Jump to a key chapter
Definition of Fermentation Processes in Engineering
Fermentation processes are vital in engineering, especially within the fields of biotechnology and chemical engineering. These processes involve the conversion of sugars and other carbohydrates into essential products like acids, gases, or alcohol through the action of microorganisms such as yeast or bacteria. Fermentation is widely applied not only in the food and beverage industry but also in pharmaceuticals, waste treatment, and biofuel production.
Key Concepts in Fermentation Processes
The study of fermentation involves several key concepts that are essential for understanding how these processes are utilized in engineering. Here are the primary concepts:
- Substrate: The material or substance on which an enzyme acts. In fermentation, common substrates include sugars such as glucose and lactose.
- Microorganism: These are the living entities such as yeast, molds, and bacteria that carry out fermentation.
- Enzymes: Biological catalysts that accelerate the fermentation reactions.
- Anaerobic Conditions: Most fermentation processes occur in the absence of oxygen, although some can proceed aerobically.
- Bioproducts: The output of fermentation, which may include ethanol, lactic acid, carbon dioxide, and other valuable compounds.
Mathematically, the fermentation process can be expressed in terms of balanced chemical equations. For example, the production of ethanol from glucose by yeast is represented as:
\[ C_6H_{12}O_6 \rightarrow 2C_2H_5OH + 2CO_2 \]
Each of these concepts underpins the efficiency and functionality of fermentation systems, with significant attention given to process optimization and product yield.
Importance of Fermentation in Chemical Engineering
Fermentation processes play a crucial role in chemical engineering due to their versatile applications. Here are some of the reasons:
- Sustainable Production: Fermentation offers a renewable method to produce chemicals and fuels, significantly reducing reliance on fossil fuels.
- Scalability: These processes can be scaled up from laboratory to industrial levels, optimizing production capacity and efficiency.
- Diverse Applications: Fermentation is used in producing pharmaceuticals like antibiotics, organic acids, vitamins, and biofuels.
- Waste Reduction: It contributes to waste treatment by converting organic waste into useful products, minimizing environmental impact.
An important aspect of its significance is the conversion efficiency, which can be quantified through yield and productivity calculations such as:
\[ Y_{Product/Substrate} = \frac{Product\text{ } formed}{Substrate\text{ } consumed} \]
This equation helps researchers and engineers determine the effectiveness of a fermentation process, leading to informed decisions about process improvements.
Fermentation can be both aerobic and anaerobic, but most industrial processes prefer anaerobic conditions due to their cost-effectiveness.
Anaerobic Fermentation Process Overview
Anaerobic fermentation is a type of fermentation process where microorganisms convert substrates like sugars into products such as alcohols, acids, and gases in the absence of oxygen. This process is crucial in industries like pharmaceuticals, biofuels, and waste treatment due to its versatility and efficiency.
Core Steps of Anaerobic Fermentation
Anaerobic fermentation consists of several key steps that transform substrates into desired products. Understanding these steps is essential for optimizing production and efficiency in industrial applications:
- Substrate Preparation: Breaking down complex carbohydrates into simpler sugars.
- Initiation: Introduction of microorganisms to the substrate.
- Metabolic Conversion: Microorganisms metabolize the substrate to generate energy, resulting in by-products such as ethanol or lactic acid.
- Growth Phase: Biomass growth phase where microorganisms reproduce, increasing the fermentation rate.
- Harvesting: Separation and collection of the end products from the biomass.
Each step in the process can be modeled mathematically to ensure optimal conditions are maintained. For instance, the metabolic conversion can be represented as:
\[ C_6H_{12}O_6 + 2ADP + 2P_i \rightarrow 2C_2H_5OH + 2CO_2 + 2ATP \]
In anaerobic fermentation, the energy yield per glucose molecule is often lower than in aerobic processes, but it remains a critical pathway for producing commodities like ethanol. Consider the energy balance:
\[ \Delta G = - 239 \text{ kJ/mol} \] This negative Gibbs free energy indicates the process is spontaneous, albeit lower in energy efficiency compared to aerobic pathways. The limited ATP production per glucose also reflects this disparity.
Conditions Required for Anaerobic Fermentation
Several conditions are critical for effective anaerobic fermentation, ensuring that microorganisms thrive and the desired products are efficiently produced.
