Jump to a key chapter
Definition of Glass Transition
Glass transition is a fascinating and critical concept in material science, especially in the study of polymers and glasses. It refers to the reversible transition in amorphous materials from a hard and relatively brittle state into a molten or rubber-like state. Understanding this process is essential for applications in material engineering.
Glass Transition Explained
During the glass transition, materials do not move instantaneously from one rigid state to another; instead, they exhibit a gradual relaxation of molecular chains.Key aspects of glass transition include:
- It is a second-order transition, meaning that it affects the derivative properties of materials such as specific heat capacity, rather than heat transfer processes.
- It affects amorphous regions in polymeric materials and is distinct from the melting point, which is a first-order transition observed in crystalline regions.
- Chemical structure
- Polymer morphology
- Cooling rate
The glass transition temperature can be modified by incorporating plasticizers, which lower T_g and make materials more flexible at lower temperatures.
At a molecular level, the glass transition occurs when there is enough thermal energy to allow substantial molecular motion, enabling the chains in polymers to assume a random coil configuration. Mathematically, the T_g can be estimated using the Fox equation for copolymers: \[\frac{1}{T_g} = \frac{w_1}{T_{g1}} + \frac{w_2}{T_{g2}}\] where w_1 and w_2 are the weight fractions of components, and T_{g1} and T_{g2} are their respective glass transition temperatures. In practical scenarios, factors such as humidity and pressure can also affect T_g, which requires consideration of these variables in quality control and design processes.This deeper understanding of the glass transition at the molecular level allows engineers and scientists to tailor materials for specific applications and predict how they will behave under different environmental conditions.
Understanding Glass Transition Phase
Understanding the glass transition phase involves examining the changes that occur in specific material properties. The glass transition phase is observed in amorphous solids like polymers, where it signifies the transformation from a glassy to a rubbery state.Characteristics of the glass transition phase include:
- Viscosity: In the glassy state, materials have extreme viscosity, while in the rubbery state, the viscosity decreases significantly.
- Molecular Mobility: The transition allows increased molecular mobility, resulting in a more flexible material.
For a real-world application, consider common packaging materials like polystyrene. Polystyrene has a T_g around 100°C, meaning it retains rigidity under room conditions but becomes more pliable at higher temperatures. This characteristic explains its widespread use in packaging and insulation, where temperature variability can be controlled.
Glass Transition Temperature
The glass transition temperature (T_g) represents a crucial thermal property for amorphous materials, signifying the transformation from a rigid, glassy state to a more flexible, rubbery state.
Factors Affecting Glass Transition Temperature
Several factors influence the glass transition temperature of a material, impacting its practical applications and performance.
- Chemical Structure: The presence of side groups, chain flexibility, and bond strength in polymers significantly affect T_g. For example, stiff chain structures generally lead to a higher T_g.
- Molecular Weight: As molecular weight increases, T_g also rises due to increased chain entanglement and reduced mobility.
- Crosslinking: Introducing crosslinks in a polymer network enhances stiffness and raises T_g, as the network constrains molecular motion.
- Plasticizers: By adding plasticizers, T_g can be reduced, allowing the material to be more flexible at lower temperatures.
- Cooling Rate: A faster cooling rate might lead to a higher observed T_g due to insufficient time for the polymers to reorganize.
Understand that changing the environmental conditions such as humidity and pressure can influence the observed T_g.
Consider polystyrene, a widely used polymer. Its T_g is around 100°C. By adding plasticizers, this T_g can be tailored for applications that demand flexibility at lower temperatures, such as household insulation materials.
Measuring Glass Transition Temperature
The measurement of glass transition temperature is performed using various techniques, each offering unique benefits and precision.
- Differential Scanning Calorimetry (DSC): This technique measures heat flow into and out of a sample as it is heated or cooled, detecting changes that correspond to the glass transition.
- Dynamic Mechanical Analysis (DMA): DMA evaluates mechanical properties by applying periodic stress and measuring the material's response, identifying T_g through changes in viscoelastic properties.
- Thermomechanical Analysis (TMA): TMA measures dimensional changes over a temperature range, indicating T_g by observing expansion behavior.
- w_1 and w_2 are the weight fractions of monomeric units,
- T_{g1} and T_{g2} are their respective glass transition temperatures.
Understanding the accuracy of glass transition measurements involves considering several parameters. Differential Scanning Calorimetry (DSC) often requires careful calibration and baseline adjustments to ensure reliable data. This method is favored for its analytical precision in determining T_g and other thermal transitions within a sample. In comparison, Dynamic Mechanical Analysis (DMA) provides insights into mechanical behavior alongside thermal properties, offering a more comprehensive picture of the material's performance in real-world scenarios.For practical use, the choice of measurement technique depends on the properties of interest and the specific application requirements. Where understanding mechanical performance is critical, such as with structural polymers, DMA might be preferred over DSC. Moreover, understanding different T_g implications can help engineers develop materials that remain effective under varying service temperatures.
