Jump to a key chapter
Intermetallics in Chemical Engineering
In the realm of chemical engineering, the study of intermetallics plays a pivotal role. These materials exhibit unique properties that are essential for advanced engineering applications, and understanding them can lead to innovations in various fields such as aerospace and electronics. Dive into the details of intermetallic compounds and their bonding characteristics to enhance your knowledge.
Intermetallic Compound Definition
An intermetallic compound is a solid-state compound exhibiting defined stoichiometry and ordered crystal structures, consisting of two or more metallic elements. These compounds differentiate from regular alloys due to their specific atomic arrangements.
Intermetallic compounds are characterized by their ordered structures and specific stoichiometries, making them distinct from more generic alloys. They are formed when metals combine in precise atomic ratios, resulting in new materials with properties separate from their elemental constituents. These properties might include enhanced mechanical strength, high-temperature stability, and sometimes increased corrosion resistance.
A notable example of an intermetallic compound is Ni_3Al (nickel aluminide). This compound is structured in an ordered two-phase region providing exceptional strength and resistance at high temperatures, making it valuable for turbine blade production in jet engines.
Intermetallic compounds are often used in situations where materials face extreme environments due to their unique property combinations.
Intermetallic Bonding Explained
The bonding in intermetallic compounds combines characteristics of metallic and covalent bonds. This hybrid bonding results in distinct electronic and structural properties. Understanding the nature of these bonds is crucial for leveraging the most desirable attributes of intermetallic materials in practical applications.
Intermetallic bonding can be primarily attributed to the electron sharing mechanisms found within their structure. The electrons are often delocalized across the lattice, similar to metallic bonds, enhancing conductivity. However, unlike pure metallic bonding, the directionality found in these structures can introduce covalent character. The bonding strength in intermetallics can also be attributed to the cohesive energy associated with their ordered structures. This contributes to exceptional mechanical properties at high temperatures, which otherwise might lead to the failure of conventional materials. A mathematical perspective of intermetallic bonding can be addressed by considering the bonding energy E_b, which is often calculated via quantum mechanical principles, accounting for both ionic and covalent contributions: \[ E_b = E_{\text{ionic}} + E_{\text{covalent}} \] This equation suggests that enhancing either ionic or covalent interactions can improve the overall stability and strength of the material. Further exploration and manipulation of E_b have the potential to lead to novel intermetallic materials with tailored properties for specific engineering challenges.
Intermetallic Compound Phase Diagram
Phase diagrams are vital tools in material science, providing insights into the transformations that intermetallic compounds undergo under different temperature and pressure conditions. By understanding phase diagrams, you can predict which phases will form at a given temperature and composition.
Reading a Phase Diagram
When examining a phase diagram, observe the axes, which typically show temperature and composition. Different regions within the diagram represent various phases or combinations of phases. To accurately analyze these diagrams, follow these steps:
- Identify the temperature and composition of the point of interest.
- Examine the phase boundaries to understand transitions between phases.
- Utilize tie lines to determine compositions of coexisting phases.
Consider a simple binary intermetallic phase diagram, often illustrated with a eutectic system. Suppose there are two metals, A and B, with an intermetallic phase 'AB'. The phase diagram will show lines (liquidus and solidus) indicating where different phases, such as A + AB or B + liquid, appear. For example:
Phase | Temperature Range |
Liquid + A | Above eutectic |
AB (Intermetallic) | Mid temperatures |
Understanding equilibrium in intermetallic phase diagrams can be deepened by exploring the Gibbs phase rule: \[ P + F = C + 2 \] where \(P\) is the number of phases present, \(F\) is the degrees of freedom (variables like temperature or pressure that can change without affecting the number of phases), and \(C\) is the number of components. Through this equation, one can determine the possible phase transformations an intermetallic system may undergo by varying composition or temperature. This mathematical approach highlights the complexity in predicting material behaviors under varying thermodynamic conditions.
Importance in Material Science
Intermetallic compounds are integral to material science due to their unique properties, such as high strength-to-weight ratios, corrosion resistance, and thermal stability. By examining phase diagrams, engineers can design materials with specific properties tailored for applications like engine components or electronic devices. Some key importance factors include:
- Advanced mechanical properties at elevated temperatures
- Tailored electrical and magnetic properties for electronics
- Enhanced durability in extreme environments
Intermetallic compounds often bridge the gap between metals and ceramics, combining beneficial properties of both classes.
