Jump to a key chapter
Introduction to Chemical Plant Design
Chemical plant design is a crucial area of engineering that involves developing and optimizing industrial processes to convert raw materials into valuable products. Understanding the key elements of plant design is essential for efficient production, safety, and environmental sustainability.
Basics of Chemical Engineering Plant Design
Chemical engineering plant design begins with comprehensive planning and encompasses various elements to create a functioning chemical facility. The primary objectives include maximizing efficiency, minimizing costs, and ensuring safety and compliance with regulations. Here are some basic elements you should be aware of:
- Process Flow Diagram (PFD): Illustration of major equipment and flow paths for a chemical process.
- Piping and Instrumentation Diagram (P&ID): Detailed diagram showing piping and related components in process flow.
- Material Balance and Energy Balance: Calculations to ensure input equals output in terms of both mass and energy. For example: \( \text{Input Mass} = \text{Output Mass} \) and \( \text{Input Energy} = \text{Output Energy} \).
- Site Layout: Allocation of space for equipment, storage, and other facilities within the plant site.
Chemical Engineering Design: The process of developing specifications and strategies to create a chemical plant capable of producing specified products at desired production rates safely and economically.
A simple example of material balance in a mixing tank involves blending two streams with the following parameters: Stream A: 100 kg/h at 70% concentration, and Stream B: 200 kg/h at 20% concentration. The output stream concentration can be calculated using the formula: \( C_{\text{output}} = \frac{{C_A \cdot F_A + C_B \cdot F_B}}{{F_A + F_B}} \), where \( C \) is concentration and \( F \) is flow rate.
Principles of Chemical Plant Design
Designing a chemical plant relies on several core principles that guide engineers in the configuration and operation of the plant. Some of these principles include:
- Sustainability: Designing plants with minimal environmental impact.
- Efficiency: Optimizing processes to achieve the best possible yield with the least energy input.
- Safety: Ensuring the design includes safety mechanisms to protect workers and surrounding communities.
- Scalability: Creating designs that can easily be scaled up or down depending on demand.
Incorporating these principles into design decisions can significantly affect the plant's operational success.
Safety in chemical plant design not only accounts for the basic installation of protective systems such as relief valves and alarms but also considers more advanced strategies like inherently safer design (ISD). This approach involves minimizing hazards at the design stage by avoiding highly reactive substances and reducing the inventory of hazardous materials on-site. For instance, employing continuous processes over batch reactions can reduce the amount of hazardous inventory, limiting the potential impact of an accident.
Designing a Chemical Plant: Key Steps
Designing a chemical plant involves multiple critical steps aimed at ensuring the plant operates efficiently, safely, and economically. Here are the key steps you need to consider in a chemical plant design:
- Feasibility Study: Conducting initial assessments to determine the viability of the proposed plant.
- Conceptual Design: Developing basic design concepts and deciding on key technologies and processes.
- Detailed Design: Creating detailed drawings and specifications for equipment, processes, and systems.
- Procurement and Construction: Acquiring the necessary equipment and materials and constructing the plant.
- Commissioning and Testing: Initial testing to verify that the plant meets design intentions and can produce desired products.
These steps collectively form the foundation of a structured approach to chemical plant design, ensuring that plant objectives align with production goals and regulatory standards.
Unit Operations in Chemical Plant Design
Unit operations are fundamental in chemical plant design, serving as the building blocks for processing raw materials into finished products. Understanding unit operations is crucial for creating efficient and effective chemical plants.
Overview of Unit Operations
The concept of unit operations is essential in chemical engineering, as they are the individual physical stages in a chemical process. These operations can be categorized into distinct types, primarily involving mass transfer, heat transfer, and mechanical separations.
The main types are:
- Mass Transfer: Involves operations such as distillation, extraction, and absorption. For instance, distillation is used to separate components based on different boiling points.
- Heat Transfer: Includes processes like heat exchangers and evaporators, essential for temperature regulation in chemical reactions.
- Mechanical Separations: Examples include filtration and centrifugation, which separate solids from liquids.
These unit operations enable engineers to model the plant's efficiency and output effectively. To understand their application, consider the role of a distillation column, which is critical for refining processes, where separation of mixtures occurs based on different boiling points.
