Materials: Integrating carbon with other materials like tin or phosphorus to enhance electronic conductivity and structural stability.
- Hard Carbon: Utilizing hard carbon due to its disordered structure that allows better sodium-ion accommodation.
A deep dive into carbon-based anode technologies reveals fascinating aspects of material science innovations. For example, nanostructuring carbon can significantly impact the electrochemical properties of sodium-ion batteries. By manipulating carbon on the nanoscale, engineers can create spaces and channels that enhance the diffusion and storage of sodium ions. This is especially significant considering the larger size of sodium ions compared to lithium ions.Additionally, research into composite materials involves integrating carbon with metals or metal oxides, enhancing overall battery performance. Tin-based composites, for instance, can help balance the expansion and contraction during charge-discharge cycles, thereby improving battery longevity. The chemical reactions for these composites during battery operation involve complex reduction and oxidation processes that can be mathematically represented but extend beyond basic intercalation.Exploring these advanced carbon-based anode technologies not only pushes the boundaries of energy storage capabilities but also contributes to developing more sustainable and efficient energy solutions.
Carbon-Based Materials as Supercapacitor Electrodes
Supercapacitors are increasingly popular in the field of energy storage due to their ability to deliver rapid power. Carbon-based materials are often chosen as electrodes for these devices because of their excellent conductivity, large surface area, and cost-effectiveness.
Benefits of Using Carbon-Based Supercapacitor Electrodes
1. High Power Density Carbon-based materials provide supercapacitors with a high power density, enabling them to release energy quickly.2. Superior Conductivity Their remarkable electrical conductivity ensures efficient charge transfer, vital for high-performance supercapacitors.3. Environmental Stability Carbon-based electrodes are stable across a range of temperatures and resist environmental degradation.
- Activated carbon, commonly used in these applications, offers a high surface area for energy storage.
- Graphene and carbon nanotubes are also used to enhance performance due to their unique properties.
Consider a supercapacitor used in public transportation systems that require rapid acceleration and deceleration. Carbon-based electrodes provide the necessary power density and longevity to withstand repeated use without significant performance loss.
A supercapacitor is a high-capacity capacitor with higher capacitance than traditional capacitors. It combines the storage capabilities of batteries with the high power output of capacitors.
When delving into the mechanics of carbon-based supercapacitor electrodes, the principle of electrical double-layer capacitance (EDLC) comes into play. EDLC involves the electrostatic storage of charge at the interface between the electrolyte and the carbon electrode's surface. This can be expressed by the formula:\[ C = \varepsilon \frac{A}{d} \]Where:
- C is the capacitance
- \varepsilon is the permittivity of the electrolyte
- A is the surface area of the electrode
- d is the thickness of the double layer
Graphene, with its vast surface area and exceptional conductivity, is a key material being explored for next-generation supercapacitor electrodes.
Innovations in Carbon-Based Supercapacitor Electrode Design
Emerging innovations in carbon-based electrode design are breaking new ground in enhancing supercapacitor efficiency. Various methods are being developed to optimize these materials:
- Graphene Oxide Reduction: This technique increases conductivity and surface area by chemically reducing graphene oxide.
- Carbon Nanotube Integration: Embedding carbon nanotubes within the electrode structure results in enhanced electron transport and mechanical strength.
- 3D Architecture Design: Engineering electrodes with three-dimensional architectures increase accessibility to active sites, thereby improving charge storage.
One of the exciting directions in carbon-based supercapacitor design is the development of hybrid materials:A promising approach is integrating transition metal oxides with carbon materials to form a hybrid electrode. These materials aim to combine the high energy density of battery-like redox material and the structural advantages of carbon. The reaction mechanisms in such systems often involve faradaic reactions alongside capacitance, providing a dual mechanism for energy storage.The chemical reactions for these hybrid materials can be represented as:\[ \text{M}^{n+} + ne^- \rightarrow \text{M}^{(n-1)+} \]A transition metal oxide (Mn+) is reduced, storing more energy. These complex processes enhance the material's capacitance and energy density, heralding a new era of more efficient and powerful energy storage solutions.