- Anaerobic Environment: The absence of oxygen is crucial as it affects the metabolic pathways and efficiency of product formation.
- Temperature: The optimal range is specific to the type of microorganism, often between 30°C to 40°C.
- pH Level: Most anaerobic fermentations work best at a pH range of 5.0 to 6.5.
- Nutrient Availability: Adequate nutrients are necessary to support microbial growth and metabolism.
- Inoculum Concentration: A higher concentration can speed up the fermentation process, enhancing product yield.
These parameters can be adjusted to optimize the anaerobic fermentation process, using mathematical modeling to predict the impact of various conditions on fermentation outcomes:
\[ \mu = \mu_{max} \left( \frac{S}{K_s + S} \right) \]
Here, \( \mu \) represents the microbial growth rate, demonstrating how it depends on substrate concentration \( S \) and the half-saturation constant \( K_s \).
Keeping strict control of environmental conditions can significantly increase the efficiency and yield of anaerobic fermentation processes.
Lactic Acid Fermentation Process Explained
Lactic acid fermentation is a biological process where sugars such as glucose, fructose, and sucrose are converted into cellular energy and the metabolic byproduct lactate. This process occurs in bacteria and some animal cells like muscle fibers. It's anaerobic, meaning it doesn't require oxygen, making it vital for certain organisms and industries.
In lactic acid fermentation, one molecule of glucose is typically converted into two molecules of lactic acid in the absence of oxygen. This can be represented by the equation:
\[ C_6H_{12}O_6 \rightarrow 2C_3H_6O_3 \]
Understanding the steps of this process is crucial for applications in food technology and medicine.
Applications of Lactic Acid Fermentation
Lactic acid fermentation has a wide range of applications, especially in food production and preservation:
- Food Industry: This process is used to produce fermented dairy products like yogurt, cheese, and buttermilk, as well as other fermented foods such as sauerkraut, pickles, and kimchi.
- Preservation: Lactic acid acts as a natural preservative by lowering the pH and inhibiting spoilage microorganisms, effectively extending the shelf life of perishable foods.
- Pharmaceuticals: It is involved in the production of probiotics and certain vaccines.
- Bioplastics: Lactic acid is a monomer used in the synthesis of polylactic acid (PLA), a biodegradable plastic.
Each application benefits from the unique properties of lactic acid, particularly its ability to stabilize or improve the product through fermentation. For example, in cheese making, lactic acid fermentation helps in developing flavor and texture.
An example of lactic acid fermentation in action is the traditional pickling process of cucumbers. Cucumbers are submerged in a brine solution, and Lactobacillus bacteria convert sugars present in the cucumbers into lactic acid, which acts to preserve the pickles and enhance flavor.
In traditional Korean kimchi fermentation, a variety of vegetables are mixed with seasonings and lactic acid bacteria naturally present on the leaves. The microbes produce lactic acid, acetic acid, and carbon dioxide over weeks, contributing to the unique sour taste and improved nutritional profile due to increased levels of vitamins and minerals.
Differences Between Lactic Acid and Other Fermentation Processes
While lactic acid fermentation is defined by the conversion of glucose into lactic acid, other types of fermentation processes have distinct pathways and products:
- Alcoholic Fermentation: Performed by yeasts, this process transforms glucose into ethanol and carbon dioxide, primarily used in brewing and winemaking.
- Acetic Acid Fermentation: Involves the conversion of ethanol into acetic acid by bacteria such as Acetobacter, playing a role in vinegar production.
- Butyric Acid Fermentation: In some bacteria, glucose is converted into butyric acid, carbon dioxide, and hydrogen, often associated with rancid butter and cheese flavors.
- Propionic Acid Fermentation: Generates propionic acid and carbon dioxide, contributing to the flavor and eye-hole formation in Swiss cheese.
These fermentation processes differ not only in their end products but in their biological roles and industrial applications. For instance, while lactic acid fermentation is significant in dairy, alcoholic fermentation is indispensable in beverage production.
Lactic acid fermentation is unique in that it does not produce gas as a byproduct, unlike alcoholic fermentation, which produces carbon dioxide.
Fermentation Engineering Techniques
Fermentation engineering involves a variety of technologies and practices to convert organic compounds into valuable products using microorganisms. As advancements continue to reshape this field, modern techniques play a crucial role in enhancing efficiency and scalability.
