Jump to a key chapter
Definition of Catalysis Thermodynamics
Catalysis thermodynamics is a branch of thermodynamics that focuses on understanding the energy changes and equilibrium processes in reactions where a catalyst is present. It plays a crucial role in studying how catalysts alter the reaction rates and influence the direction and extent of chemical reactions.
Catalysis Thermodynamics Explained
Catalysts are substances that accelerate a chemical reaction without being consumed in the process. They work by providing an alternative pathway for the reaction, reducing the activation energy, and consequently increasing the reaction rate. The essential thermodynamic parameters involved in catalysis include enthalpy \(\Delta H\), entropy \(\Delta S\), and the Gibbs free energy \(\Delta G\). These parameters help predict whether a reaction will be spontaneous and how much energy will be exchanged.The Gibbs free energy change \(\Delta G\) in a catalyzed reaction can be calculated using the equation:\[ \Delta G = \Delta H - T\Delta S \]where:
- \(\Delta H\) is the change in enthalpy
- \(\Delta S\) is the change in entropy
- \(T\) is the temperature in Kelvin
Imagine conducting a reaction where methane \((CH_4)\) is produced from carbon monoxide \((CO)\) and hydrogen \((H_2)\). A catalyst such as nickel would facilitate the reaction:\[ CO + 3H_2 \rightarrow CH_4 + H_2O \]This process helps achieve higher conversion rates and efficiency at reduced temperatures compared to an uncatalyzed reaction.
Catalysts can also affect the equilibrium position of a reaction. While they don't alter the equilibrium constant \(K\), they enable the system to reach equilibrium faster. A classic example is the Haber process for synthesizing ammonia \((NH_3)\) from nitrogen \((N_2)\) and hydrogen \((H_2)\). In this industrial process, using an iron catalyst allows equilibrium to be achieved more quickly under high pressure and moderate temperatures, leading to practical production rates. An understanding of catalysis thermodynamics is imperative for optimizing these and other industrial chemical processes, ensuring they are both cost-effective and environmentally friendly.
Thermodynamics of Catalysis
The thermodynamics of catalysis revolves around understanding how catalysts influence the energy changes during a reaction. Key concepts include:
- Activation Energy: Catalysts function by lowering the activation energy \(E_a\), allowing reactions to proceed quicker at given temperatures. This is pivotal in energy savings in industrial processes.
- Reaction Pathway: A catalyst may provide an alternative reaction pathway, leading to a more efficient route than the uncatalyzed reaction.
- Energy Profile Diagrams: These diagrams illustrate the energy changes; a catalyzed reaction profile shows a lower peak of energy representing decreased activation energy.
While catalysts speed up reactions, it's crucial to remember they cannot change the equilibrium point or improve yields; they only make equilibrium attainment faster.
Fundamentals of Catalysis Thermodynamics
Catalysis thermodynamics explores the energy and equilibrium changes in chemical reactions with catalysts. These concepts are vital in improving reaction speeds and influencing chemical process efficiency.
Basic Concepts and Key Ideas
A catalyst alters reaction rates by providing an alternative pathway with lower activation energy, \(E_a\) without being consumed. The primary parameters in catalysis thermodynamics include:
- Enthalpy (\(\Delta H\)): Measures heat exchange in a reaction.
- Entropy (\(\Delta S\)): Indicates disorder or randomness change.
- Gibbs Free Energy (\(\Delta G\)): Predicts spontaneity; can be determined using \(\Delta G = \Delta H - T \Delta S\).
Consider synthesizing ammonia \((NH_3)\) via the Haber process from nitrogen \((N_2)\) and hydrogen \((H_2)\):\[ N_2 + 3H_2 \rightarrow 2NH_3 \]Using an iron catalyst, the reaction's rate increases and achieves equilibrium quicker while maintaining the same \(\Delta G\).
Though catalysts speed reactions, they cannot change reaction equilibrium points or yields; they only accelerate reaching equilibrium.
Catalysts, despite not altering the equilibrium constant \(K\), permit systems to reach equilibrium hastily. In the industrial synthesis of methanol \((CH_3OH)\), catalysts ensure efficiency. A reaction such as:\[ CO + 2H_2 \rightarrow CH_3OH \]demonstrates improved conversion rates when a metal-based catalyst is used. These advancements highlight the importance of catalysis thermodynamics in sustainable and efficient chemical engineering processes.
Thermodynamic Principles in Catalysis
The study of thermodynamic principles in catalysis focuses on how catalysts modify the energy landscape of a reaction. Key elements include:
- Activation Energy: Catalysis lowers \(E_a\), facilitating faster reactions at various temperatures, crucial for energy-efficient industrial processing.
- Reaction Pathway Alteration: By offering alternate pathways with lower energy barriers, catalysts redefine how reactions occur.
