Jump to a key chapter
Energy Barriers in Catalysis Overview
Energy barriers play a crucial role in catalysis, influencing the speed and feasibility of chemical reactions. In catalysis, a reaction proceeds more smoothly when an energy barrier, often known as the activation energy, is lower. Catalysts work by reducing these energy barriers, allowing reactions to occur at a faster rate and under more favorable conditions. Understanding these concepts is essential for grasping how complex reactions can be manipulated and controlled.
The Concept of Activation Energy
In chemical kinetics, the activation energy (Ea) is the minimum energy required for a chemical reaction to occur. It represents the energy difference between the reactants and the transition state. This energy can be visualized as a peak on the reaction’s potential energy surface.
Imagine energy barriers as hills that reactants must 'climb' for a reaction to take place. The height of these hills is determined by the activation energy. Catalysts are substances that effectively 'lower' these hills, making it easier for molecules to reach the transition state.
Consider a simple reaction where molecule A converts to molecule B. Without a catalyst, the energy barrier might be represented as:\[A \rightarrow A^* \rightarrow B\]Where \(A^*\) is the transition state. With a catalyst, the energy of \(A^*\) is lowered, thus requiring less energy for the reaction:\[A \rightarrow A^{**} \rightarrow B\]In this scenario, \(A^{**}\) is the new, lower-energy transition state.
Types of Catalysts and Their Impact
Different types of catalysts include enzyme catalysts (biocatalysts), heterogeneous catalysts, and homogeneous catalysts. Each type affects energy barriers uniquely:
- Enzyme catalysts: Often found in biological systems, these are highly specific and efficient. Enzymes lower activation energies through various mechanisms like binding substrates in an optimal orientation.
- Heterogeneous catalysts: These operate in a different phase than the reactants (e.g., a solid catalyst in a liquid reaction). They provide a surface for the reactant molecules to adsorb, thus altering the energy barrier.
- Homogeneous catalysts: Existing in the same phase as the reactants, these catalysts typically form intermediary compounds that lower the overall activation energy of the reaction.
Did you know? The Nobel Prize in Chemistry 2007 was awarded for studies on chemical processes on solid surfaces, contributing to the understanding of energy barriers in catalysis.
Mathematics of Energy Barriers
Mathematically, energy barriers relate to the Arrhenius equation, which expresses the rate constant \(k\) of a reaction in terms of the activation energy \(Ea\), the gas constant \(R\), and the temperature \(T\):\[k = A e^{-Ea/RT}\]Where \(A\) is the pre-exponential factor, reflecting the frequency of collisions and the probability that collisions result in a reaction. By examining this formula, you can see how increasing the temperature \(T\) or decreasing the activation energy \(Ea\) leads to an increase in reaction rate \(k\). This quantifies the impact of catalysts in lowering energy barriers: by reducing \(Ea\), they enhance the likelihood and speed of reactions.
A catalyst's impact is often visualized on an energy profile diagram, showing a decrease in the peak that represents activation energy.
Activation Energy in Catalysis
In the field of catalysis, an important concept is that of activation energy. This energy barrier must be overcome for chemical reactions to occur. By studying activation energies, you can gain insights into how catalysts make reactions feasible under various conditions.
Understanding Activation Energy
Activation energy, denoted as \( Ea \), is the minimum energy barrier that must be overcome for a chemical reaction to proceed from reactants to products. It is often depicted as a peak on a reaction coordinate diagram, representing the transition state.
Activation energy is crucial because it determines the rate at which a reaction can occur. If the energy is too high, few molecules reach the transition state at a given temperature, slowing the reaction rate. Catalysts are essential because they lower this energy barrier, enabling more molecules to achieve the necessary energy level for reactions.
Consider a reaction where primitive reactants \( A \) convert to products \( B \). The Arrhenius equation demonstrates how activation energy affects the reaction rate \( k \):\[k = A e^{-Ea/RT}\]where:
- \( A \) is the pre-exponential factor, representing collision frequency.
- \( R \) is the universal gas constant.
- \( T \) is the temperature in Kelvin.