Modern Techniques in Fermentation Engineering
Modern fermentation engineering incorporates advanced methods and technologies to optimize production processes. Here are some key techniques:
- Continuous Fermentation: Unlike batch fermentation, continuous systems allow for sustained input and output of substrates and products, leading to higher efficiency.
- Immobilized Cell Systems: Microorganisms are fixed onto a surface, facilitating repeated use and stability.
- Bioreactor Design: Modern bioreactors feature precise control over environmental conditions, enabling optimized growth and product formation.
- Genetic Engineering: This involves modifying organisms to enhance yield, efficiency, or substrate range.
- Process Control and Automation: Use of sensors and automation software to monitor variables like temperature, pH, and oxygen levels for better process outcomes.
Each technique addresses specific challenges in traditional fermentation, paving the way for increased industrial scalability and product consistency.
One fascinating development in fermentation engineering is the use of genetically modified microorganisms. For instance, scientists have engineered yeast strains to produce higher levels of desired products like ethanol or insulin precursors. This not only increases yield but can reduce the environmental footprint, as these strains may utilize alternative non-food substrates like agricultural residues.
Table: Example of Genetic Modifications in Fermentation
Modification | Outcome |
CRISPR-Cas9 in Yeast | Increased Ethanol Production |
Gene Knockout in E. coli | Enhanced Antibiotic Production |
Overexpression of Lactase Gene | Higher Lactose Metabolism |
Challenges in Fermentation Engineering
Despite advancements, fermentation engineering faces several challenges that must be addressed:
- Substrate Costs: The availability and cost of substrates can significantly impact the economics of fermentation processes.
- Contamination Risk: Sterile conditions are hard to maintain, and contamination can lead to loss of entire batches.
- Product Purification: The separation and purification of produced compounds are often expensive and complex.
- Genetic Stability: Genetically modified strains might lose modified traits over generations, affecting consistency.
- Scalability: Transitioning from lab-scale to industrial-scale processes poses significant technical and economic challenges.
Overcoming these challenges often requires interdisciplinary approaches, combining fields like material science, bioinformatics, and systems engineering.
Improving downstream processing can reduce overall production costs significantly, making the entire fermentation process more viable economically.
Fermentation Process Ethanol Production
Ethanol production through fermentation is one of the most established bioprocesses, characterized by the conversion of sugars into ethanol and carbon dioxide by yeast. This process is widely used for manufacturing biofuels, alcoholic beverages, and in other industrial applications.
Mathematically, the overall reaction for ethanol fermentation from glucose can be expressed as:
\[ C_6H_{12}O_6 \rightarrow 2C_2H_5OH + 2CO_2 \]
Steps in Ethanol Fermentation Process
The ethanol fermentation process consists of various steps that are crucial for efficient production:
- Substrate Preparation: Carbohydrate-rich biomass is prepared and hydrolyzed into fermentable sugars.
- Inoculation: Yeast or bacteria are introduced to the substrate.
- Fermentation: An anaerobic process where yeast converts sugars into ethanol and carbon dioxide.
- Distillation: The product is distilled to concentrate ethanol.
- Dehydration: Removing water content to obtain high-purity ethanol.
During the fermentation process, the microorganisms metabolize substrates according to the reaction:
\[ \text{C}_6\text{H}_{12}\text{O}_6 + \text{ATP} \rightarrow \text{2 CH}_3\text{CH}_2\text{OH} + \text{2 CO}_2 + \text{ATP} \]
Considerations in the fermentation process include temperature, pH, and substrate concentration. These factors influence the activity of Saccharomyces cerevisiae, the yeast commonly used. Optimal conditions often involve temperatures between 25°C to 30°C and a pH around 4.5 to 5.0. Understanding the enzyme kinetics, such as Michaelis-Menten, further directs process optimization:
\[ v = \frac{V_{max} [S]}{K_m + [S]} \]
This equation illustrates how the reaction velocity \( v \) depends on substrate concentration \([S]\), with \( V_{max} \) representing maximum rate and \( K_m \) the Michaelis constant.In a practical example, the production of ethanol from corn starch involves initial enzymatic hydrolysis to sugars, followed by fermentation in large vats where yeast is used to convert sugars into alcohol. This alcohol is then distilled to yield fuel ethanol.
Switching substrates from food products to non-food plants reduces competition for food resources and supports sustainable ethanol production.