- Energy Profile Diagrams: These graphs depict energy transitions; catalyzed reactions show a reduced peak, indicative of lower \(E_a\).
Thermodynamic Framework for Catalysis
Understanding the thermodynamic framework for catalysis is crucial for analyzing how reactions occur in the presence of catalysts. This framework helps you predict reaction behaviors, optimize conditions, and better comprehend how different factors affect chemical processes.
Application in Chemical Reactions
Catalysts are essential for modifying reaction pathways and rates, making reactions faster and more efficient without being consumed in the process.Key thermodynamic concepts in the application of catalysts include:
- Activation Energy (\(E_a\)): This is the minimum energy required to start a reaction. Catalysts lower \(E_a\), allowing for quicker reaction rates.
- Reaction Mechanism: Catalysts can provide an alternative pathway for reactions, often through intermediate species, thus altering the mechanism.
Analyzing the industrial synthesis of ammonia \((NH_3)\) from nitrogen \((N_2)\) and hydrogen \((H_2)\):\[N_2 + 3H_2 \rightarrow 2NH_3\]An iron catalyst reduces the activation energy, enabling the reaction to occur efficiently at a manageable temperature and pressure. This is crucial for large-scale ammonia production used in fertilizers.
Catalysts themselves do not change the intrinsic energies but provide alternative routes with lower energy requirements, enhancing reaction efficiency.
Models and Theories
Several models and theories explain the behavior and effectiveness of catalysts in reactions. Understanding these theories can assist in designing better and more efficient catalytic processes.The most common models include:
- Transition State Theory (TST): It postulates that reactants form an activated complex or transition state, which then transforms into products. Catalysts stabilize this transition state, reducing \(E_a\).
- Langmuir-Hinshelwood Mechanism: Often used in heterogeneous catalysis, this model describes reactions occurring on the surface of solid catalysts. Adsorption, reaction, and desorption are critical steps.
- Eyring Equation: This is used to relate the rate of reaction to the energy of the transition state. The equation is:\[k = \frac{k_B T}{h} e^{-\frac{\Delta G^\ddagger}{RT}}\]where \(k\) is the rate constant, \(k_B\) is the Boltzmann constant, \(T\) is the temperature, \(h\) is Planck's constant, and \(\Delta G^\ddagger\) is the Gibbs free energy of activation.
A remarkable application of catalytic theory is in the design of automotive catalytic converters. These converters use metals like platinum to lower the activation energy, transforming pollutants like carbon monoxide and nitrogen oxides into less harmful emissions such as carbon dioxide and nitrogen. The surface mechanisms and energy profiles align with Langmuir-Hinshelwood principles, showcasing how models guide practical solutions in reducing environmental impact.
Advanced Topics in Catalysis Thermodynamics
Advancements in catalysis thermodynamics are pivotal for improving reaction efficiencies and reducing energy consumption in chemical processes. These explorations delve into complex systems and emerging innovations.
Current Research and Innovations
Recent studies in catalysis thermodynamics focus on innovation to enhance catalyst efficiency and develop new catalytic materials. These advancements are crucial for applications in energy conversion, environmental remediation, and material synthesis.Key areas of research include:
- Nanocatalysts: Utilizing nanoparticles as catalysts to provide greater surface area and reactivity.
- Biocatalysts: Enzymes functioning as catalysts, promising for sustainable and specific reactions.
- Photocatalysis: Using light to enhance reaction rates, particularly in renewable energy applications.
Catalyst Type | Application |
Nanocatalysts | Fuel cells and hydrogen production |
Biocatalysts | Pharmaceuticals and biofuels |
Photocatalysts | Solar energy and environmental cleanup |
In practical terms, nanocatalysts such as platinum nanoparticles are used in fuel cells to efficiently convert chemical energy into electrical energy. Their high surface area facilitates excellent catalytic activity even at low loadings, making them economically attractive.
Catalyst research not only aims to enhance reactivity but also focuses on cost-effectiveness and minimizing environmental impact.
Complex Systems and Challenges
Catalysis thermodynamics involves tackling challenges in complex systems, particularly those involving multiple phases or reaction steps. Such complexities encompass the interactions of various reactants and intermediates within catalytic cycles.Key challenges include:
- Reaction Mechanisms: Unraveling multistep processes and identifying active sites.
- Stability: Ensuring catalyst longevity under varied operational conditions.
- Scalability: Transitioning from lab-scale reactions to industrial scales.