Glass Transition in Polymers
In the world of material science, the glass transition is an essential concept, especially when discussing polymers. It determines when a polymer switches from being brittle and glass-like to becoming more rubber-like and pliable. Understanding this transition helps in predicting and optimizing the behavior of polymers in various applications.
Role of Glass Transition Phase in Polymers
The glass transition phase is a critical period for polymers during which material properties change dramatically. This transition is influenced by the molecular architecture and external environmental conditions. Several factors impact whether and how a polymer undergoes a glass transition:
- Chemical Composition: Unique combinations of monomer units lead to variations in flexibility and rigidity, influencing the transition.
- Molecular Dynamics: The phase allows the polymers to move from an ordered glassy state to a disordered rubbery state, increasing mobility.
T_g isn't fixed but can be fine-tuned by modifying polymer structures or adding plasticizers.
Take the case of polyvinyl chloride (PVC), which has a T_g just above room temperature. By adding plasticizers, PVC can become flexible at lower temperatures, making it suitable for various applications such as water hoses and insulation panels.
Delving deeper into molecular interactions during the glass transition reveals remarkable intricacies. For instance, the free volume theory suggests that as temperature increases, so does the free volume within the polymer, facilitating chain movement.Mathematically this relationship can be considered using Williams-Landel-Ferry (WLF) equation:\[\log a_T = \frac{-C_1 (T - T_g)}{C_2 + (T - T_g)}\]where:
- a_T is the shift factor for viscoelastic properties,
- T is the temperature above T_g,
- C_1 and C_2 are material-specific constants.
Effects on Polymer Properties
The glass transition significantly affects several polymer properties, influencing their practical utility in numerous industries. During and after the transition, polymers exhibit distinct changes to attributes such as:
- Mechanical Properties: Transition from a glassy to rubbery state means polymers can elongate more without breaking, impacting tensile strength and elasticity.
- Thermal Properties: As polymers undergo glass transition, their heat capacity and thermal expansion coefficients experience marked shifts.
- Electrical Properties: For conductive polymers, the transition affects electronic configurations due to increased segmental movements, altering conductivity.
Glass Transition Properties
The glass transition significantly influences the properties of materials, affecting how they respond to external forces and conditions. This section explores these properties and their implications in engineering applications.
Physical Properties During Glass Transition
During the glass transition, materials undergo a variety of changes in their physical attributes. Understanding these changes is essential, particularly when working with polymers and glasses.
- Elastic Modulus: As materials transition from a glassy to a rubbery state, they exhibit decreased stiffness. The elastic modulus may drop by several orders of magnitude, providing flexibility.
- Thermal Expansion: Polymers experience an increase in thermal expansion coefficient during transition. This behavior is crucial for applications requiring dimensional stability across temperature ranges.
- Viscoelasticity: The glass transition phase marks a point where materials display both viscous and elastic characteristics, contributing to the damping properties of the material.
- \(k\) is the rate constant
- \(A\) is the pre-exponential factor
- \(E_a\) is activation energy
- \(R\) is the gas constant
- \(T\) is temperature in Kelvin
Consider the case of polycarbonate. It has a glass transition temperature around 147°C, significantly influencing its use in eyewear lenses and electronic housings, where clarity and durability under variable temperatures are vital.
In-depth analysis of the glass transition reveals insights into molecular dynamics. The balance between rigidity and flexibility is explained by theories that model free volume dynamics. The Doolittle equation links viscosity and free volume: \[\eta = \eta_0 \cdot e^{\frac{B}{vf}}\]where:
- \(\eta\) is viscosity, \(\eta_0\) is reference viscosity
- \(B\) is a constant
- \(vf\) is the free volume fraction
Importance in Material Design and Engineering
The glass transition is pivotal when it comes to designing materials intended for a wide array of engineering applications. Its effects inform decisions in product development and material selection.The role of the glass transition in material design includes:
- Improved Flexibility: By controlling T_g, materials can be made to withstand cold environments without becoming brittle.
- Thermal Insulation: Energy-efficient materials that maintain flexibility and structural integrity rely on managing glass transition properties.
- Structural Applications: Establishing accurate T_g values ensures materials like composites maintain desired mechanical properties across variable temperatures.
- \(\tau(T)\) is the relaxation time
- \(\tau_0\) is a pre-exponential factor
- \(B\) and \(T_0\) are material-specific constants
Incorporating nanomaterials can enhance control over T_g, providing new opportunities to fine-tune material properties for innovative uses.
glass transition - Key takeaways
- Glass Transition Definition: A reversible transition in amorphous materials from a hard and brittle state to a molten or rubber-like state, significant in material science and polymer studies.
- Glass Transition Temperature (Tg): The temperature at which glass transition occurs, influenced by factors such as chemical structure, polymer morphology, and cooling rate.
