Jump to a key chapter
Chemical Kinetics Definition
Understanding chemical kinetics is essential in mastering the reaction processes within the field of chemistry. It involves analyzing the speed or the rate at which a chemical reaction proceeds, as well as the factors affecting this rate. Below are some core concepts and definitions that will help you navigate the basics of chemical kinetics.
What is Chemical Kinetics?
Chemical kinetics is the branch of chemistry that studies the rates of chemical processes. It investigates how different experimental conditions can influence the speed of a chemical reaction. Here are some key aspects you should focus on when studying chemical kinetics:
Reaction Rate: The change in concentration of a reactant or product per unit time, usually measured in \text{M/s} (molarity per second).
To better understand reaction rate, consider the following example. For the decomposition of hydrogen peroxide (\text{H_2O_2}) into water and oxygen: \[2\text{H}_2\text{O}_2 \rightarrow 2\text{H}_2\text{O} + \text{O}_2 \] The rate of this reaction can be expressed as: \[-\frac{d[\text{H}_2\text{O}_2]}{dt} = \frac{d[\text{O}_2]}{dt} \] This equation shows how the concentration of \text{H}_2\text{O}_2 decreases while \text{O}_2 increases over time.
Factors Affecting Reaction Rates
The rate at which a chemical reaction occurs can vary significantly depending on several influencing factors:
- Concentration of Reactants: An increased concentration typically results in an increased reaction rate due to more frequent particle collisions.
- Temperature: Higher temperatures often increase reaction rates as particles gain more energy, resulting in more effective collisions.
- Catalysts: These substances can speed up reactions without being consumed by offering an alternative reaction pathway with a lower activation energy.
- Surface Area: For reactions involving solids, a greater surface area can increase the reaction rate.
One fascinating aspect of chemical kinetics is its application in studying reaction mechanisms. Reaction mechanisms detail the step-by-step sequence of elementary reactions by which a chemical change occurs. By analyzing kinetic data, scientists can propose various possible pathways. These mechanisms play a crucial role in fields ranging from pharmaceuticals to energy production, allowing for the design of processes that are more efficient and environmentally friendly. Additionally, chemical kinetics also helps in understanding phenomena like enzyme kinetics in biological systems, which are vital for life functions.
Chemical Reaction Kinetics Basics
When you delve into the world of chemistry, understanding the basics of chemical reaction kinetics is crucial. Chemical kinetics involves studying the speed of chemical reactions and the factors that influence them. This section covers foundational elements that will prepare you to explore more complex kinetic concepts.
Kinetics of Chemical Reactions
The study of chemical kinetics focuses on reaction rates, which is the speed at which reactants are converted into products. Reaction rates can significantly vary depending on several factors. Here are some central concepts important to chemical kinetics:Factors that affect reaction rates are often examined through kinetics, expanding our understanding and control over reaction dynamics:
Reaction Rate: This is the change in concentration of a reactant or product per unit time, typically measured in \text{M/s} (molarity per second). Mathematically, it's expressed as: \[Rate = -\frac{d[Reactants]}{dt} = \frac{d[Products]}{dt}\]
Consider a simple reaction where nitrogen dioxide reacts with fluorine: \[2\text{NO}_2 + \text{F}_2 \rightarrow 2\text{NO}_2\text{F}\]If the concentration of \text{NO}_2 decreases by \(0.03\, \text{mol/L}\) in \(10\, \text{seconds}\), the rate of the reaction with respect to \text{NO}_2 is:\[Rate = -\frac{\Delta[\text{NO}_2]}{\Delta t} = -\frac{0.03}{10} = 0.003\, \text{M/s}\]
Chemical reactions are influenced by several key factors, each playing a role in determining how quickly or slowly a reaction proceeds. These factors include:
Chemical kinetics also explores complex mechanisms such as consecutive reactions, where the product of one reaction becomes the reactant in the next. Consider the following scheme depicting consecutive reactions:\[A \rightarrow B \rightarrow C\]In such sequences, understanding the rates of each step is vital, as they determine the overall progress and rate of the full reaction. Advanced studies involve the use of differential equations to analyze various such multi-step reactions, which has profound implications in industrial chemistry and biology.
First Order Chemical Kinetics
First order reactions are a specific type of reaction where the rate depends linearly on one reactant concentration. The rate law for a first order reaction can be written as: \[Rate = k[Reactant]\]Where \(k\) is the rate constant.Practically, this means changes in the concentration of reactants are directly proportional to changes in reaction rate. This property can simplify kinetic analysis in many situations.
For a first order reaction, you can determine the time required for the concentration to decrease to half its initial value using the half-life formula: \(t_{1/2} = \frac{0.693}{k}\). This is particularly useful in calculating decay processes.
An example of a first order reaction is radioactive decay. Consider a sample where the radioactive material decreases in concentration over time. If a sample with initial concentration \([N]_0 = 100\, \text{g/mol}\) has a half-life of \(5\, \text{years}\), you can calculate the concentration after \(10\, \text{years}\) (two half-lives): After \(5\, \text{years}: [N] = \frac{1}{2} [N]_0 = 50\, \text{g/mol}\)After \(10\, \text{years}: [N] = \frac{1}{2}\cdot 50\, \text{g/mol} = 25\, \text{g/mol}\)This simple exponential decay is characteristic of first order reactions.
