Jump to a key chapter
Electrocatalysis Definition
Electrocatalysis refers to the enhancement of chemical reactions using an electric field in the presence of a catalyst. This process is integral to electrochemical cells and is crucial in fields such as fuel cells, batteries, and water splitting for hydrogen production. The catalyst, often a metal or metal oxide, lowers the energy barrier for the reaction, allowing it to proceed more efficiently and selectively.
Basic Principles of Electrocatalysis
The basic principles of electrocatalysis center around the interaction between the catalyst and the reactants within an electrochemical cell. To grasp these principles, consider the following components:1. **Electroactive Sites**: These are specific regions on the catalyst's surface where the reaction occurs. For electrocatalysis, maximizing these sites boosts the overall efficiency.2. **Adsorption and Desorption**: The reactants must first adsorb onto the catalyst's surface. This is followed by a chemical transformation and finally, the desorption of the reaction products.3. **Charge Transfer**: Electrons must be efficiently transferred between the electrode and reactants. This is often represented by the Tafel equation, which describes the rate of an electrochemical reaction at a metal surface: \[ \text{j} = \text{j}_0 \times e^{\frac{\text{nF}}{\text{RT}}(\text{E} - \text{E}_0)} \]where:- **j** is the current density (A/m²)- **j_0** is the exchange current density- **n** is the number of electrons transferred- **F** is the Faraday constant- **R** is the gas constant- **T** is the temperature in Kelvin- **E** is the electrode potential- **E_0** is the standard electrode potential.4. **Mass Transport**: Efficient movement of reactants to the catalyst surface and products away from it is vital for maintaining reaction rates.
Consider the hydrogen evolution reaction (HER) on a platinum catalyst in an acidic medium:\[ \text{H}^+ + \text{e}^- \rightarrow \text{H}_2 \] In this reaction, the platinum catalyst facilitates the reduction of protons to molecular hydrogen, showcasing its high electrocatalytic efficiency.
In electrocatalysis, the efficiency of a catalyst can often be gauged by its 'overpotential', which is the extra energy required beyond the theoretical potential to drive a reaction at a desired rate.
Electrocatalysis plays a crucial role in sustainable energy solutions by enabling efficient energy conversion processes. For example, in a fuel cell, electrocatalysis allows the conversion of chemical energy stored in fuels into electricity with high efficiency and minimal emissions. The design of advanced catalysts is crucial as it determines not only the rate of the reaction but also the selectivity and stability of the cell. Recent advances in nanotechnology have allowed the engineering of catalysts at the atomic level, optimizing their electronic properties and providing insights into reaction mechanisms. The development of electrocatalysts that use abundant non-precious metals is a key area of research, aimed at reducing costs while maintaining performance.
Electrocatalysis Mechanism
The mechanism of electrocatalysis involves specific interactions between catalysts and reactants that enhance the efficiency of electrochemical reactions. Understanding the underlying process is essential for improving applications in energy conversion and storage.
How Electrocatalysis Works
Electrocatalysis functions through a series of well-defined steps that facilitate the conversion of reactants to products with minimal energy loss. Here's a breakdown of the process:
- Electrode Surface Interaction: Reactants are absorbed onto the surface of an electrode that is coated with a catalyst. This step is crucial as it positions the molecules at the active sites for reaction progress.
- Electron Transfer: A key feature of electrocatalysis is the transfer of electrons through the metal catalyst. This is quantitatively described using the Butler-Volmer equation:\[ j = j_0 \left( e^{\frac{\alpha nF}{RT}(E - E_0)} - e^{-\frac{(1-\alpha)nF}{RT}(E - E_0)} \right) \]where:- **j** is the current density- **j_0** is the exchange current density- **\alpha** is the charge transfer coefficient- **n** is the number of electrons involved- **F** is Faraday's constant- **R** is the gas constant- **T** is the temperature- **E** is the electrode potential- **E_0** is the standard electrode potential.
- Intermediary Formation: Often involves the formation of reaction intermediates that help reduce activation energy.