Industrial Applications of Ethanol Fermentation
Fermentation-derived ethanol is utilized in numerous industries beyond fuel production:
- Alcoholic Beverages: Wine, beer, and spirits primarily rely on ethanol from fermentation.
- Chemicals Industry: Ethanol serves as a precursor for various solvents and chemical syntheses.
- Pharmaceuticals: Utilized as a disinfectant and in the manufacture of certain medications.
- Food Preservation: Ethanol is used in preserving and enhancing taste.
The economic viability of ethanol production stems from its diverse applications and demand as a sustainable energy source. It forms an integral part of the global push towards renewable energy solutions.
Advancements in genetic engineering have increased ethanol yields by creating more robust yeast strains capable of higher ethanol tolerance.
Examples of Fermentation Processes in Engineering
Fermentation processes are pivotal in various engineering domains, transforming raw materials into valuable end products. These processes are extensively applied in different sectors ranging from pharmaceuticals to bioenergy and food production. Here, you'll explore detailed examples that showcase the versatility and innovation across engineering disciplines.
Case Studies in Fermentation Engineering
Through different case studies, you can see how fermentation processes are implemented in real-world applications, highlighting their efficiency and scalability.
Industrial Biofuel Production: Utilizing lignocellulosic biomass, such as corn stover, for the production of bioethanol through fermentation is an excellent environmental alternative to fossil fuels. Saccharomyces cerevisiae is engineered to improve ethanol yield, demonstrating the integration of genetic engineering in fermentation technology.
Here's a simplified model of the bioethanol production reaction:
\[ C_6H_{12}O_6 \rightarrow 2C_2H_5OH + 2CO_2 \]
Pharmaceutical Antibiotic Production: The fermentation of Streptomyces bacteria to produce antibiotics such as penicillin is an enduring example. This process involves precise control of environmental conditions in bioreactors to maximize yield.
Aspect | Example |
Microorganism | Streptomyces chrysogenum |
Product | Penicillin |
Substrate | Lactose, Corn Steep Liquor |
Exploring the evolution of fermentation in industrial settings reveals the advancement from batch to continuous production systems. Batch fermentation is characterized by its endpoint nature, leading to downtime between cycles. However, continuous fermentation maintains a steady state, allowing for higher productivity. Engineers monitor variables such as microbial growth rate \( \mu \) using the formula:
\[ \mu = \frac{dX}{dt} = \frac{1}{X} \frac{dX}{dt} \]
This approach helps improve the efficiency and predictability of fermentation processes, ensuring high throughput and sustainability.
Continuous fermentation reduces downtime but requires precise control of conditions to prevent contamination.
Innovative Fermentation Process Applications
Innovation in fermentation processes has opened new possibilities across various industries. These breakthroughs enhance not only production capacities but also environmental sustainability.
Bioplastic from Lactic Acid: Fermenting glucose into lactic acid, further polymerized to produce polylactic acid (PLA), contributes to biodegradable plastic production. Enhanced microbial strains increase productivity, showcasing sustainability in product lifecycle management.
Microbial Fuel Cells: Utilizes bacteria to convert organic substrates into electricity, demonstrating the potential of fermentation in generating renewable energy.
- Key System Input: Organic matter like glucose or waste
- Output: Electrical current
These innovative applications underline the ability of fermentation processes to adapt and meet the demands of modern industry challenges.
An exemplary application in food technology is the use of fermentation to produce trendy fermented beverages like kombucha, where tea and sugar are fermented by a symbiotic colony of bacteria and yeast (SCOBY). This method not only results in a probiotic-rich drink but highlights fermentation's versatility in creating health-oriented products.
fermentation processes - Key takeaways
- Fermentation Processes Definition: Conversion of sugars into products like acids, gases, or alcohol using microorganisms, essential in biotechnology and chemical engineering.
- Anaerobic Fermentation Process: Without oxygen, microorganisms convert substrates to produce alcohols, acids, and gases, used in industries like pharmaceuticals and biofuels.
- Lactic Acid Fermentation Process: Sugars are converted into lactic acid without oxygen, applied in food technology and pharmaceuticals.
- Fermentation Engineering Techniques: Includes continuous fermentation, immobilized cell systems, and genetic engineering to enhance efficiency and scalability.
- Fermentation Process Ethanol: Conversion of sugars to ethanol and carbon dioxide by yeast, used in biofuel and beverage production.