Techniques for Synthesizing Carbon-Based Materials
The synthesis of carbon-based materials is crucial in engineering and scientific research, given their diverse applications and unique properties. Numerous techniques have been developed for creating these materials, each tailored to achieve specific characteristics or forms.
Common Methods for Carbon-Based Materials Synthesis
Several traditional methods are employed in the synthesis of carbon-based materials. Each approach has its advantages and is selected based on the desired properties of the material.
- Chemical Vapor Deposition (CVD): Involves decomposing gaseous hydrocarbons at high temperatures to form a thin film of carbon on a substrate.
- Sol-Gel Process: A solution containing a carbon precursor is subjected to gelation and drying to produce carbon xerogels or aerogels.
- Pyrolysis: This thermal decomposition of organic material in an inert atmosphere produces carbon materials such as activated carbon.
An example of the common methods is the use of CVD to produce high-quality graphene films, essential for electronic applications due to their excellent electrical properties.
Advanced Techniques in Carbon-Based Materials Synthesis
For tailored applications requiring more precise material characteristics, advanced synthesis techniques are employed. These methods often involve more intricate procedures and state-of-the-art technology.
- Laser Ablation: Utilizes a high-energy laser to vaporize carbon targets and deposit carbon nanostructures.
- Electrochemical Deposition: Applies electrical current to deposit carbon from a solution onto a substrate, achieving uniform coatings.
- Plasma Enhanced Chemical Vapor Deposition (PECVD): A variation of CVD that employs plasma to enhance the reaction kinetics and improve material quality.
Laser Ablation is a technique wherein high-intensity laser light is used to remove material from a solid surface, in this context, used to synthesize carbon nanomaterials.
One of the exciting developments in advanced carbon synthesis is using plasma technology. This technology allows for the precise synthesis of carbon nanostructures by creating high-energy environments that can break down carbon-containing compounds into atomic or molecular forms. Once decomposed, these components can then deposit onto substrates, forming structures like graphene or carbon nanotubes.A deeper look into Plasma Enhanced Chemical Vapor Deposition (PECVD) reveals its capabilities in manipulating material properties. The plasma's energy enhances chemical reactions that deposit carbon films or nanotubes with superior quality and scalability.By controlling parameters like plasma density and substrate temperature, you can fine-tune the materials' electrical and mechanical properties. The outcome is a high-purity product essential for advanced electronics, sensor technologies, and energy applications.
In material synthesis, controlling the environment’s temperature and pressure is crucial in determining the final product's properties.
carbon-based materials - Key takeaways
- Definition of Carbon-Based Materials: Materials primarily composed of carbon atoms, ranging from simple hydrocarbons to complex structures like graphene and carbon nanotubes.
- Carbon-Based Anode Materials for Lithium-Ion Batteries: Graphite is commonly used due to its capacity for high energy density, long cycle life, and good electrical conductivity, despite challenges like limited capacity and safety concerns.
- Carbon-Based Anode Materials for Sodium Ion Batteries: Alternative carbon materials like hard carbon can accommodate the larger Na+ ions effectively, offering promise in the development of sodium-ion batteries.
- Carbon-Based Materials as Supercapacitor Electrodes: Used in supercapacitors for high power density, superior conductivity, and environmental stability.
- Techniques for Synthesizing Carbon-Based Materials: Common methods include Chemical Vapor Deposition (CVD), Sol-Gel Process, and Pyrolysis, with advanced techniques like Laser Ablation and Plasma Enhanced Chemical Vapor Deposition (PECVD).
- Examples of Carbon-Based Materials: Graphite, graphene, carbon nanotubes, and fullerenes, each with distinct properties and applications.
Learn faster with the 10 flashcards about carbon-based materials
Sign up for free to gain access to all our flashcards.