- Desorption of Products: The final chemical products are released from the surface, completing the catalytic cycle.
An example of electrocatalysis is the oxygen reduction reaction (ORR) that occurs in fuel cells:\[ \text{O}_2 + 4\text{H}^+ + 4\text{e}^- \rightarrow 2\text{H}_2\text{O} \]This reaction is slow without a catalyst, but becomes highly efficient with a platinum-based electrocatalyst.
The performance of electrocatalysts is often assessed by their turnover frequency (TOF), which measures the number of times a site on the catalyst converts reactants to products per second.
The precise control of atomic arrangements in catalysts can dramatically influence their electrocatalytic performance. Atomic-scale precision offers a unique opportunity to tune electronic structures and reaction pathways for more efficient energy conversion. This can be seen in the emerging field of single-atom catalysts (SACs), which involve dispersing isolated metal atoms onto support materials. Such innovations are paving the way for significant advancements in photochemical applications and low-temperature catalytic processes.
Role in Chemical Reactions
In the realm of chemical reactions, electrocatalysis serves as a bridge between electrical and chemical energy conversion. Its role is pivotal in several important contexts:
- Fuel Cells: Electrocatalysis is integral in fuel cells where it facilitates reactions such as the oxygen reduction reaction (ORR) and hydrogen oxidation reaction (HOR), both crucial for power generation.
- Batteries: Advanced batteries leverage electrocatalysis for improved charge and discharge dynamics, maximizing their energy density and cycle life.
- Water Splitting: A key process for sustainable hydrogen production, electrocatalysis aids in both the hydrogen evolution reaction (HER) and oxygen evolution reaction (OER).
- Carbon Dioxide Reduction: Converts CO2 into valuable chemicals and fuels, offering a promising pathway for carbon capture and utilization.
The integration of electrocatalysis in renewable energy technologies is foundational for the development of zero-carbon energy systems. Research into non-precious metal catalysts and materials such as carbon nanostructures seeks to reduce reliance on expensive noble metals like platinum. Furthermore, tailored nanostructured catalysts, which are synthesized to have high surface area and optimal active site exposure, are leading the charge in achieving more sustainable and economically viable catalytic systems. As these technologies evolve, the scalability of electrochemical devices and their commercial viability will be significantly influenced by these advancements in electrocatalysis.
Electrocatalysis Water Splitting
Water splitting is a significant process in the quest for clean energy, where electrocatalysis plays a pivotal role. This process involves separating water into its constituent elements, hydrogen, and oxygen, using an electrical current in the presence of a catalyst. Understanding this process is critical for developing sustainable energy technologies.
Hydrogen Production
Hydrogen production through electrocatalysis involves the hydrogen evolution reaction (HER), a crucial component of water splitting. In this reaction, protons (\text{H}^+) are reduced to form molecular hydrogen (\text{H}_2) at the cathode of an electrochemical cell. This is typically facilitated by a catalyst, such as platinum, known for its high efficiency and low overpotential. The overall reaction is expressed as follows:\[ 2\text{H}^+ + 2\text{e}^- \rightarrow \text{H}_2 \]This reaction is essential for generating clean hydrogen fuel, which can be used in various applications, from fuel cells to industrial processes. The efficiency and sustainability of hydrogen production can greatly benefit from advancements in electrocatalyst materials that reduce costs and enhance performance.A table of common catalysts used in HER reactions and their characteristics:
Catalyst | Material | Characteristic |
Platinum | Metal | High efficiency, low overpotential |
Nickel-Molybdenum | Alloy | Cost-effective, good efficiency |
Cobalt-Phosphide | Compound | Moderate efficiency, stable |
An efficient HER process can be achieved using a nickel-molybdenum foam electrocatalyst. It exhibits enhanced durability and activity for the hydrogen evolution reaction in alkaline solutions, where the overall reaction kinetics are improved compared to conventional catalysts.
Developments in nanostructured catalysts can significantly reduce the amount of precious metal needed in electrocatalysis, making technologies more accessible and sustainable.
