Jump to a key chapter
Energy Conversion Processes Overview
Understanding energy conversion processes is essential in engineering. These processes play a key role in transforming energy from one form to another to facilitate various applications. This overview guides you through the fundamental concepts and mechanisms behind energy transformations.
Introduction to Energy Forms
Energy exists in several forms, each having distinct properties and applications. The primary forms of energy include:
- Mechanical Energy: Energy due to an object's motion or position. It includes both kinetic and potential energy.
- Thermal Energy: Comes from heat, which is the result of molecules moving rapidly and colliding.
- Chemical Energy: Stored in chemical bonds and released during a chemical reaction.
- Electrical Energy: Results from electric charges and their movement.
- Nuclear Energy: Released in nuclear reactions, either from fission or fusion processes.
Basic Energy Conversion Principles
Energy conversion is based on the law of conservation of energy, which states that energy cannot be created or destroyed; it can only change form. The general equation describing energy conversion is:
\[ E_{\text{in}} = E_{\text{out}} + E_{\text{loss}} \]
Where:
- \(E_{\text{in}}\) is the input energy.
- \(E_{\text{out}}\) is the output energy.
- \(E_{\text{loss}}\) accounts for energy lost in the conversion process, often in the form of heat.
Understanding Entropy: In energy conversions, entropy plays a crucial role. Entropy measures the level of disorder or randomness in a system. The second law of thermodynamics states that entropy in an isolated system will always increase over time, meaning that energy conversions are never 100% efficient. As energy transforms, some of it is inevitably lost as heat, increasing the system's disorder. This concept is crucial in designing efficient systems and understanding the limitations of energy conversion.
Real-world Applications of Energy Conversion
Energy conversion processes have numerous applications in everyday life. Consider the following examples:
- Combustion Engines: Convert chemical energy from fuel into mechanical energy, propelling vehicles.
- Solar Panels: Transform solar energy into electrical energy through photovoltaic cells.
- Hydroelectric Power Plants: Use mechanical energy from flowing water to generate electricity.
- Wind Turbines: Convert wind energy into electrical energy using a generator.
- Battery Storage: Store electrical energy as chemical energy and release it when needed.
Remember that the efficiency of an energy conversion process often determines its practicality and relevance in real-world applications.
Significance of Energy Conversion Efficiency
The efficiency of an energy conversion process is a measure of how well it converts input energy into useful output energy. Efficiency \(\eta\) can be mathematically defined as:
\[ \eta = \frac{E_{\text{out}}}{E_{\text{in}}} \times 100 \% \]
This formula helps evaluate the performance of devices and systems involved in energy conversion. For instance, electric motors may have efficiencies of around 90%, while internal combustion engines might only achieve 30-40% efficiency due to significant energy losses as heat.
Solar Energy Conversion Process Basics
Solar energy is a nearly endless source of energy that can be harnessed for various uses. Understanding the conversion processes that transform sunlight into usable energy forms is crucial for advancing renewable energy technologies.
Photovoltaic Energy Conversion
Photovoltaic (PV) systems are devices that convert sunlight directly into electricity. They are composed of solar cells, which are typically made from silicon. When sunlight hits these cells, it excites electrons, creating an electric current. Here's a basic explanation of the process:
- Sunlight strikes the solar cell, causing electrons to be knocked loose from their atoms.
- Conductors attached to the positive and negative sides of the cell form an electrical circuit.
- When electrons flow through the circuit, they generate electricity.
Advanced PV Materials: Recent advancements in PV technology involve new materials like perovskite solar cells. These materials have shown high efficiency rates and can be produced with less energy-intensive processes than traditional silicon, making them a promising option for future solar technologies. Perovskite cells can potentially achieve efficiencies over 25%, rivaling and even surpassing some traditional silicon cells.
Solar Thermal Energy Conversion
Solar thermal systems capture and utilize the sun's heat, converting it into thermal energy. This energy can be used for heating, generating steam to drive turbines, or producing hot water. There are a few key types of solar thermal systems:
- Flat-plate collectors are used for heating water and space heating.
- Concentrated solar power systems (CSP) use mirrors or lenses to focus a large area of sunlight onto a small area, producing high temperatures that can generate steam.
The conversion efficiency of CSP systems is determined by the formula:
\[ \eta = \frac{Q_{\text{out}}}{Q_{\text{in}}} \]
Where \(Q_{\text{out}}\) is the useful thermal energy output, and \(Q_{\text{in}}\) is the solar energy input.