Let's consider enzymes, biological catalysts that drastically decrease activation energies. Enzymes work by positioning reactants perfectly and stabilizing the transition state, facilitating reactions that would otherwise be too slow to sustain life. Enzymes have different active sites that retain reactants, aligning them in a manner that allows transitions at lower activation energies.
Role in Catalytic Reaction Pathways
Catalysts impact not only the rate of reactions but also the pathways these reactions take. By providing alternative routes with lower activation energies, catalysts enable more efficient chemical processes. This alteration of reaction pathways changes both the energy landscape and the speed at which reactants transform into products.
A reaction pathway is a sequence of steps that take place during a chemical reaction. Each step involves the formation and breaking of chemical bonds and has its own associated activation energy.
In an industrial process converting \( X \) to \( Y \), a non-catalyzed pathway may require overcoming an energy barrier of 200 kJ/mol. The catalyst might offer an alternative path with steps requiring energy barriers of 50, 100, and 60 kJ/mol instead. Table representation:
Non-catalyzed Pathway | 200 kJ/mol |
Catalyzed Pathway Step 1 | 50 kJ/mol |
Catalyzed Pathway Step 2 | 100 kJ/mol |
Catalyzed Pathway Step 3 | 60 kJ/mol |
Catalysts provide alternative pathways that often involve intermediates not present in the uncatalyzed reaction, thus offering unique and lower-energy steps.
Catalysis Mechanisms and Energy Barriers
Catalysis involves the acceleration of chemical reactions by substances known as catalysts, which reduce the activation energy or energy barriers required for the reaction. By understanding the various mechanisms in catalysis, you can see how these energy barriers are effectively lowered, enabling reactions to occur more rapidly and efficiently.
Key Catalysis Mechanisms
Catalysis can be categorized into several mechanisms, each with distinct ways of affecting energy barriers and reaction pathways. Here are some of the major types:
- Enzymatic Catalysis: Enzymes, which are biological catalysts, lower energy barriers by positioning substrates in a way that stabilizes the transition state.
- Heterogeneous Catalysis: Involves catalysts in a different phase from reactants, usually solids facilitating reactions between gases or liquids by adsorbing reactants to their surface.
- Homogeneous Catalysis: Catalysts are in the same phase as reactants, often in a liquid state, forming temporary intermediary compounds that decrease the energy needed for reactions.
Let's discuss heterogeneous catalysis as an example. Consider the Haber process for ammonia synthesis, where nitrogen and hydrogen gases react over an iron catalyst. The catalyst surface provides sites for the gases to adsorb and dissociate into reactive atoms. These atoms react faster than the molecules, significantly lowering the energy barrier. The effectiveness of heterogeneous catalysis can be mathematically represented by the Langmuir-Hinshelwood model, which considers the rates at which reactants adsorb, react, and desorb from the catalyst surface. Understanding these steps can help optimize catalyst efficiency, illustrating the profound impact of catalysis mechanisms on reaction dynamics.
The efficiency of a catalyst often depends on its surface area and ability to remain unaltered during the reaction.
Transition State Theory
The Transition State Theory (TST) provides a framework for understanding how chemical reactions progress through transient states with maximum energy. This theory explains how catalysts alter these transient states to make reactions more accessible. In TST, the transition state is conceptualized as a high-energy, short-lived configuration of atoms that exist during the conversion of reactants to products. The energy required to reach this state from the reactants is the activation energy. By lowering this energy, catalysts increase the reaction rate by enabling more molecules to reach and transition through this state.
The transition state is the configuration corresponding to the highest energy point along the reaction path. It is a fleeting state through which reactants must pass on their way to becoming products.
Suppose a reaction involves converting A to B via a transition state A*. Without a catalyst, the reaction follows this coordinate diagram:\[A \rightarrow A^* \rightarrow B\]With a catalyst, the energy of A* is reduced, effectively changing the reaction path to:\[A \rightarrow A^{**} \rightarrow B\]This demonstrates how the catalytic presence alters the transition state, reducing the energy barrier and enabling quicker conversion.
In TST, reactants are in equilibrium with the transition state, making it a powerful tool in calculating reaction rates.
Reaction Coordinate Diagrams
A reaction coordinate diagram is a graphical representation that illustrates the energy changes that occur during a chemical reaction. It helps visualize the transition states, intermediates, and energy barriers associated with the reaction.