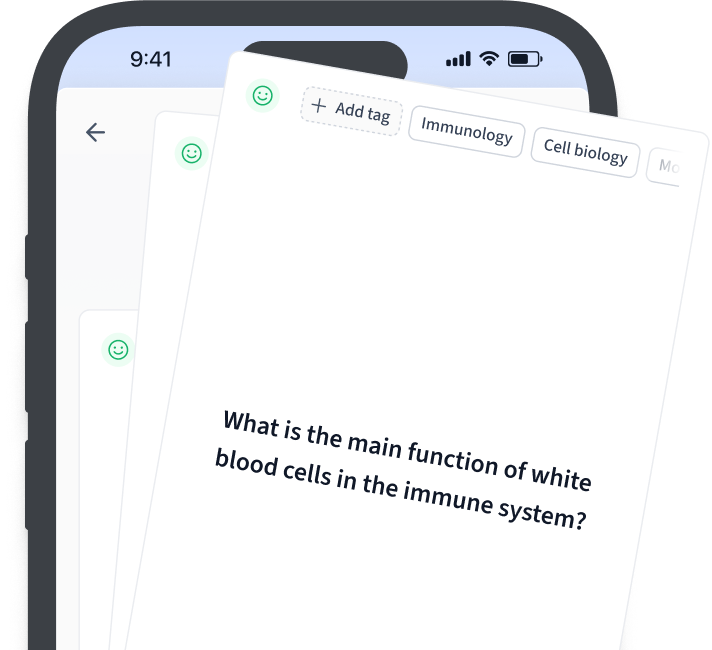
Frequently Asked Questions about carbon-based materials
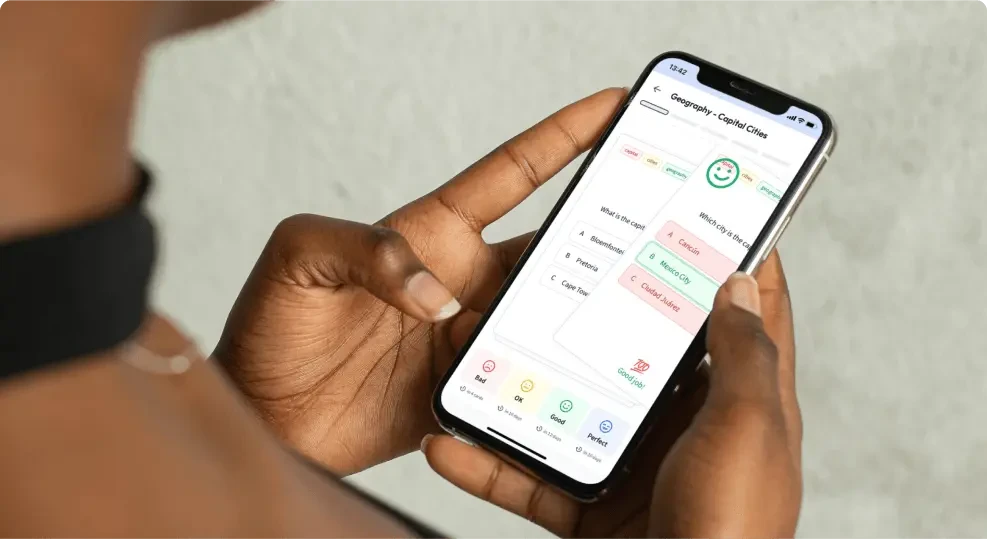
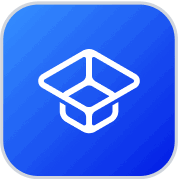
About StudySmarter
StudySmarter is a globally recognized educational technology company, offering a holistic learning platform designed for students of all ages and educational levels. Our platform provides learning support for a wide range of subjects, including STEM, Social Sciences, and Languages and also helps students to successfully master various tests and exams worldwide, such as GCSE, A Level, SAT, ACT, Abitur, and more. We offer an extensive library of learning materials, including interactive flashcards, comprehensive textbook solutions, and detailed explanations. The cutting-edge technology and tools we provide help students create their own learning materials. StudySmarter’s content is not only expert-verified but also regularly updated to ensure accuracy and relevance.
Learn more