Intermetallics Phases in Materials Science
Intermetallic phases are a crucial focus within materials science due to their unique properties and applications. These phases arise from specific combinations of metals, resulting in compounds with distinctive crystal structures and attributes. Understanding these phases helps predict the behavior of materials under various conditions, which is pivotal for material development in advanced technologies.
Common Intermetallic Phases
Commonly encountered intermetallic phases include structures such as B2, L12, or Laves phases. Each of these has distinct atomic arrangements and emphasizes different properties, such as impact resistance or corrosion resistance. The B2 phase, for instance, features a body-centered cubic structure known for its high-temperature strength. The L12 structure, conversely, offers a face-centered cubic arrangement typical for compounds like Ni3Al, exhibiting superior toughness.In practical applications, these intermetallics are employed in:
- High-temperature aerospace components
- Corrosion-resistant coatings
- Magnetostrictive materials
A practical example is the gamma titanium aluminide (TiAl) phase, renowned for its lightweight characteristics and high strength at elevated temperatures, making it ideal for aerospace engine components. Its ordered \(\text{L1}_2\) crystalline structure enhances strength and thermal stability.
To delve deeper, consider the mathematical representation of intermetallic phase stability through the Gibbs free energy concept. The phase stability is observed when the change in Gibbs free energy, \(\text{ΔG}\), is negative. This can be expressed as: \[\text{ΔG} = \text{ΔH} - T\text{ΔS}\] where \(\text{ΔH}\) is the change in enthalpy, \(\text{ΔS}\) is the change in entropy, and \(T\) is the temperature. By analyzing \(\text{ΔG}\), you can predict phase stability and transitions under varying thermal conditions, facilitating the enhancement of desired material properties.
Intermetallic phases often display brittleness, which requires careful alloy design to improve ductility without sacrificing strength.
Role in Material Development
Intermetallic compounds play a pivotal role in the development of advanced materials. Their unique combination of properties — such as high strength, low density, and strong electronic and magnetic characteristics — makes them ideal for many high-tech applications. These materials can be engineered to achieve:
- Enhanced energy efficiency in engines
- Extended material lifespan in hostile environments
- Improved performance in electronic devices
Advances in computational materials science allow for the prediction and tailoring of intermetallic properties before physical manufacturing commences.
Intermetallic Alloy Properties
Intermetallic alloys are a complex and fascinating area of materials science, offering unique combinations of properties that make them valuable in advanced engineering applications. These alloys result from precise atomic arrangements of two or more metals, often displaying ordered crystal structures and improved mechanical characteristics compared to their constituent elements.
Mechanical Properties of Intermetallics
The mechanical properties of intermetallic compounds are essential for their use in various industrial applications. These properties include high strength, resistance to creep and wear, and good fatigue performance, especially at elevated temperatures.
Consider Ni3Al (nickel aluminide), which exhibits excellent mechanical strength at high temperatures, making it ideal for aerospace applications. Its ordered structure contributes to this strength and its notable resistance to wear.
Creep resistance refers to a material's ability to resist deformation under mechanical stress at elevated temperatures.
The performance of intermetallics under mechanical stress affects their implementation in engineering. Their impressive creep resistance and wear resistance make them suitable for high-stress applications such as turbine blades in jet engines and power plants. When choosing an intermetallic for a specific mechanical application, assessing its:
- Yield strength and tensile strength
- Fracture toughness
- Fatigue life
While intermetallics offer high strength, they can be brittle, requiring careful design and processing to enhance ductility.
One must consider the role of microstructural engineering in optimizing mechanical properties. Techniques such as alloying and heat treatment can influence grain size, phase distribution, and defect densities in intermetallics, impacting their mechanical behavior. Advanced modeling software allows engineers to predict these interactions, facilitating the development of materials with tailored properties to meet specific engineering challenges.
Applications in Engineering
Intermetallics find extensive applications across various engineering fields due to their superior physical and chemical properties. Key applications include:
- Aerospace: Use in jet engine components due to high temperature and creep resistance.
- Automotive: Lightweight and high-strength materials for engine components.
- Electronics: Usage in connectors and semiconductors because of thermal and electrical stability.
- Energy: Applications in power generation systems, such as combustion chambers and turbine blades.
Intermetallics such as Titanium Aluminide (TiAl) are widely used in the aerospace industry due to their beneficial high-temperature mechanical properties and low density, significantly improving engine efficiency.
By optimizing material properties through advanced manufacturing processes, intermetallics can become even more integral to future technologies.
intermetallics - Key takeaways
- Intermetallic compound definition: Solid-state compounds with defined stoichiometry and ordered crystal structures, consisting of two or more metallic elements.