Example Calculation: Consider a CSP system that receives 500 \(\text{kJ/m}^2\) of solar energy and produces 200 \(\text{kJ/m}^2\) of thermal energy. The efficiency \(\eta\) is calculated as:
\[ \eta = \frac{200}{500} \times 100\% = 40\% \]
This indicates that 40% of the incoming solar energy is converted into useful thermal energy.
Importance of Solar Energy Conversion
The conversion of solar energy into usable forms is vital for several reasons:
- It offers a sustainable alternative to fossil fuels and helps reduce carbon emissions.
- Solar energy systems have low operational costs once installed.
- They can be deployed in remote areas where traditional power grids are unavailable.
Remember, while solar energy systems have variable outputs depending on weather and time of day, advances in storage technologies are helping to mitigate these challenges.
Wind Energy Conversion Process Principles
Wind energy harnesses the power of nature by transforming the kinetic energy of wind into electrical energy. Understanding the principles behind this conversion process is essential as you explore renewable energy solutions and their engineering applications.
Components of Wind Energy Systems
Wind energy systems consist of various components that work together to capture and convert wind energy. These components include:
- Turbine Blades: Capture wind's kinetic energy and convert it into rotational energy.
- Rotor: The assembly of blades that rotates to capture energy.
- Nacelle: Houses the generator and other mechanical components.
- Generator: Converts mechanical energy into electrical energy.
Kinetic Energy: The energy an object possesses due to its motion, given by the formula:
\[ KE = \frac{1}{2}mv^2 \]
Where \(m\) is the mass and \(v\) is the velocity of the object.
The efficiency of wind energy systems depends on both the wind speed and the design of the turbine blades.
Basic Mechanics of Wind Energy Conversion
The basic mechanics of converting wind energy involve capturing the wind's kinetic energy and transforming it into mechanical energy before converting it into electricity. This process can be summarized as follows:
- Wind spins the turbine blades, creating rotational energy.
- The rotor transfers this rotational energy to the generator.
- The generator converts rotational energy into electrical energy.
\[ P = \frac{1}{2} \rho A v^3 \]
Where:
- \(P\) is the power output.
- \(\rho\) is the air density.
- \(A\) is the area swept by the turbine blades.
- \(v\) is the wind velocity.
Example Calculation: For a wind turbine with a blade swept area of 50 \(\text{m}^2\), an air density of 1.225 \(\text{kg/m}^3\), and a wind speed of 10 \(\text{m/s}\), calculate the power output:
\[ P = \frac{1}{2} \times 1.225 \times 50 \times (10)^3 = 30625 \text{ Watts} \]
Efficiency of Wind Energy Conversion
The efficiency of wind energy conversion depends on various factors, including turbine design and environmental conditions. The efficiency, known as the power coefficient \(Cp\), is calculated using:
\[ Cp = \frac{P_{out}}{P_{in}} \]
Where:
- \(P_{out}\) is the actual power output of the turbine.
- \(P_{in}\) is the power available from the wind.
Innovative Turbine Designs: Recent advances in turbine technology have led to unique designs like vertical-axis wind turbines and bladeless turbines. These innovative designs aim to optimize efficiency, reduce noise levels, and increase suitability for urban environments. Vertical-axis turbines can capture wind from any direction, and bladeless turbines use oscillation to generate energy, showing promise for future developments in wind energy technology.
Nuclear Energy Conversion Process Explained
The conversion of nuclear energy into usable forms is a critical aspect of modern energy production. This process involves the transformation of nuclear reactions into thermal energy, which can then be converted into electricity.
Thermodynamics and Energy Conversion in Nuclear Systems
Nuclear reactions such as fission and fusion release energy that can be harnessed to generate electricity. In nuclear power plants, thermodynamics plays a significant role in this energy conversion process.
Nuclear fission involves splitting heavy nuclei like uranium to release energy. The energy from fission heats water, producing steam that drives turbines connected to generators.
The general energy conversion cycle in a nuclear power plant includes:
- Fission reaction heats water in the reactor core.
- High-pressure steam is produced.
- Steam drives turbines to generate electricity.
- Steam is condensed back into water and recirculated.
\[ \eta = 1 - \frac{T_{\text{cold}}}{T_{\text{hot}}} \]
where \(T_{\text{cold}}\) and \(T_{\text{hot}}\) are the absolute temperatures of the cold and hot reservoirs, respectively.
Nuclear Fission: A process where the nucleus of an atom splits into smaller parts, releasing a large amount of energy.
Example Calculation: Consider a reactor operating between a hot reservoir at 600K and a cold reservoir at 300K. The maximum efficiency can be calculated as:
\[ \eta = 1 - \frac{300}{600} = 0.5 \text{ or } 50\% \]
In practice, actual efficiencies are lower than the theoretical maximum due to energy losses and engineering limitations.