- Glass Transition Phase: Describes the change from a glassy to a rubbery state in amorphous solids like polymers, altering properties such as viscosity and molecular mobility.
- Glass Transition in Polymers: Marks a critical change in polymers' material properties, affecting their behavior and functionality in applications.
- Measuring Glass Transition Temperature: Techniques include Differential Scanning Calorimetry (DSC), Dynamic Mechanical Analysis (DMA), and Thermomechanical Analysis (TMA).
- Glass Transition Properties: During transition, materials exhibit changes in physical attributes like elastic modulus, thermal expansion, and viscoelasticity, crucial for engineering applications.
Learn faster with the 12 flashcards about glass transition
Sign up for free to gain access to all our flashcards.
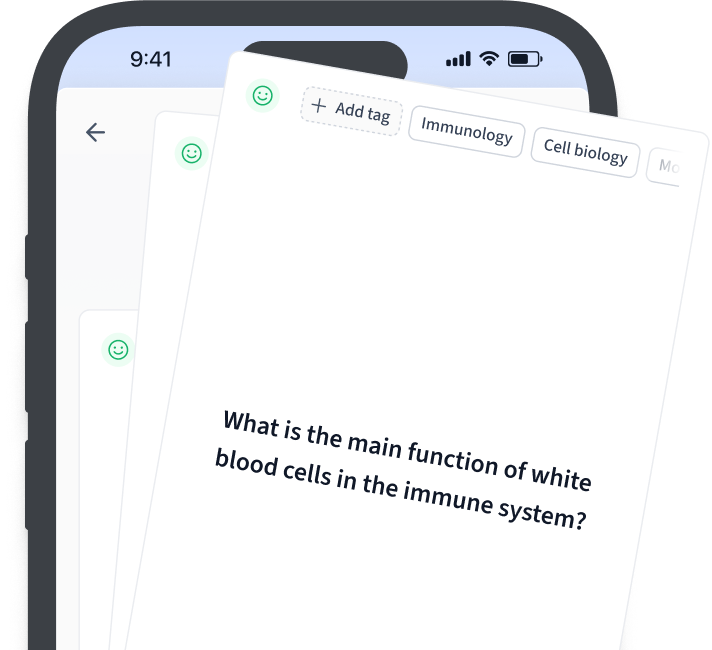
Frequently Asked Questions about glass transition
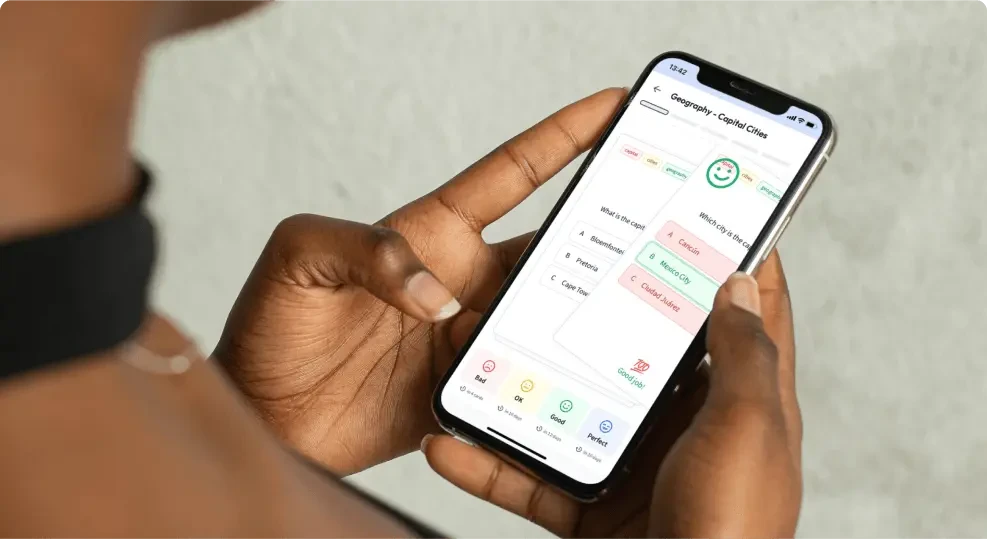
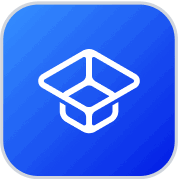
About StudySmarter
StudySmarter is a globally recognized educational technology company, offering a holistic learning platform designed for students of all ages and educational levels. Our platform provides learning support for a wide range of subjects, including STEM, Social Sciences, and Languages and also helps students to successfully master various tests and exams worldwide, such as GCSE, A Level, SAT, ACT, Abitur, and more. We offer an extensive library of learning materials, including interactive flashcards, comprehensive textbook solutions, and detailed explanations. The cutting-edge technology and tools we provide help students create their own learning materials. StudySmarter’s content is not only expert-verified but also regularly updated to ensure accuracy and relevance.
Learn more