- Intermetallic bonding: Combination of metallic and covalent bonds, characterized by electron sharing and directionality, contributing to unique electronic and structural properties.
- Intermetallic compound phase diagram: Tool used in materials science to understand the transformations of intermetallic compounds under varying temperature and pressure, guiding phase formation predictions.
- Intermetallics phases in materials science: Specific combinations of metals with distinct crystal structures, creating unique properties and applications in advanced technologies.
- Intermetallic alloy properties: These materials feature ordered atomic arrangements, mechanical strength, high-temperature stability, and corrosion resistance.
- Applications of intermetallics: Extensively used in aerospace, automotive, electronics, and energy sectors due to their superior physical and chemical properties and ability to withstand extreme conditions.
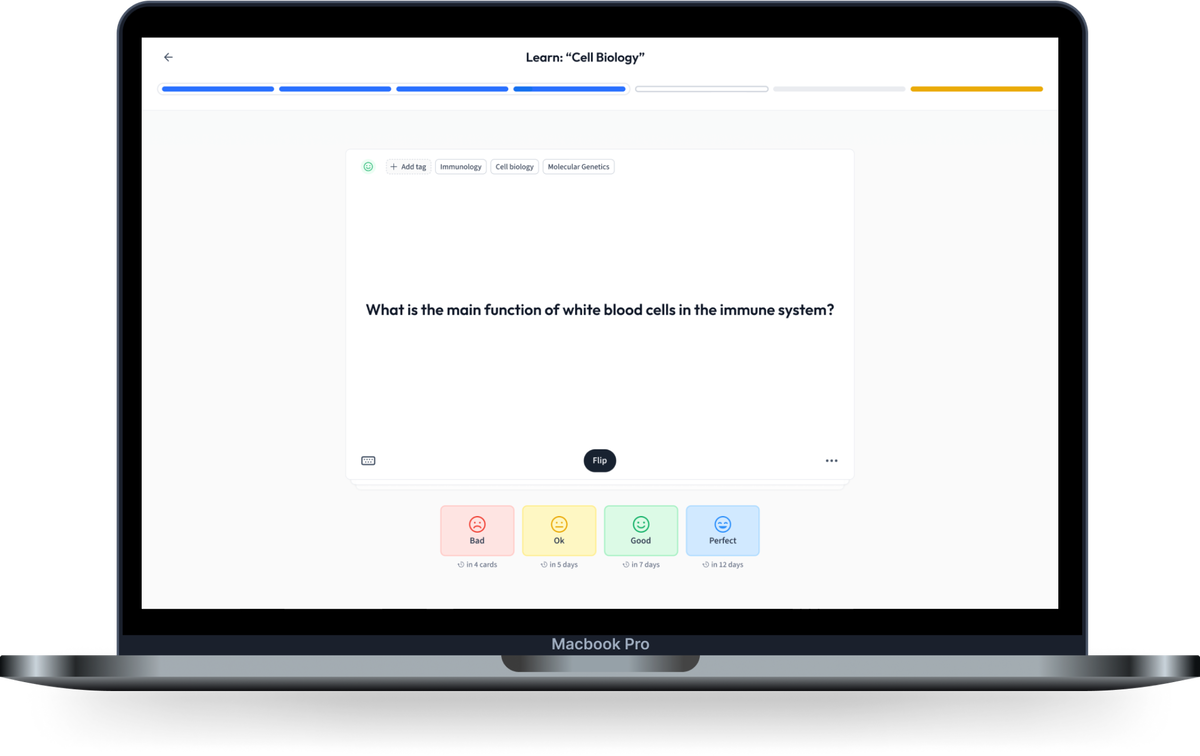
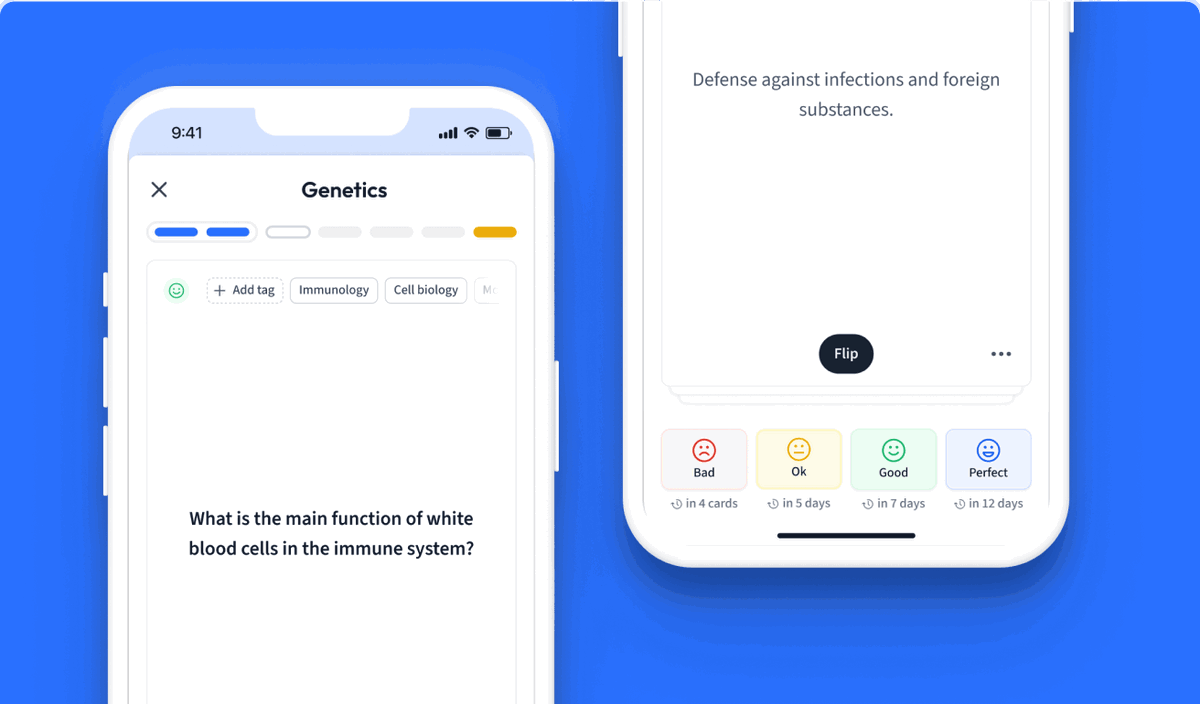