- Examples in Engineering: Includes biofuel production from biomass, antibiotic production via bacteria fermentation, and bioplastic from glucose fermentation.
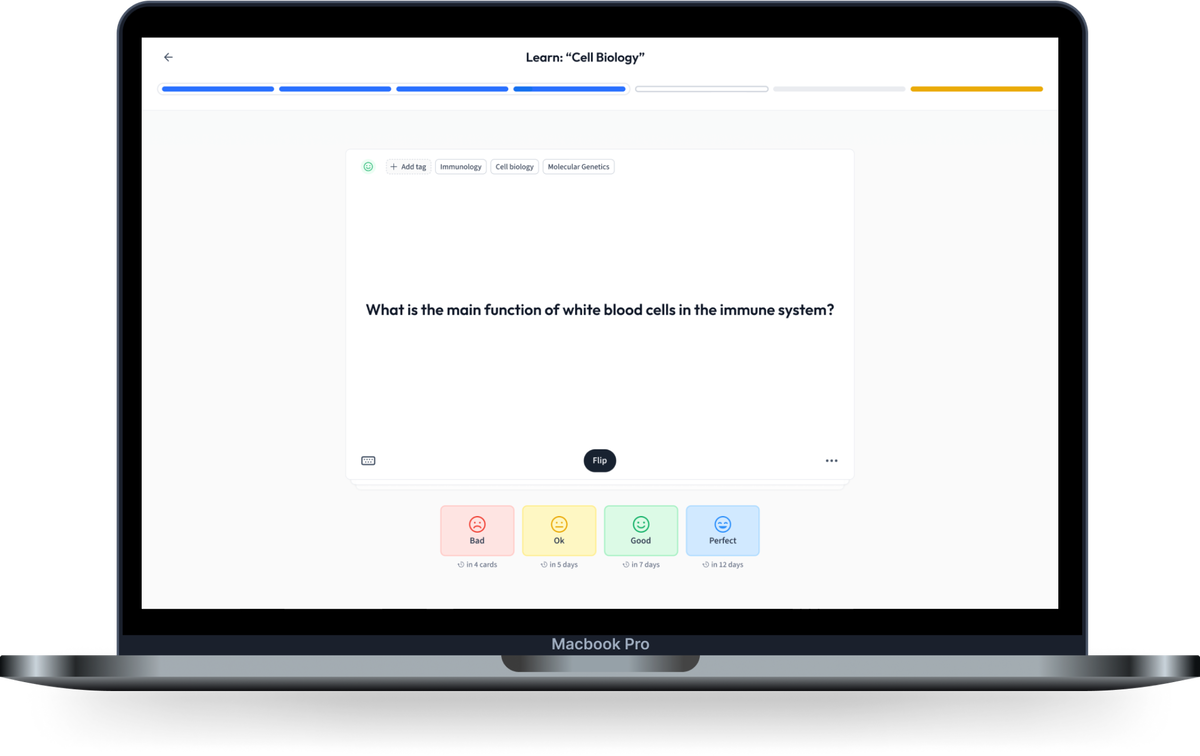
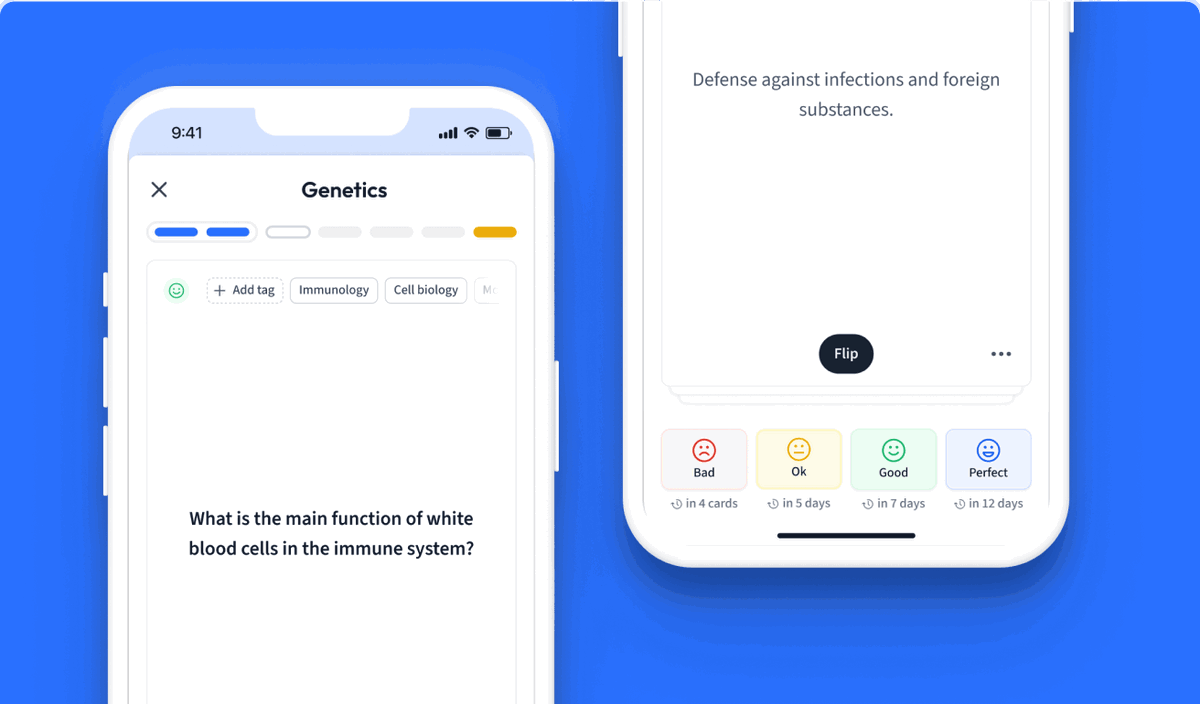
Learn with 12 fermentation processes flashcards in the free StudySmarter app
Already have an account? Log in
Frequently Asked Questions about fermentation processes
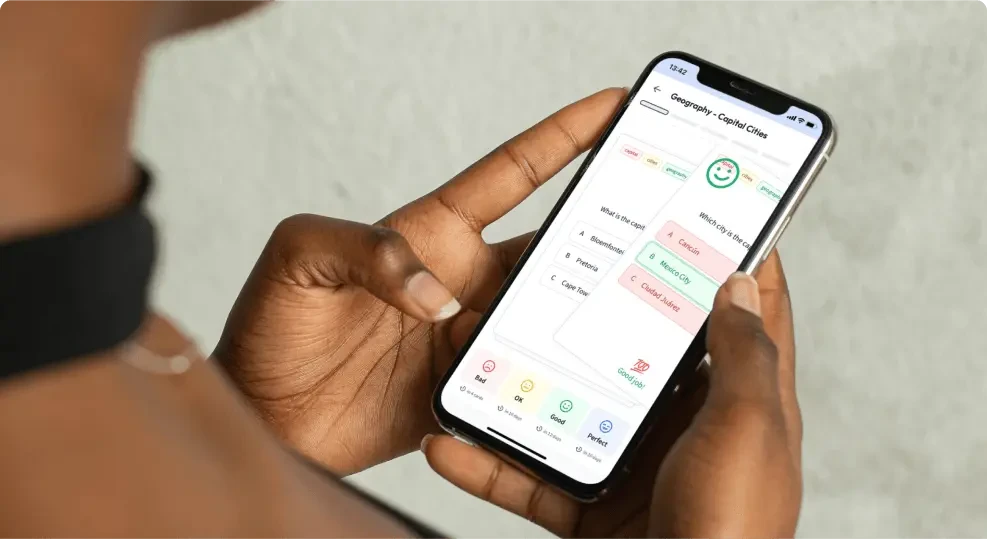
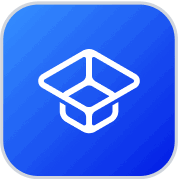
About StudySmarter
StudySmarter is a globally recognized educational technology company, offering a holistic learning platform designed for students of all ages and educational levels. Our platform provides learning support for a wide range of subjects, including STEM, Social Sciences, and Languages and also helps students to successfully master various tests and exams worldwide, such as GCSE, A Level, SAT, ACT, Abitur, and more. We offer an extensive library of learning materials, including interactive flashcards, comprehensive textbook solutions, and detailed explanations. The cutting-edge technology and tools we provide help students create their own learning materials. StudySmarter’s content is not only expert-verified but also regularly updated to ensure accuracy and relevance.
Learn more