Distillation is one of the most important unit operations. Its efficiency can be represented by the Fenske equation, which helps in determining the minimum number of stages required for a desired separation in a distillation column. This equation is represented as \[N_{min} = \frac{\log \left( \frac{X_D}{1-X_D} \right) - \log \left( \frac{X_B}{1-X_B} \right)}{\log \alpha} \] where \(N_{min}\) is the minimum number of theoretical stages, \(X_D\) and \(X_B\) are the mole fractions of the more volatile component in the distillate and bottom product, respectively, and \(\alpha\) is the relative volatility of the components.
Key Unit Operations in Designing Chemical Plants
Key unit operations in chemical plant design play a pivotal role in determining the operational success of a plant. Different operations are selected based on the specific requirements of the process, and each comes with its set of design considerations and challenges.
- Mixing: Ensures that chemicals are evenly distributed throughout a process, critical for reactions and reactions uniformity. It involves agitators and mixing tanks.
- Reaction: Chemical reactors are core to production, designed for optimal conversion of reactants to products, often using catalysts to increase reaction rates.
- Separation: Techniques like filtration, centrifugation, and distillation separate components based on different physical or chemical properties.
- Heat Exchange: Managing thermal energy is critical, with heat exchangers transferring heat, maintaining reaction conditions, or recovering waste heat.
An example of a reactor design consideration is the CSTR (Continuous Stirred-Tank Reactor). The material balance equation for a CSTR is given by \( F_{in} \cdot C_{in} = F_{out} \cdot C + V \cdot r \), where \( F_{in} \) and \( F_{out} \) are the inflow and outflow rates, \( C_{in} \) and \( C \) are inlet and outlet concentrations, \( V \) is volume, and \( r \) is the reaction rate.
Selecting the optimal unit operations for your chemical plant design involves balancing trade-offs like energy efficiency, capital cost, and operational complexity.
Process Flow Diagrams in Chemical Engineering
In chemical engineering, Process Flow Diagrams (PFDs) serve as essential visual tools to map out the major components of a chemical plant. You can use PFDs to understand the flow of materials and energy within a plant, guiding the design and operation of processes.
Importance of Process Flow Diagrams
Process Flow Diagrams are more than just schematic representations; they are crucial for effective communication and design validation in chemical engineering. Here's why they matter:
- Visual Representation: PFDs provide a clear, visual overview of the major equipment and the general flow paths between them, allowing for easier understanding and communication.
- Process Insight: They offer insights into the sequence of chemical processes, highlighting key steps and potential areas for improvement.
- Efficiency Optimization: By analyzing PFDs, you can identify bottlenecks, redundancy, and inefficiencies in the process.
- Cost Estimation: Detailed PFDs aid in cost estimation by outlining necessary equipment and materials.
- Safety Consideration: They help in ensuring that safety measures are integrated into the design, identifying areas requiring protective equipment.
For instance, visualizing the flow through a distillation column in a PFD can highlight how different components branch off at various stages.
A PFD will typically include equipment such as reactors, distillation columns, heat exchangers, and separators. Each piece of equipment is tagged with a unique identifier. Tagging helps to coordinate maintenance and operation, which can significantly reduce downtimes and enhance performance. Moreover, the PFD distinguishes between different phases—solid, liquid, vapor—or chemical composition flowing through the process, often represented by arrows and other symbology.
Creating Effective Process Flow Diagrams
Designing Process Flow Diagrams requires precision and a thorough understanding of the process. To create effective PFDs, follow these guidelines:
- Identify Key Components: Begin by identifying all major equipment and their purpose. Ensure their placement reflects the actual process sequence.
- Define Process Streams: Clearly define all input and output streams, including their direction and connections. Label stream compositions and conditions if needed.
- Use Standard Symbols: Apply industry-standard symbols for equipment and components to maintain clarity and uniformity. This helps avoid misunderstandings, especially among interdisciplinary teams.
Component | Symbol |
Distillation Column | Vertical Cylinder |
Heat Exchanger | Two Horizontal Lines with Arrows |
Error-checking: Regularly verify the PFDs to ensure they accurately reflect the design intentions and incorporate feedback from cross-functional teams.