Learn with 12 intermetallics flashcards in the free StudySmarter app
Already have an account? Log in
Frequently Asked Questions about intermetallics
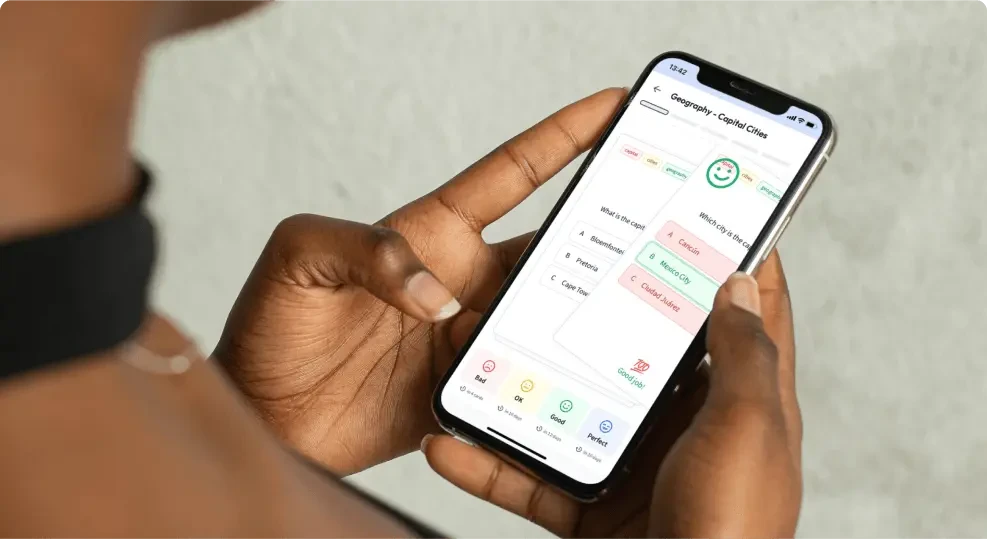
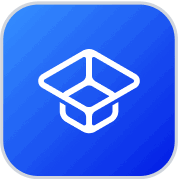
About StudySmarter
StudySmarter is a globally recognized educational technology company, offering a holistic learning platform designed for students of all ages and educational levels. Our platform provides learning support for a wide range of subjects, including STEM, Social Sciences, and Languages and also helps students to successfully master various tests and exams worldwide, such as GCSE, A Level, SAT, ACT, Abitur, and more. We offer an extensive library of learning materials, including interactive flashcards, comprehensive textbook solutions, and detailed explanations. The cutting-edge technology and tools we provide help students create their own learning materials. StudySmarter’s content is not only expert-verified but also regularly updated to ensure accuracy and relevance.
Learn more