Analyzing Enthalpy of Activation
The enthalpy of activation (ΔH‡) is the energy difference between the reactants and the transition state. It is a component of the activation energy that impacts the rate at which the reaction proceeds.
In a reaction coordinate diagram, the x-axis represents the reaction coordinate, which follows the progress of the reaction, while the y-axis represents the potential energy. The enthalpy of activation can be visualized as the vertical distance from the reactants' energy level to the peak of the transition state.Consider the Arrhenius equation, which relates to the rate constant \( k \) and incorporates the enthalpy of activation:\[ k = Ae^{-\frac{Ea}{RT}} \]Here, \( Ea \), the activation energy, includes both enthalpic (\( ΔH‡ \)) and entropic (\( ΔS‡ \)) contributions, represented through the Eyring equation:\[ k = \frac{k_B T}{h} e^{-\frac{ΔH‡}{RT} + \frac{ΔS‡}{R}} \]Understanding the enthalpy of activation is crucial in engineering reactions that are more efficient and require less energy.
Example: When a catalyst is introduced in a reaction of molecules \( X \) to \( Y \), the enthalpy of activation is reduced. Without a catalyst, the enthalpy might be 150 kJ/mol. With the catalyst, it is decreased to 100 kJ/mol. This reduction illustrates how catalysts lower the energy barrier, thereby increasing the reaction rate.
Reaction coordinate diagrams are also effective in understanding multistep reactions, where each step has its own activation energy. Such diagrams reveal intermediates and multiple transition states, highlighting how catalysts may selectively lower the energy of specific steps to accelerate the overall reaction. For example, in biological systems, enzymatic pathways are often highly selective, ensuring each step proceeds efficiently. In these pathways, the highest point of each step represents the transition state, and a well-designed catalyst can change this landscape significantly.
Visualizing Energy Barriers
Energy barriers are the peaks in reaction coordinate diagrams corresponding to transition states between reactants and products. By visualizing these barriers, you can better understand how catalysts affect chemical reactions. In general, lowering energy barriers is the key to increasing reaction rates.
An energy barrier is the energy required to transform reactants into an activated complex or transition state, represented by the highest point on a reaction coordinate diagram.
Example: In the conversion of \( A \) to \( C \) with an intermediate \( B \), the reaction coordinate diagram shows two energy barriers:\[ A \rightarrow B \rightarrow C \]The peaks represent the transition states \( A^* \) and \( B^* \). A catalyst can lower these peaks and thus the energy barriers, effectively facilitating the conversion from \( A \) to \( C \).
Catalysts do not change the overall energy difference between the reactants and products; they only alter the pathway and lower the activation energy.
Visualizing energy barriers can be particularly complex when dealing with reactions involving multiple reactants and products, or in complex systems such as organic synthesis. In these scenarios, reaction coordinate diagrams become multidimensional, showcasing various reaction pathways and their respective energy barriers. Computational chemistry and advanced modeling techniques help us pursue such visualizations, enabling detailed studies into where energy barriers lie and how they can be efficiently minimized through catalysis.
energy barriers in catalysis - Key takeaways
- Energy Barriers in Catalysis: Energy barriers, referred to as activation energy, determine the ease and speed of reactions; catalysts reduce these barriers, enhancing reaction rates.
- Activation Energy in Catalysis: Activation energy (Ea) is the minimum energy required for a reaction to occur, visualized as a peak in potential energy diagrams, reduced by catalysts.
- Catalysis Mechanisms: Catalysts lower activation energy through different kinds: enzymatic (biological), heterogeneous (different phase from reactants), and homogeneous (same phase).
- Catalytic Reaction Pathways: Catalysts offer alternative reaction routes with lowered activation energies, altering energy landscapes for more efficient reactions.
- Reaction Coordinate Diagrams: These illustrate energy changes during reactions, highlighting transition states and energy barriers that catalysts aim to reduce.
- Transition State Theory: Catalysts affect the transition state by lowering the energy required to reach it, thus increasing reaction rates.
Learn faster with the 12 flashcards about energy barriers in catalysis
Sign up for free to gain access to all our flashcards.