Chemical Kinetics Techniques
Chemical kinetics techniques are essential for understanding how the rate of a reaction can be measured and analyzed. These techniques help in investigating how various conditions affect reaction speeds and the mechanisms involved. The analysis typically involves several sophisticated methods and tools listed below.
Experimental Methods in Kinetics
One of the fundamental approaches in studying chemical kinetics is through experimental methods. These methods allow you to directly measure reaction rates and deduce other kinetic parameters. Here are some of the most commonly employed techniques:
- Stop-Flow Technique: Allows rapid mixing of reactants and immediate measurement of reaction rates, useful for fast reactions.
- Spectroscopy: Utilizes light absorption or emission to track reaction progress, commonly seen in UV-Vis and IR spectroscopy.
- Temperature Jump Method: Involves sudden temperature change to perturb equilibrium, providing insights into reaction kinetics.
To illustrate, consider the use of spectroscopic techniques in analyzing the reaction between hydrogen peroxide and potassium iodide: \[2\text{H}_2\text{O}_2 + 2\text{KI} \rightarrow 2\text{H}_2\text{O} + \text{I}_2 + 2\text{KOH}\] In this experiment, the appearance of iodine (I2) can be monitored using UV-Vis spectroscopy, where increased absorbance at a specific wavelength correlates to more product formation, allowing the reaction rate to be inferred.
Mathematical Models and Rate Equations
Another major component of chemical kinetics involves mathematical models and rate equations. These models help in predicting how reactions proceed over time. Here are some important concepts:
- Rate Laws: Express the relationship between reaction rates and concentrations of reactants. For a general reaction \(aA + bB \rightarrow cC\), the rate law might be: \[Rate = k[A]^m[B]^n\]
- Arrhenius Equation: Describes how rate constants change with temperature: \[k = Ae^{-E_a/RT}\] where \(A\) is the pre-exponential factor, \(E_a\) is the activation energy, \(R\) is the gas constant, and \(T\) is the temperature in Kelvin.
- Integrated Rate Laws: Provide concentration-time relationship for reactions. Example - for a first-order reaction: \[[A]_t = [A]_0e^{-kt}\]
One intriguing aspect of chemical kinetics is its use in developing computer simulations for chemical processes. These simulations can model complex reactions involving many steps or phases that are difficult to analyze experimentally. They incorporate kinetics data, rate laws, and thermodynamic properties to predict reaction behavior under different conditions. Such computational models are essential in areas like atmospheric chemistry, drug development, and material science, allowing researchers to explore theoretical outcomes and optimize reaction conditions effectively. Advanced simulations even integrate quantum chemistry for accuracy in electron-level processes.
Chemical Kinetics Explained Through Examples
Chemical kinetics plays a crucial role in understanding the dynamics of chemical reactions. By examining examples, you can gain insights into how reaction rates are determined and the factors that influence these rates. In this section, we'll explore some key examples in chemical kinetics to solidify your understanding.
Reaction Rate Laws in Practice
Rate laws are mathematical expressions that describe the relationship between the rate of a reaction and the concentration of its reactants. Consider a simple reaction where the rate law is given by:\[Rate = k[A]^m[B]^n\]This expression shows the dependence of the reaction rate on the concentrations of reactants \([A]\) and \([B]\). Here, \(k\) is the rate constant, and \(m\) and \(n\) are the reaction orders determined experimentally.
For a reaction such as the decomposition of ammonia on a platinum surface, the rate law may be:\[2\text{NH}_3 \rightarrow N_2 + 3\text{H}_2\]The observed rate law is:\[Rate = k[\text{NH}_3]^2\]This indicates a second-order reaction with respect to ammonia concentration, meaning the rate is proportional to the square of \([\text{NH}_3]\).
In this example, if the concentration of ammonia doubles, the rate of the reaction quadruples, showcasing the power of kinetics in predicting the behavior of reactions. Understanding this principle is crucial when designing experiments or industrial processes.
Remember that the rate constant \(k\) can change with temperature, as described by the Arrhenius equation: \[k = Ae^{-\frac{E_a}{RT}}\]
Chemical kinetics is not just limited to simple reactions but extends to complex systems. For example, enzyme kinetics explores how enzymes increase reaction rates. Enzymes bind substrates and form a transition state, lowering the energy required for the reaction. The Michaelis-Menten equation models this behavior:\[v_0 = \frac{V_{max}[S]}{K_m + [S]}\]Here, \(v_0\) is the initial reaction rate, \(V_{max}\) is the maximum rate achieved by the system, \(K_m\) is the substrate concentration at which the reaction rate is half of \(V_{max}\), and \([S]\) is the substrate concentration. This model is instrumental in biochemistry and drug design, revealing how slight changes in enzyme or substrate concentration can significantly alter reaction rates.
chemical kinetics - Key takeaways
- Chemical Kinetics Definition: The study of the rates of chemical processes and the factors affecting them.
