Jump to a key chapter
Definition of Catalyst Poisoning
Catalyst poisoning occurs when a catalyst's effectiveness is diminished due to the presence of an undesired substance. This phenomenon is of significant importance in chemical reactions where catalysts are used to speed up the process by reducing the activation energy required.
Causes of Catalyst Poisoning
Catalyst poisoning can occur due to:
- Unintentional impurities in the reaction mixture.
- Engineered processes that do not properly account for potential contaminants.
- Decomposition of other reactants that produce inhibitory by-products.
An adsorption is a surface-based process where a substance accumulates on the surface of a solid or liquid.
Consider a refinery where sulfur compounds are inadvertently introduced into a catalytic converter used for desulfurization. The sulfur can poison the catalyst by adhering to its active sites, rendering the catalyst ineffective until it is regenerated or replaced.
Consequences of Catalyst Poisoning
The main consequence of catalyst poisoning is a reduction in reaction efficiency, which may lead to:
- Increased operational costs due to more frequent replacement of catalysts.
- Lower yield of desired product, impacting overall profitability.
- Increased environmental concerns if processes are not efficiently managed.
Interestingly, certain catalyst poisons can sometimes be used deliberately to control a reaction's rate or selectivity.
Prevention and Management
To prevent or manage catalyst poisoning, industries employ various strategies, such as:
- Careful selection of catalyst materials that are resistant to the specific poisons expected in the system.
- Implementation of pre-treatment methods to remove unwanted impurities.
- Using regenerative techniques to renew spent catalysts.
Regeneration techniques range from simple thermal treatments to complex chemical processes. For example, one common method involves heating the catalyst to high temperatures in the presence of air to burn off accumulated poisons. This process can help restore some of the catalyst's activity but is not always 100% effective. For noble metal catalysts, with examples including platinum and palladium, regeneration can be particularly challenging due to the high costs and energy requirements.
Catalyst Poisoning Explained
Catalyst poisoning is a critical issue in the field of chemical engineering. It involves the reduction in catalyst activity due to the presence of impurities or reactants that bind strongly to the active sites of the catalyst. This can inhibit the intended chemical reactions, rendering the catalyst ineffective.
Types of Catalyst Poisoning
Catalyst poisoning can be categorized based on the nature of the interaction and the type of poison involved.
- Reversible Poisoning: Occurs when a poison temporarily deactivates a catalyst, but the activity can be restored by removing the poison.
- Irreversible Poisoning: The poison permanently deactivates the catalyst. This often necessitates replacing the catalyst.
- Competitive Poisoning: Involves poisons competing with reactants for the active sites on a catalyst, reducing its effectiveness.
- Non-competitive Poisoning: Occurs when poisons do not compete with reactants but still affect the catalyst's performance.
In catalytic converters used in automobiles, leaded fuel can cause irreversible poisoning. Lead compounds attach to the catalyst's active sites, permanently blocking them and reducing emissions control effectiveness.
The mechanisms involving catalyst poisoning are often explored through mathematical equations and models. For instance, the Langmuir-Hinshelwood model is used to describe reaction rates \[Rate = k \frac{K_aC_A}{1 + K_aC_A + K_iC_i}\] where - \(k\) is the rate constant, - \(K_a\) is the adsorption constant of the reactant, - \(C_A\) is the concentration of the reactant, - \(K_i\) is the adsorption constant of the poison, - \(C_i\) is the concentration of the poison.
Understanding catalyst poisoning requires examining both physical and chemical interactions at the atomic level. For example, some poisons form chemical bonds with the catalyst, altering its surface properties. Analytical techniques such as X-ray photoelectron spectroscopy (XPS) and electron microscopy help scientists visualize these changes, leading to improved catalyst design and regeneration techniques.
Catalyst regeneration often employs high-temperature treatments or chemical washes to remove surface contaminants and restore activity, but effectiveness varies based on the poison and catalyst type.
Catalyst Deactivation by Poisoning
Catalyst poisoning is a complex process that impacts the efficiency of catalysts in chemical reactions. It involves unwanted substances binding to catalyst active sites, thus inhibiting their function. Understanding the various aspects of catalyst poisoning is crucial for improving reaction efficiencies and innovating better catalytic processes.