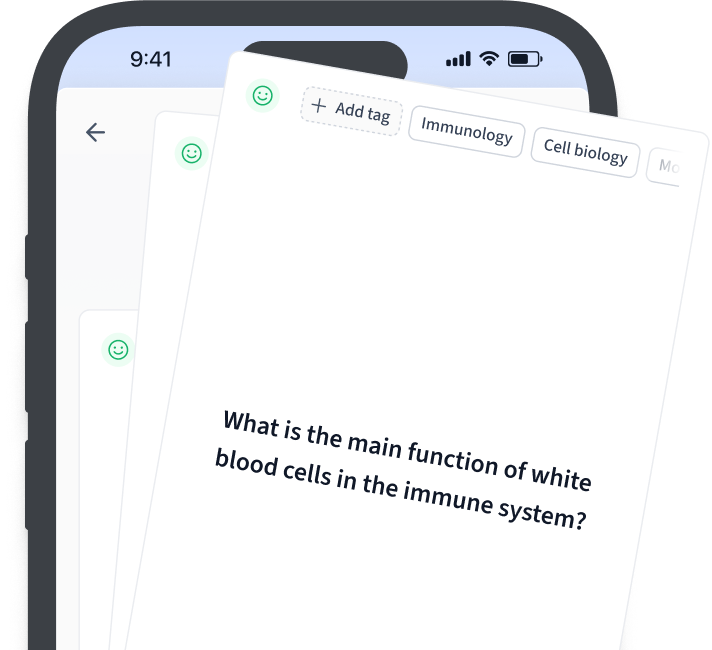
Frequently Asked Questions about energy barriers in catalysis
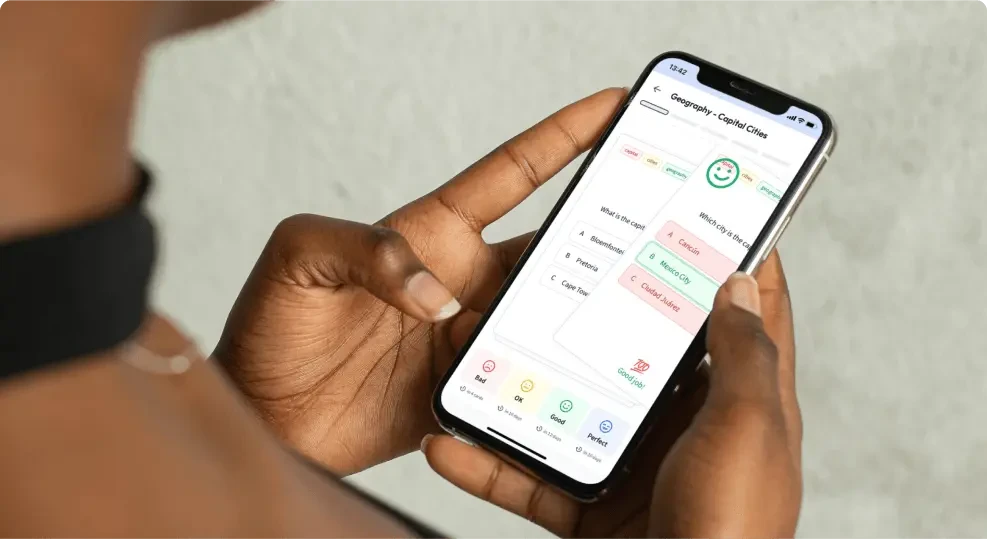
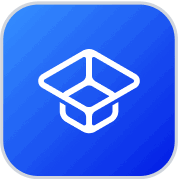
About StudySmarter
StudySmarter is a globally recognized educational technology company, offering a holistic learning platform designed for students of all ages and educational levels. Our platform provides learning support for a wide range of subjects, including STEM, Social Sciences, and Languages and also helps students to successfully master various tests and exams worldwide, such as GCSE, A Level, SAT, ACT, Abitur, and more. We offer an extensive library of learning materials, including interactive flashcards, comprehensive textbook solutions, and detailed explanations. The cutting-edge technology and tools we provide help students create their own learning materials. StudySmarter’s content is not only expert-verified but also regularly updated to ensure accuracy and relevance.
Learn more