An example of a process flow diagram might include a simple reaction system where an input feed is heated, reacted, and then cooled before it exits the system. This process could be represented as: Input Stream → Heater → Reactor → Cooler → Output Stream. Mathematically, the heat balance around the reactor might be expressed as \( Q = m \cdot C_p \cdot (T_{out} - T_{in}) \), where \( Q \) is the heat added, \( m \) is mass flow rate, \( C_p \) is specific heat capacity, and \( T_{out} \) and \( T_{in} \) are outlet and inlet temperatures.
Using computer-aided design (CAD) software can significantly streamline the creation and modification of process flow diagrams.
Advanced Topics in Chemical Engineering Plant Design
Advanced topics in chemical engineering plant design delve deep into considerations that improve process efficiency, safety, and sustainability. As you explore these areas, you'll gain insights into how to optimize and safeguard chemical plants effectively.
Optimization in Chemical Plant Design
Optimization in chemical plant design is vital to enhance operational efficiency and cost-effectiveness. It involves the systematic process of improving various aspects of plant operations, such as input resources, energy consumption, and product yield.
Key Optimization Goals:
- Maximizing Production: Increase the output while maintaining or reducing input resources. Utilize precise mathematical models to predict plant behavior under different scenarios.
- Reducing Energy Consumption: Employ energy-efficient technologies and optimize heat management systems to cut down on energy usage.
- Minimizing Waste: Implement waste reduction and recycling strategies to enhance sustainability.
The mathematical approach often used in optimization is known as linear programming, which involves an objective function that can be minimized or maximized. For example, consider the profit maximization equation: \( Z = c_1x_1 + c_2x_2 + \text{...} + c_nx_n \) subject to various resource constraints defined by inequalities like \( a_{11}x_1 + a_{12}x_2 + \text{...} + a_{1n}x_n \le b_1 \).
Suppose a chemical plant produces two products. The profit from product A is $80 per unit, and from product B is $120 per unit. The plant's task is to maximize profit limited by resource constraints. The constraints and demands are modeled using the equations \( 3x_1 + 2x_2 \le 12 \) and \( 2x_1 + x_2 \le 8 \). Solving these using linear programming can yield the optimal production schedule for maximum profit.
Incorporating simulation software can assist in visualizing optimization efforts, providing a clearer picture of potential improvements.
Beyond simple optimization, consider leveraging multi-objective optimization techniques, which involve simultaneously optimizing two or more conflicting objectives, such as minimizing costs while maximizing energy efficiency. This method often uses Pareto efficiency to find an ideal balance. Mathematically, this balance is represented by a Pareto frontier where no objective can be improved without degrading another.
Safety Considerations in Designing Chemical Plants
Safety is a paramount concern in the design of chemical plants, requiring a comprehensive approach to mitigate risks associated with chemical processes. Effective safety considerations can prevent accidents, protect the environment, and ensure regulatory compliance.
Critical Safety Measures:
- Hazard Identification: Use qualitative and quantitative methods to assess potential hazards, such as fault tree analysis (FTA) or hazard and operability study (HAZOP).
- Risk Assessment: Quantify the probabilities and consequences of process-related risks using tools like Layer of Protection Analysis (LOPA).
- Safety Systems: Implement automated control systems, alarms, and emergency shutdown systems to manage abnormal conditions.
- Inherently Safer Design: Modify designs to eliminate hazards rather than control them, for example, using less hazardous materials.
Mathematically, risk can be assessed using the risk matrix, where risk \( R \) is evaluated as a function of probability \( P \) and consequence \( C \, R = P \times C \).
Inherently Safer Design (ISD): A methodology that focuses on eliminating or significantly reducing hazards instead of using controls or protective measures to mitigate them.
chemical plant design - Key takeaways
- Chemical plant design: Involves developing and optimizing processes to convert raw materials into products, focusing on efficiency, safety, and sustainability.
- Chemical engineering plant design: Begins with planning to maximize efficiency, minimize costs, and ensure safety compliance, including tools like Process Flow Diagrams (PFDs).
- Principles of chemical plant design: Include sustainability, efficiency, safety, and scalability for optimal plant operation and environmental impact.
- Designing a chemical plant: Follows steps like feasibility study, conceptual design, detailed design, procurement, construction, and commissioning.
- Unit operations in chemical plant design: Essential building blocks, include mass transfer, heat transfer, and mechanical separations for process efficiency.
- Process flow diagrams in chemical engineering: Serve as visual tools to map out major plant components, crucial for design validation and operational optimization.