Chemical Interactions in Catalyst Poisoning
The chemical interactions in catalyst poisoning involve specific poisons adhering to a catalyst's surface, affecting its reactivity. Factors influencing these interactions include:
- Nature of the catalyst: Different materials like metals or oxides react distinctively to poisons.
- Type of poison: Substances such as sulfur, lead, or carbon monoxide are common poisons.
- Reaction environment: Temperature, pressure, and reactant concentrations play vital roles.
Catalyst poisoning occurs when foreign substances bind strongly to catalyst sites, reducing its catalytic activity.
A classic example can be observed in ammonia synthesis using iron catalysts. Sulfur compounds bind to the iron active sites, rendering the catalyst ineffective until it is treated or replaced.
In-depth studies using spectroscopy have revealed how poisons interact at the atomic level. For instance, sulfur forms stable bonds with metal atoms on the catalyst surface, creating a passivation layer that prevents reactants from accessing these active sites. Such insights lead to the development of more resilient catalysts, able to maintain activity despite potential poisoning scenarios.
Some catalysts are designed with specific resistance to known poisons, providing longer operational lifetimes in industrial settings.
Impact on Reaction Efficiency
Catalyst poisoning can significantly reduce the efficiency of chemical processes, leading to increased operational costs and environmental hazards. For example, consider the reaction: \[ A + B \rightarrow C \] The introduction of a poison can decrease the reaction rate or shift the equilibrium: \[Rate = k \frac{C_A}{1 + C_iK_i}\] Where: - \(C_A\) is the concentration of reactant A - \(K_i\) is the adsorption constant of the poison - \(C_i\) is the poison concentration
Mitigating catalyst poisoning can dramatically enhance process efficiency and sustainability.
Palladium Catalyst Poisoning
Palladium catalysts are widely used in industrial processes due to their ability to facilitate chemical reactions efficiently. However, these catalysts are not immune to poisoning, which can severely impact their performance and the overall efficiency of chemical processes.
Causes of Palladium Catalyst Poisoning
There are several causes of palladium catalyst poisoning:
- Presence of Halides: Chloride and bromide ions can strongly adhere to the active sites of palladium, hindering its catalytic capability.
- Sulfur Compounds: Sulfur is known for irreversibly poisoning palladium by forming strong bonds with the metal.
- Hydrocarbons: Certain hydrocarbons, through adsorption, can block active sites and alter surface properties.
- Oxygen Exposure: Palladium can oxidize, forming a less active oxide layer.
An example of palladium catalyst poisoning occurs in hydrogenation reactions. When sulfur-containing gases are present, they strongly bind to palladium, decreasing its activity until these gases are removed or the catalyst is regenerated.
Shielding palladium catalysts from potential poisons can be achieved by pretreating feedstocks to remove impurities.
Solutions for Palladium Catalyst Poisoning
Addressing and preventing palladium catalyst poisoning involves multiple strategies:
- Feedstock Purification: Removing impurities such as sulfur and halides from reactant streams can mitigate poisoning risks.
- Use of Promoters: Adding small amounts of another element can enhance the resistance of palladium to poisons.
- Regeneration Techniques: Employ thermal or chemical methods to remove adsorbed poisons and restore catalyst activity.
- Development of Alloy Catalysts: Creating palladium-based alloys can decrease sensitivity to poisons.
Advanced palladium catalysts have been developed using nanoscale engineering. By creating specific surface geometries and compositions, these catalysts show reduced sensitivity to poisons. Additionally, encapsulating palladium particles within protective shells that allow the diffusion of reactants while blocking poisons can enhance their durability.
Platinum Catalyst Poisoning
Platinum catalysts are integral to many industrial processes, especially in the automotive and chemical industries. Despite their robust catalytic properties, platinum catalysts are susceptible to poisoning, which can significantly impair performance. Understanding the factors leading to platinum catalyst poisoning is crucial for maintaining efficiency.
Factors Leading to Platinum Catalyst Poisoning
There are several key factors that contribute to the poisoning of platinum catalysts:
- Sulfur Compounds: Sulfur-containing substances, such as hydrogen sulfide (H2S), bind strongly to platinum surfaces, blocking active sites.
- Carbon Monoxide: As a strong adsorbate, carbon monoxide competes with reactants, hindering the catalytic process.
- Lead and Other Metals: Trace metals can deposit on the catalyst surface, causing irreversible deactivation.