Future of Nuclear Fusion: Unlike fission, nuclear fusion involves combining light nuclei, such as hydrogen, to form a heavier nucleus. Fusion has the potential to offer a nearly limitless and cleaner source of energy. However, achieving and maintaining the conditions necessary for fusion — extreme temperatures and pressures — is a significant scientific and engineering challenge. Researchers are working on experimental reactors like the ITER project, aiming to demonstrate the viability of fusion as a sustainable energy source.
Geothermal Energy Conversion Process Insights
Geothermal energy capitalizes on the Earth's internal heat to generate electricity and provide direct heating. This renewable energy source is abundant and has great potential for sustainable energy production.
Thermodynamics and Energy Conversion in Geothermal Applications
Thermodynamics plays a pivotal role in harnessing geothermal energy. At the core of many geothermal energy systems is the process of converting heat energy from the Earth's crust into mechanical and then electrical energy.
In geothermal power plants, heat from deep underground is used to heat water and produce steam. This steam is then utilized to drive turbines, which in turn activate generators to produce electricity.
The efficiency of geothermal systems can be evaluated using the formula for Carnot efficiency, given by:
\[ \eta = 1 - \frac{T_{\text{cool}}}{T_{\text{hot}}} \]
Here, \(T_{\text{cool}}\) and \(T_{\text{hot}}\) represent the absolute temperatures of the heat sink and source, respectively.
Geothermal Energy: Energy derived from the heat stored within the Earth.
Example of Geothermal Energy Use: A binary cycle power plant operates with a hot reservoir temperature of 150°C (423.15K) and a cold sink temperature of 30°C (303.15K). The theoretical Carnot efficiency is:
\[ \eta = 1 - \frac{303.15}{423.15} \approx 28.36\% \]
Geothermal power plants often have lower temperature differentials compared to conventional power plants, impacting their overall efficiency.
Advanced Geothermal Technologies: Enhanced Geothermal Systems (EGS) represent a cutting-edge approach to geothermal energy. EGS involves creating reservoirs in areas with hot rocks but lacking water. By fracturing these rocks and introducing water, EGS aims to significantly expand geothermal power capacity. This technology could tap into vast heat reserves, increasing the geographical feasibility and output of geothermal energy systems.
Biomass Energy Conversion Process Fundamentals
Biomass energy conversion involves transforming energy stored in biological materials into heat, electricity, or biofuels. This process is an integral part of renewable energy systems, contributing to sustainable energy goals by utilizing organic matter.
Thermodynamics and Energy Conversion in Biomass
The conversion of biomass into usable energy often involves thermal processes governed by the principles of thermodynamics. Biomass can be directly converted into thermal energy through combustion, or processed into biofuels using biochemical methods.
In thermochemical conversion, biomass undergoes combustion, pyrolysis, or gasification:
- Combustion: Direct burning of biomass to produce heat and power.
- Pyrolysis: Biomass is heated in the absence of oxygen, producing bio-oil, syngas, and biochar.
- Gasification: Biomass is converted to syngas (a mixture of hydrogen and carbon monoxide) through partial oxidation.
\[ \eta = \frac{Q_{\text{out}}}{Q_{\text{in}}} \]
Where \(Q_{\text{out}}\) is the useful energy output and \(Q_{\text{in}}\) is the energy input from biomass.
Biomass: Organic material derived from plants and animals used as a renewable energy source.
Example Calculation: If a biomass gasification process inputs 1000 kJ of energy and outputs 350 kJ of useful energy, the efficiency is:
\[ \eta = \frac{350}{1000} \times 100\% = 35\% \]
Advancements in Biochemical Conversion: Biochemical processes like fermentation and anaerobic digestion convert biomass into biofuels. Leveraging enzymes and microorganisms, these methods produce biofuels such as ethanol and biogas efficiently. Innovative research in genetic engineering aims to enhance microbial efficiency and increase biofuel yields, addressing biomass feedstock variability and environmental sustainability.
Biomass energy not only reduces waste but also provides a carbon-neutral energy option when resources are managed sustainably.
energy conversion processes - Key takeaways
- Energy conversion processes involve transforming energy from one form to another and are essential in applications such as solar, wind, nuclear, geothermal, and biomass energy.
- Solar energy conversion process: Utilizes photovoltaic cells to convert sunlight into electricity and includes solar thermal systems for heat conversion.
- Wind energy conversion process: Involves wind turbines converting wind’s kinetic energy into electrical energy, with efficiency influenced by turbine design and environmental conditions.
- Nuclear energy conversion process: Converts nuclear reactions like fission into thermal energy, which is then transformed into electricity in power plants, heavily relying on thermodynamics.