A deeper understanding of complex catalytic systems can be gained through computational modeling and advanced characterization techniques. Computational chemistry tools, such as Density Functional Theory (DFT), allow for the prediction of reaction pathways and the exploration of active sites. These methods enable researchers to design improved and more efficient catalysts. Additionally, spectroscopy methods, such as X-ray diffraction and electron microscopy, help elucidate the structural and compositional changes within catalysts during reaction cycles. As such, the development of robust catalysts capable of operating under harsh conditions continues to be a dynamic research area, merging theoretical insights with experimental validations.
catalysis thermodynamics - Key takeaways
- Catalysis Thermodynamics: Focuses on energy changes and equilibrium in reactions with catalysts, affecting reaction rates and directions.
- Thermodynamic Parameters: Enthalpy (abla H), Entropy (abla S), and Gibbs Free Energy (abla G) help predict the spontaneity and energy exchange in catalyzed reactions.
- Reaction Spontaneity: A reaction is spontaneous when abla G is negative, and catalysts lower the activation energy without changing overall abla G.
- Equilibrium and Kinetics: Catalysts allow reactions to reach equilibrium faster, crucial in processes like the Haber synthesis of ammonia.
- Thermodynamic Principles in Catalysis: Include lowering activation energy and providing alternative reaction pathways for energy efficiency.
- Thermodynamic Framework: Helps in designing efficient catalytic systems, predicting behaviors, and optimizing conditions in chemical processes.
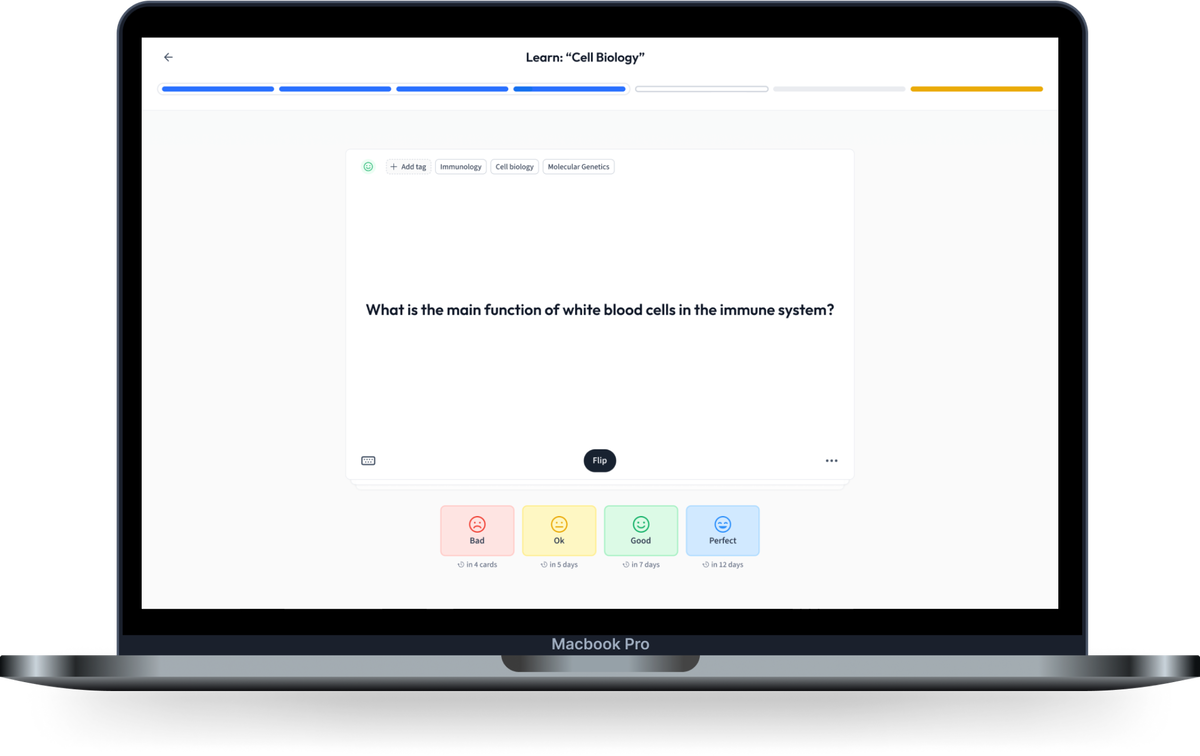
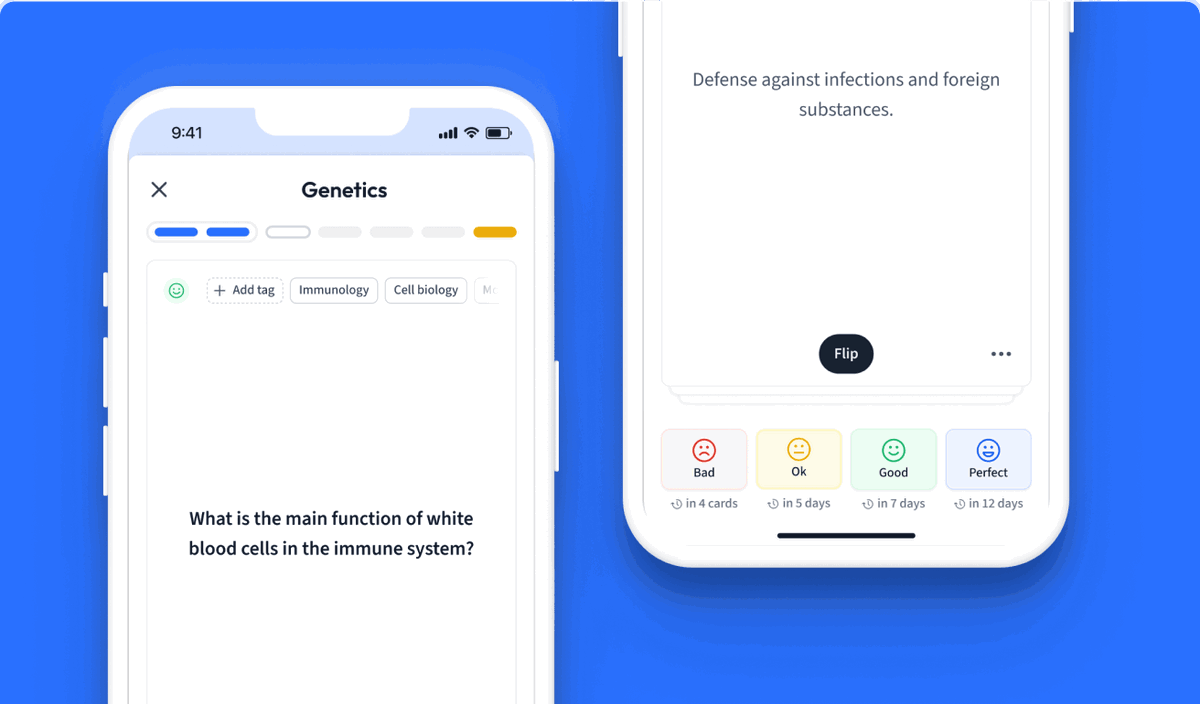
Learn with 12 catalysis thermodynamics flashcards in the free StudySmarter app
Already have an account? Log in
Frequently Asked Questions about catalysis thermodynamics
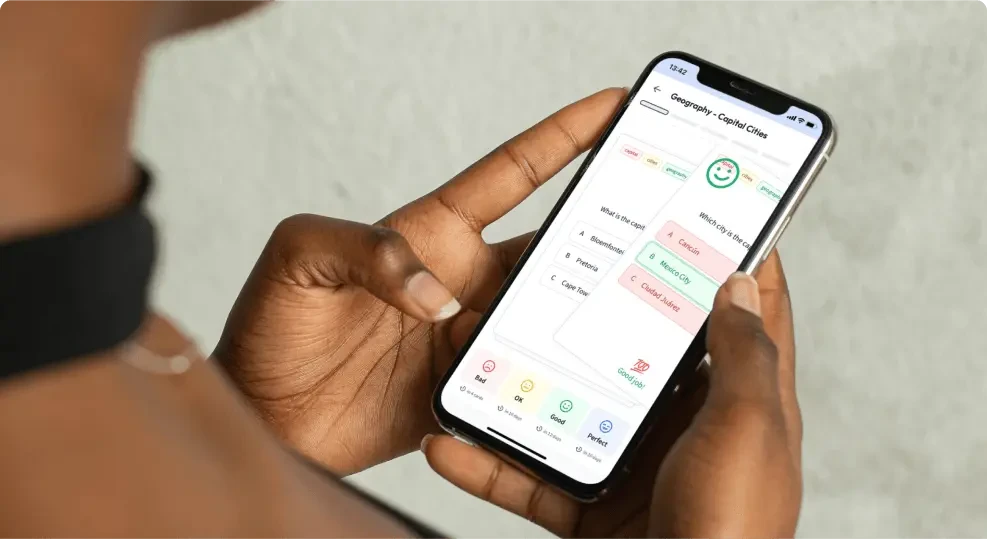
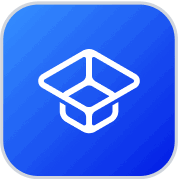
About StudySmarter
StudySmarter is a globally recognized educational technology company, offering a holistic learning platform designed for students of all ages and educational levels. Our platform provides learning support for a wide range of subjects, including STEM, Social Sciences, and Languages and also helps students to successfully master various tests and exams worldwide, such as GCSE, A Level, SAT, ACT, Abitur, and more. We offer an extensive library of learning materials, including interactive flashcards, comprehensive textbook solutions, and detailed explanations. The cutting-edge technology and tools we provide help students create their own learning materials. StudySmarter’s content is not only expert-verified but also regularly updated to ensure accuracy and relevance.
Learn more