In automotive catalytic converters, exposure to sulfur from fuel can poison platinum catalysts, leading to reduced efficiency in eliminating harmful emissions. A vehicle using leaded gasoline is another example where lead deposits significantly hinder catalyst activity.
Maintaining strict control of feedstock purity can help mitigate catalyst poisoning.
From a mathematical standpoint, the effect of poisons on catalyst performance can be expressed using rate equations. The Langmuir-Hinshelwood model exemplifies this: \[Rate = \frac{k K_A C_A}{1 + K_A C_A + K_i C_i}\]Here:
- \(k\) is the reaction rate constant,
- \(K_A\) is the adsorption equilibrium constant of the primary reactant,
- \(C_A\) is the concentration of the primary reactant,
- \(K_i\) is the adsorption equilibrium constant of the poison,
- \(C_i\) is the concentration of the poison.
Remedies for Platinum Catalyst Poisoning
There are various strategies for tackling the issue of platinum catalyst poisoning:
- Regeneration Techniques: Using thermal treatment or chemical washing can restore some activity by removing the adsorbed poisons.
- Improved Catalyst Design: Developing more resistant alloys or coatings that prevent poison adsorption.
- Feedstock Purification: Employing pre-treatment methods to remove potential poisons before they reach the catalyst.
Innovative approaches in catalyst protection are constantly emerging. One technique involves encapsulating platinum particles in porous shells that allow reactants to enter while preventing poison access. This method enhances durability and maintains high catalytic activity. Additionally, advanced catalysts use nanoscale engineering to minimize active site exposure to poisons, offering a promising solution to common poisoning problems.
How to Prevent Catalyst Poisoning
Preventing catalyst poisoning is essential in maintaining the efficiency and longevity of catalytic systems. By understanding the nature of poisons and implementing appropriate protective measures, you can enhance the performance of catalysts used in various industrial processes.Effective prevention strategies help avert the deterioration of catalysts, ensuring optimal reaction rates and reducing unnecessary costs.
Protective Measures Against Catalyst Poisoning
To safeguard catalysts from being poisoned, several protective measures can be implemented:
- Pretreatment of Reactants: Clean reagents by filtering or chemically treating them to remove impurities that might act as poisons.
- Catalyst Design: Use catalysts that incorporate protective layers or materials resistant to specific poisons.
- Environmental Controls: Maintain reaction environments that minimize exposure to potential poisons. For instance, controlling humidity or temperature can be crucial.
Catalyst poisoning refers to the process by which contaminants bind to active sites on a catalyst, thereby reducing its effectiveness.
Another approach is to use
Adsorbents | Materials that capture and hold poisons before they reach the catalyst. |
Inhibitors | Substances added to the reaction that prevent poison formation or binding. |
In the hydrogenation industry, contaminant gases like H2S can be scrubbed using adsorbents prior to contact with the catalyst, preserving its activity.
Regular monitoring and analysis of feedstock can help in early detection and removal of potential poisons before significant poisoning occurs.
Recent advancements have introduced the use of smart catalysts equipped with sensors capable of detecting and neutralizing poisons in real-time. These systems adjust conditions dynamically to minimize catalytic activity loss. Developing intelligent monitoring systems further aids in understanding poison dynamics and refines preventive strategies. By leveraging advanced technologies, industries are now able to ensure catalysts remain active over extended periods, minimizing production downtimes and costs associated with catalyst replacement.
catalyst poisoning - Key takeaways
- Definition of Catalyst Poisoning: Catalyst poisoning occurs when active sites on a catalyst are blocked by foreign substances, reducing its effectiveness in facilitating chemical reactions.
- Catalyst Deactivation by Poisoning: This process involves the binding of undesired substances to the catalyst, rendering it less effective or inactive.
- Palladium and Platinum Catalyst Poisoning: Palladium and platinum catalysts can be poisoned by substances like sulfur compounds, halides, and hydrocarbons, hindering their catalytic capabilities.
- Types of Catalyst Poisoning: Includes reversible, irreversible, competitive, and non-competitive poisoning, each affecting catalyst activity differently.
- Preventing Catalyst Poisoning: Prevention strategies include pre-treating reactants, designing resistant catalysts, using adsorbents and inhibitors, and maintaining environmental controls.