- Reaction Rate: The change in concentration of a reactant or product per unit time, usually measured in M/s (molarity per second).
- Factors Affecting Reaction Rates: Include concentration of reactants, temperature, catalysts, and surface area.
- First Order Chemical Kinetics: A type of reaction where the rate depends linearly on one reactant concentration.
- Chemical Kinetics Techniques: Methods like stop-flow technique, spectroscopy, and temperature jump method used to study reaction rates.
- Kinetics of Chemical Reactions: Focuses on reaction rates and the impacts of various factors, critical for understanding chemical reaction dynamics.
Learn faster with the 12 flashcards about chemical kinetics
Sign up for free to gain access to all our flashcards.
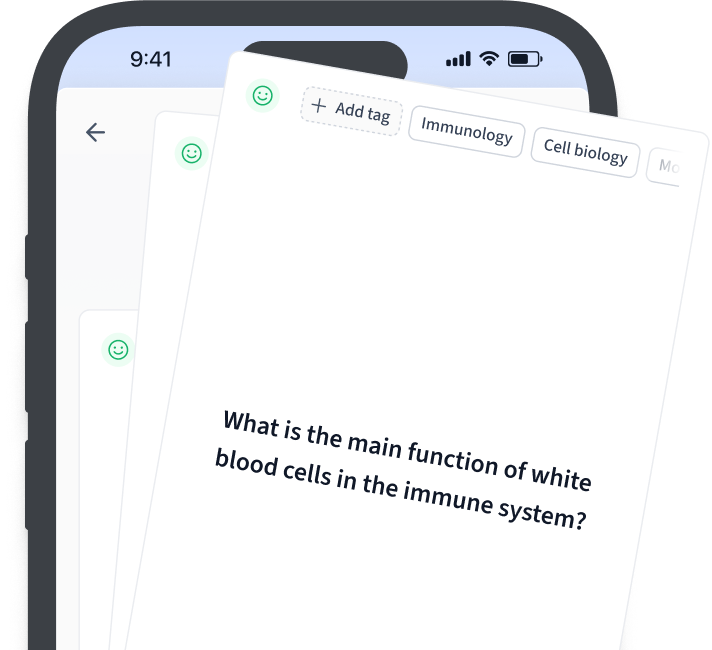
Frequently Asked Questions about chemical kinetics
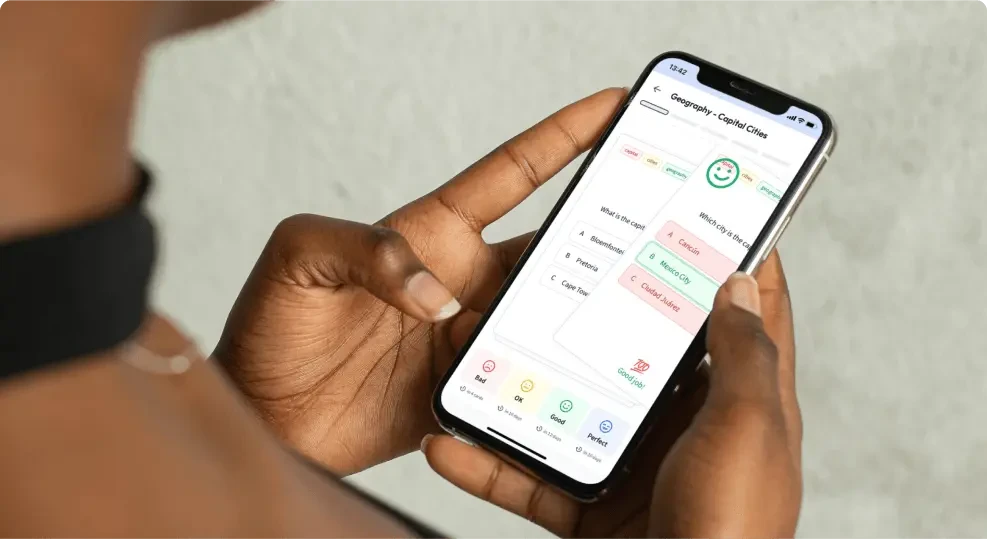
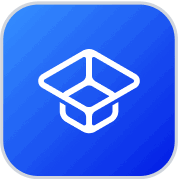
About StudySmarter
StudySmarter is a globally recognized educational technology company, offering a holistic learning platform designed for students of all ages and educational levels. Our platform provides learning support for a wide range of subjects, including STEM, Social Sciences, and Languages and also helps students to successfully master various tests and exams worldwide, such as GCSE, A Level, SAT, ACT, Abitur, and more. We offer an extensive library of learning materials, including interactive flashcards, comprehensive textbook solutions, and detailed explanations. The cutting-edge technology and tools we provide help students create their own learning materials. StudySmarter’s content is not only expert-verified but also regularly updated to ensure accuracy and relevance.
Learn more