Learn faster with the 12 flashcards about chemical plant design
Sign up for free to gain access to all our flashcards.
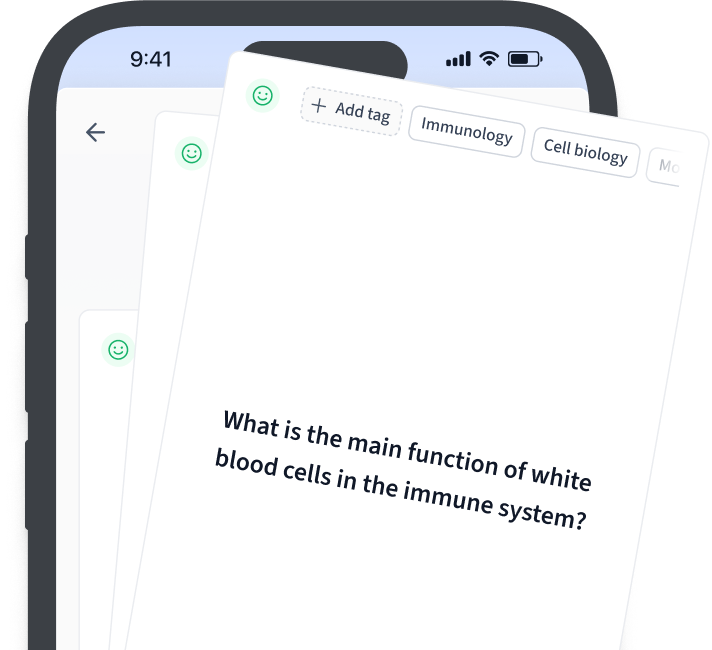
Frequently Asked Questions about chemical plant design
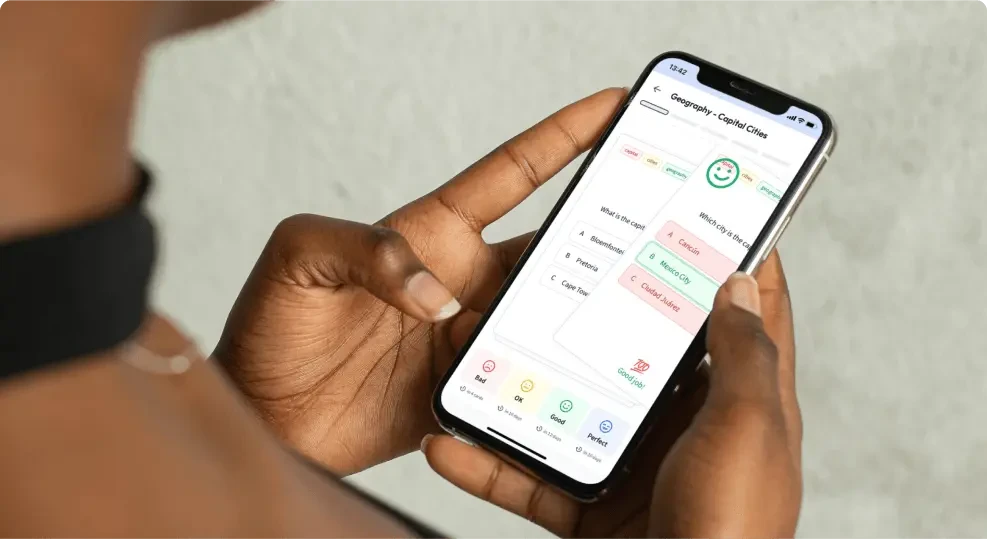
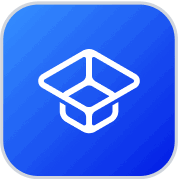
About StudySmarter
StudySmarter is a globally recognized educational technology company, offering a holistic learning platform designed for students of all ages and educational levels. Our platform provides learning support for a wide range of subjects, including STEM, Social Sciences, and Languages and also helps students to successfully master various tests and exams worldwide, such as GCSE, A Level, SAT, ACT, Abitur, and more. We offer an extensive library of learning materials, including interactive flashcards, comprehensive textbook solutions, and detailed explanations. The cutting-edge technology and tools we provide help students create their own learning materials. StudySmarter’s content is not only expert-verified but also regularly updated to ensure accuracy and relevance.
Learn more