- Consequences of Catalyst Poisoning: Leads to reduced reaction efficiency, increased operational costs, lower yield of products, and necessitates additional steps for poison management.
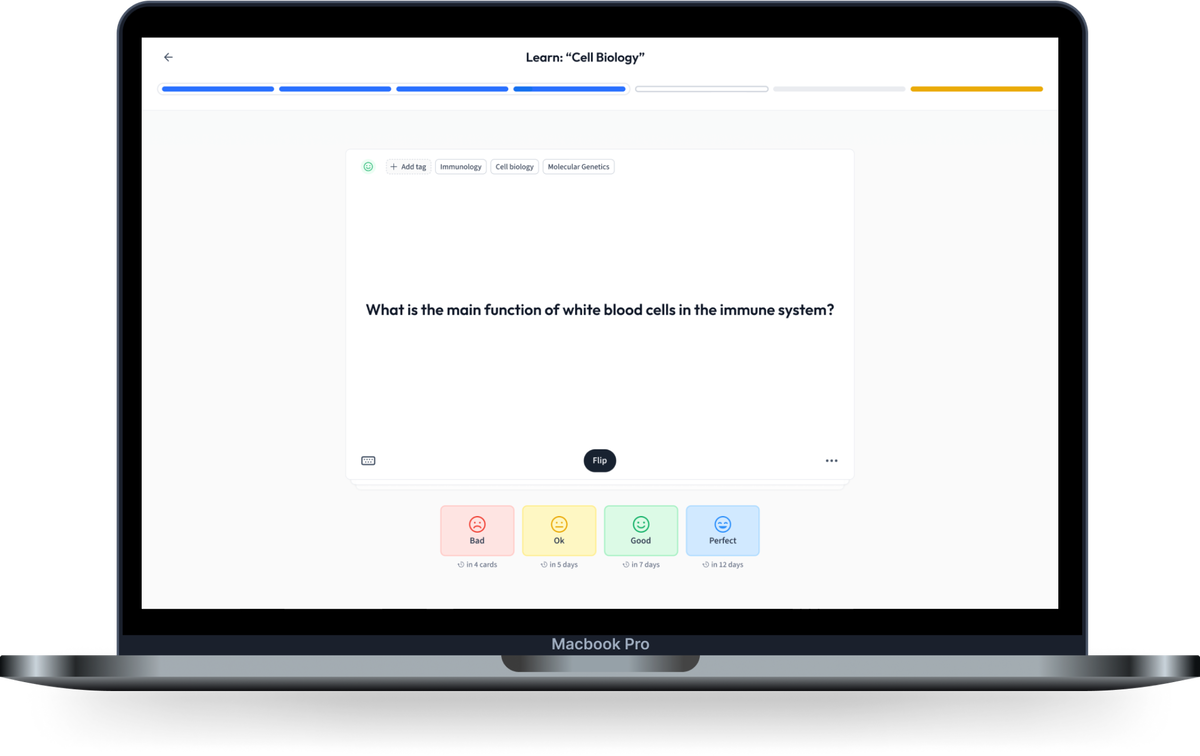
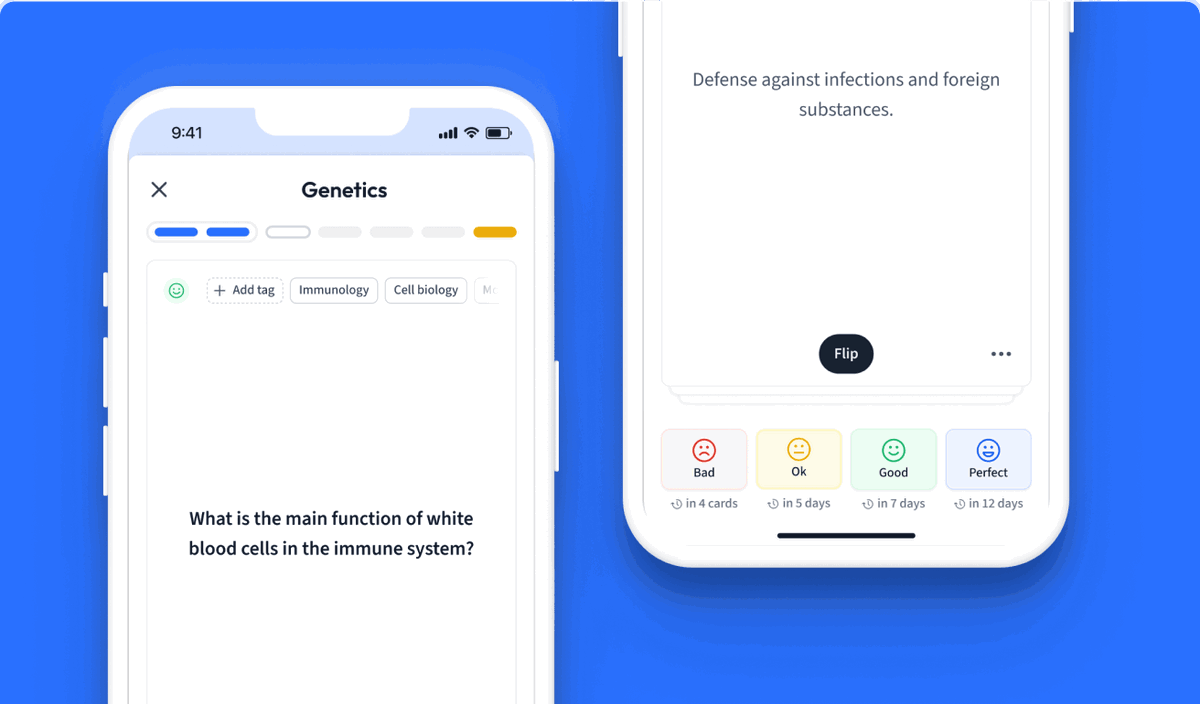
Learn with 12 catalyst poisoning flashcards in the free StudySmarter app
Already have an account? Log in
Frequently Asked Questions about catalyst poisoning
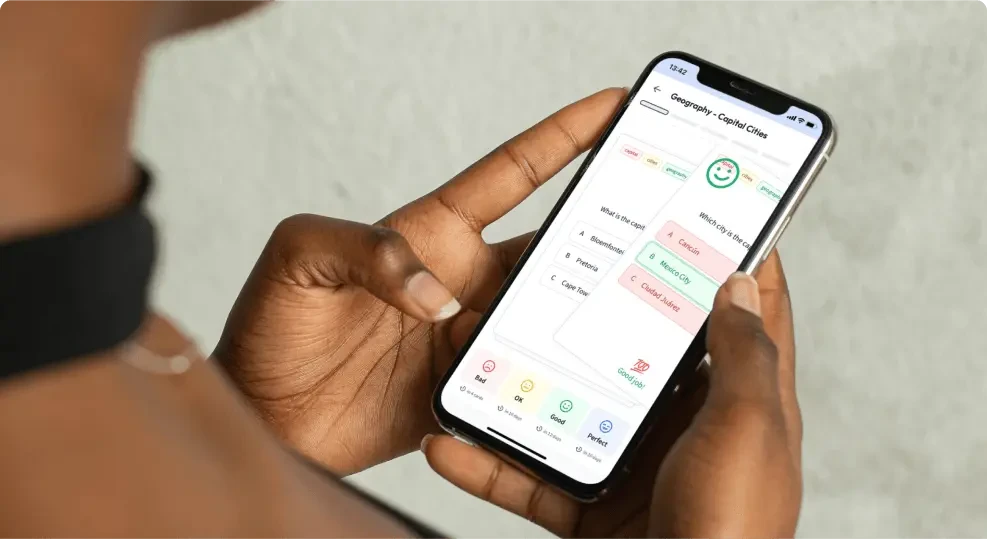
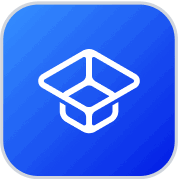
About StudySmarter
StudySmarter is a globally recognized educational technology company, offering a holistic learning platform designed for students of all ages and educational levels. Our platform provides learning support for a wide range of subjects, including STEM, Social Sciences, and Languages and also helps students to successfully master various tests and exams worldwide, such as GCSE, A Level, SAT, ACT, Abitur, and more. We offer an extensive library of learning materials, including interactive flashcards, comprehensive textbook solutions, and detailed explanations. The cutting-edge technology and tools we provide help students create their own learning materials. StudySmarter’s content is not only expert-verified but also regularly updated to ensure accuracy and relevance.
Learn more