Breaking down the HER mechanism reveals that it primarily consists of two types of steps: Volmer and either Heyrovsky or Tafel steps. The Volmer step involves the initial reduction of a proton through adsorption on the catalyst's surface:\[ \text{H}^+ + \text{e}^- + \text{Cat-Site} \rightarrow \text{H-Cat-Site} \]Here, \text{Cat-Site} represents an active site on the catalyst surface. This is followed by either the Heyrovsky step, where the adsorbed hydrogen reacts with another proton and electron to form \text{H}_2:\[ \text{H-Cat-Site} + \text{H}^+ + \text{e}^- \rightarrow \text{H}_2 + \text{Cat-Site} \]or the Tafel step, where two adsorbed hydrogen atoms combine:\[ 2\text{H-Cat-Site} \rightarrow \text{H}_2 + 2\text{Cat-Site} \]Recent advances focus on optimizing these intermediate reactions, reducing activation energy, and enhancing overall system efficiency.
Environmental Impact
The environmental impact of electrocatalysis in water splitting is profound as it offers a path toward renewable and clean hydrogen production. Utilizing water as a primary feedstock for hydrogen avoids the carbon emissions associated with traditional fossil fuel-based methods, contributing significantly to reducing the overall carbon footprint. Such technologies could substantially mitigate air pollution and global warming when integrated into the energy systems.Benefits of hydropower derived from electrocatalytic water splitting include:
- Renewable Energy Source: Utilizes water and electricity from renewable sources like wind or solar to produce hydrogen, offering a sustainable cycle.
- No CO2 Emissions: Hydrogen combustion results in water vapor, not carbon dioxide, reducing greenhouse gas emissions.
- Energy Storage: Hydrogen acts as an energy carrier, effectively storing excess energy from renewable sources.
A city incorporating hydrogen fuel cells into its public transportation system can massively lower its carbon footprint. Hydrogen buses emit only water vapor and heat, contrasting sharply with diesel or gasoline vehicles.
Some electrocatalysts may contain materials that are non-recyclable or environmentally hazardous, making the development of sustainable and green catalysis a priority for researchers.
While hydrogen production offers numerous environmental benefits, associated challenges such as the sourcing and disposal of catalyst materials remain. Rare and precious metals, though effective in catalytic reactions, are limited and often involve environmentally detrimental mining practices. The push for catalysts based on common earth-abundant materials or those that can be regenerated through green chemistry processes is critical. Life-cycle assessments of electrocatalytic systems that encompass all stages from production to disposal will ensure that these clean energy technologies truly offer net positive environmental impacts.
Electrocatalysis Applications
Electrocatalysis is an essential concept that finds applications across various industries, enhancing the efficiency of numerous processes. Its ability to accelerate chemical reactions through the use of catalysts makes it invaluable in settings ranging from industrial manufacturing to renewable energy production.
Industrial Uses
Electrocatalysis plays a key role in various industrial applications by facilitating reactions that are otherwise slow or energy-intensive. Here are some examples:
- Ammonia Production: The Haber process, which synthesizes ammonia from nitrogen and hydrogen, can be enhanced using electrocatalysis by optimizing reaction conditions and catalyst surfaces.
- Chlor-Alkali Process: Used for producing chlorine and caustic soda from saltwater, electrocatalysis can improve efficiency and reduce energy consumption.
- Organic Synthesis: Electrocatalysis aids in the selective oxidations and reductions of organic compounds, crucial for pharmaceuticals and fine chemicals.
In the chlor-alkali industry, the membrane cell process can use electrocatalysts to separate chlorine and sodium hydroxide efficiently, reducing the energy expenditure compared to older methods like mercury cells.
Using electrocatalysis in industrial processes not only boosts reaction speeds but can also lead to more environmentally friendly production pathways by reducing toxic byproducts.
In the realm of organic synthesis, electrocatalysis offers the ability to direct the reactivity of molecules through precise control of electron flow. This is especially useful for creating high-value pharmaceuticals that require exact molecular configurations. Energy and atom efficiency, as well as the reduction of hazardous reagents, align electrocatalyst applications with the principles of green chemistry. Insight into the electronic structure and potential distribution within the catalysts provides detailed understanding and further optimization of these reactions.