- Geothermal energy conversion process: Harnesses the Earth's internal heat for electricity and heating, with efficiency assessed through the Carnot efficiency formula.
- Biomass energy conversion process: Involves converting biological materials into heat, electricity, or biofuels using thermochemical methods like combustion, pyrolysis, and gasification.
Learn faster with the 12 flashcards about energy conversion processes
Sign up for free to gain access to all our flashcards.
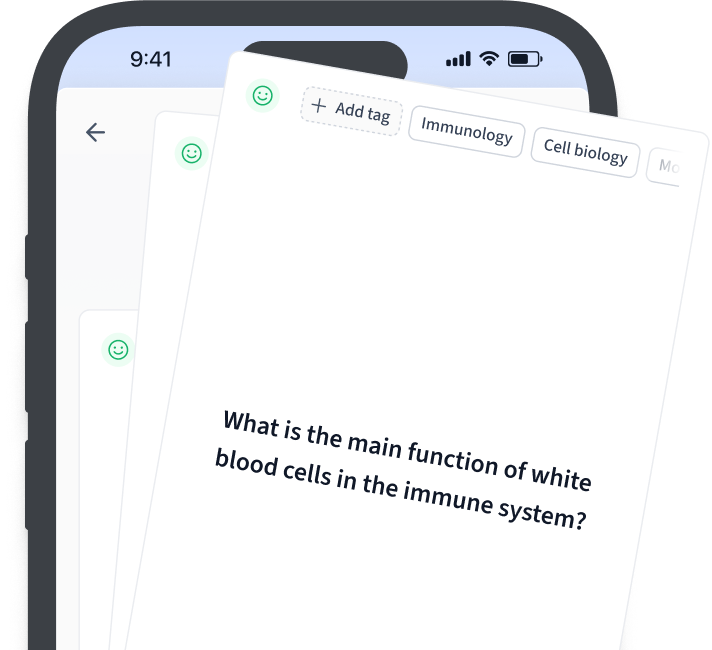
Frequently Asked Questions about energy conversion processes
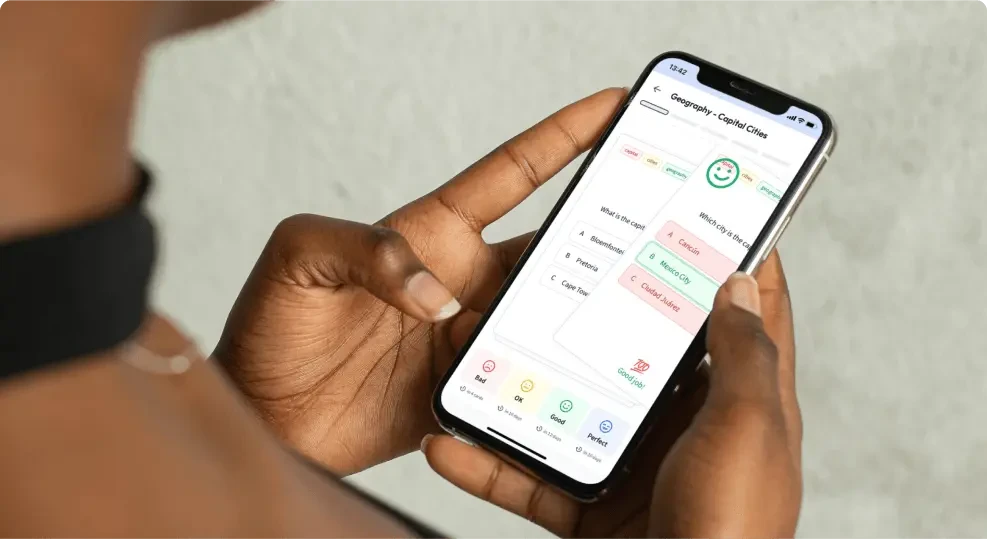
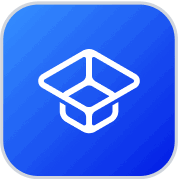
About StudySmarter
StudySmarter is a globally recognized educational technology company, offering a holistic learning platform designed for students of all ages and educational levels. Our platform provides learning support for a wide range of subjects, including STEM, Social Sciences, and Languages and also helps students to successfully master various tests and exams worldwide, such as GCSE, A Level, SAT, ACT, Abitur, and more. We offer an extensive library of learning materials, including interactive flashcards, comprehensive textbook solutions, and detailed explanations. The cutting-edge technology and tools we provide help students create their own learning materials. StudySmarter’s content is not only expert-verified but also regularly updated to ensure accuracy and relevance.
Learn more