Renewable Energy Production
In the field of renewable energy production, electrocatalysis is a cornerstone technology. It is employed across several domains including:
- Fuel Cells: Convert chemical energy from fuels directly into electricity. Electrocatalysts enhance reaction rates in components such as the fuel cell's anode and cathode, supporting a range of applications from vehicles to stationary power sources.
- Photovoltaic-Hybrid Systems: Incorporating electrocatalytic processes to store solar energy chemically, bridging gaps when sunlight is not available.
- Carbon Dioxide Reduction: Electrocatalysts can convert CO2 into useful hydrocarbons, addressing both energy needs and reducing emissions.
A PEM (Proton Exchange Membrane) fuel cell uses platinum-based electrocatalysts to facilitate the conversion of chemical energy in hydrogen into electrical energy with high efficiency.
The role of electrocatalysis in photovoltaics enables the integration of solar panels with energy storage systems, offering a viable solution to fluctuating energy demands and conditions.
The development of electrocatalysts for CO2 reduction is particularly promising for creating a circular carbon economy. State-of-the-art catalysts are being refined to enhance selectivity, allowing for the production of complex hydrocarbon chains directly from CO2 and water. Research efforts focus on increasing the stability and efficiency of these reactions, driven by concerns over CO2 emissions and climate change. Upcoming materials, such as those derived from metal-organic frameworks (MOFs) and dendrimers, are demonstrating great potential in facilitating effective CO2 conversion processes.
Advantages of Electrocatalysis
The advantages of electrocatalysis are manifold, providing significant improvements to reaction processes across both industrial and renewable applications. Key benefits include:
- Higher Reaction Rates: By lowering the activation energy, electrocatalysis speeds up chemical reactions.
- Improved Selectivity: Targeted reaction pathways reduce byproduct formation, increasing yield and purity.
- Energy Efficiency: Reduced energy consumption in driving chemical processes, leading to lower operational costs.
- Environmental Benefits: By enabling cleaner production methods and integrating with renewable resources, electrocatalysis contributes to sustainability and reduced emissions.
In the context of battery technology, electrocatalysis can be used to enhance the performance and longevity of lithium-ion batteries, contributing to better energy storage solutions.
The widespread adoption of electrocatalytic processes can drive substantial economic benefits owing to reduced energy demands and heightened process efficiencies.
The pursuit of advanced electrocatalytic systems presents significant innovation opportunities, particularly in the optimization of earth-abundant materials. For instance, the exploration of transition metal phosphides and carbides as well as layered double hydroxides (LDHs) provides cheaper and readily available alternatives to noble metals like platinum. These developments aim at customizing electronic properties and promoting active site availability to improve catalytic action. Furthermore, integration with digital platforms, such as artificial intelligence, is paving the way for rapid prediction and testing of electrocatalyst performance under various conditions.
electrocatalysis - Key takeaways
- Electrocatalysis Definition: Enhancement of chemical reactions using electrical fields with catalysts in electrochemical cells.
- Electrocatalysis Mechanism: Involves adsorption of reactants, charge transfer, formation of intermediates, and desorption of products.
- Electrocatalysis Water Splitting: Utilized for hydrogen production by splitting water into hydrogen and oxygen using a catalyst.
- Electrocatalysis Applications: Found in fuel cells, batteries, water splitting, and CO2 reduction for various industrial and renewable energy uses.
- Electrocatalysis Processes: Processes involve reactions like oxygen reduction and hydrogen evolution in electrochemical devices.
- Advantages of Electrocatalysis: Offering higher reaction rates, improved selectivity, energy efficiency, and environmental benefits.
Learn faster with the 12 flashcards about electrocatalysis
Sign up for free to gain access to all our flashcards.
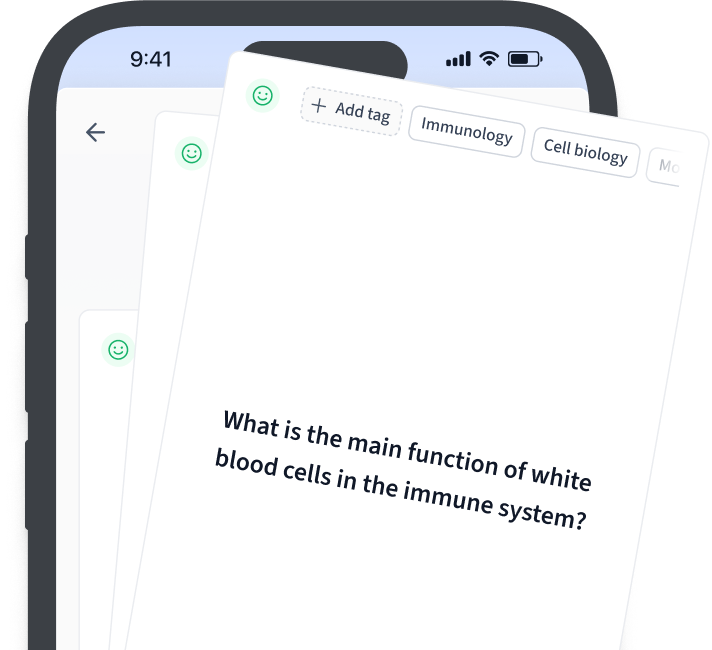
Frequently Asked Questions about electrocatalysis
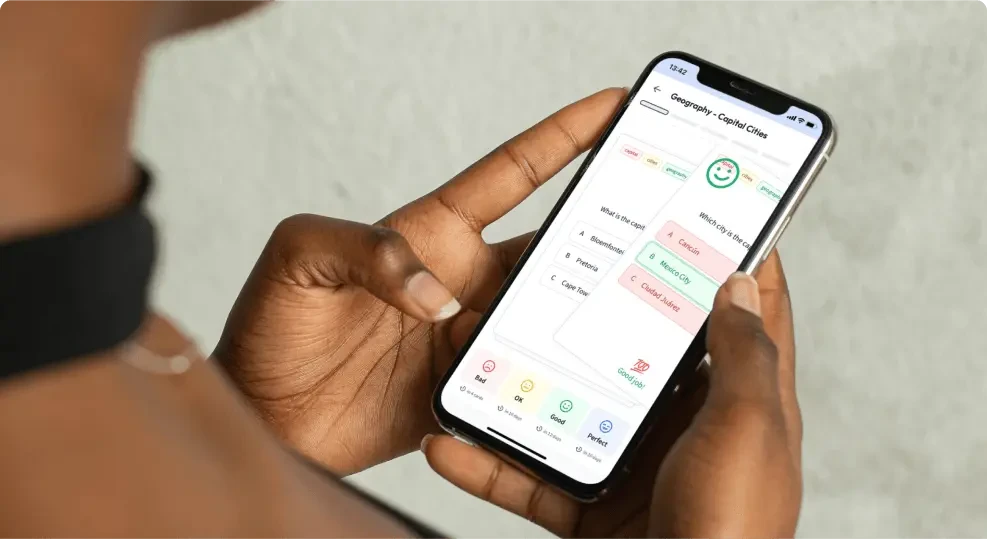
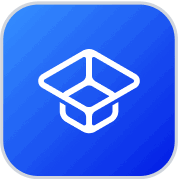
About StudySmarter
StudySmarter is a globally recognized educational technology company, offering a holistic learning platform designed for students of all ages and educational levels. Our platform provides learning support for a wide range of subjects, including STEM, Social Sciences, and Languages and also helps students to successfully master various tests and exams worldwide, such as GCSE, A Level, SAT, ACT, Abitur, and more. We offer an extensive library of learning materials, including interactive flashcards, comprehensive textbook solutions, and detailed explanations. The cutting-edge technology and tools we provide help students create their own learning materials. StudySmarter’s content is not only expert-verified but also regularly updated to ensure accuracy and relevance.
Learn more