Jump to a key chapter
Fundamentals of Ionic Materials in Engineering
Ionic materials are significant in the field of engineering due to their unique properties which arise from electrostatic interactions between charged particles. Understanding these materials requires knowledge of their fundamental concepts, structure, characteristics, and applications.
Basic Concepts of Ionic Materials
Ionic materials are composed of ions, which are atoms or molecules that have gained or lost electrons. This gain or loss of electrons results in ions having a positive or negative charge, respectively. In an ionic compound, these oppositely charged ions come together to form a stable compound through ionic bonds.
Ions are charged particles that form when neutral atoms or molecules gain or lose electrons. Cations are positive while anions are negative.
The crystal lattice structure is a common arrangement in ionic materials, where ions are systematically organized to maximize attraction and minimize repulsion between ions. One of the simplest forms of crystal lattice is the cubic lattice.
For example, sodium chloride (NaCl) is an ionic compound where sodium (\text{Na}^+) and chloride (\text{Cl}^-) ions form a lattice structure. The formula for ionic compounds like NaCl follows the principle of charge neutrality, ensuring that the total positive charge equals the total negative charge.
The electrostatic force that holds ions together in ionic materials is a crucial concept. This force can be calculated using Coulomb's law. The formula is given by \[ F = k_e \frac{|q_1 q_2|}{r^2} \] where
- F is the magnitude of the force between the charges,
- kʀ is the electrostatic constant,
- q_1 and q_2 are the magnitudes of the charges, and
- r is the distance between the centers of the two charges.
The strength of ionic bonds can be related to lattice energy, which indicates the stability of an ionic compound.
Ionic Material Structure and Characteristics
Ionic materials typically have high melting and boiling points due to the substantial energy required to overcome the ionic bonds. They are also good insulators when solid but become conductive when dissolved in a liquid or melted.
The structure of ionic materials is determined by the sizes of the ions and their charges. The arrangement affects the physical properties like solubility and melting point. Ionic materials often have the following structural characteristics:
- Rigid and brittle due to the fixed position of ions within the lattice.
- High density, resulting from the close packing of ions.
A deep dive into the properties of ionic materials reveals insights such as polymorphism. Some compounds can exist in more than one form of crystal structure, known as polymorphs, and each form can exhibit different physical and chemical characteristics. For instance, calcium carbonate exists as calcite and aragonite, each with distinct properties.
Ionic Material Properties in Engineering
In engineering, ionic materials are valued for their electrical conductivity, especially in applications such as batteries and fuel cells. The ability of an ionic material to conduct electricity is due to the movement of ions within the compound when in liquid form or solution.
Solid-state ionic materials like yttria-stabilized zirconia (YSZ) serve as electrolytes in solid oxide fuel cells. These materials allow the passage of oxygen ions, enabling the chemical reactions necessary for electricity generation.
Mechanically, ionic materials possess unique strengths and limitations. They are often strong, but brittleness can be a drawback. Engineering applications often exploit their compression strength rather than tensile strength.
Increased temperature can enhance the ionic conductivity of materials like salts, which is essential in thermal energy storage solutions.
Ionic Material Design
The design of ionic materials is pivotal in developing new technologies and enhancing existing applications in engineering. These materials, reliant on ionic bonds, exhibit properties that can be tailored for specific uses.
Designing Ionic Materials for Specific Applications
Creating ionic materials for specific applications involves understanding the targeted functionality and the properties required for those functions. Ionic materials have applications in various fields, including electronics, energy storage, and biomedicine. Here are some essential aspects of designing ionic materials:
- Chemical Composition: Different ions yield varying properties. For example, incorporating metal ions can enhance conductivity.
- Structure: The arrangement of ions affects mechanical and thermal properties.
- Size and Shape: Micro and nanoscale considerations can optimize surface area and reactivity.
Functionalization in ionic materials is the process of adding specific chemical groups to modify properties for targeted applications, enhancing compatibility with other materials in a composite structure.
For designing ionic materials in batteries, lithium ions are often utilized for high energy density. Solid electrolytes might use lithium lanthanum zirconate (LLZO) to improve efficiency and safety.
In the realm of biomedicine, ionic materials can be tailored for drug delivery systems. Researchers explore encapsulation techniques that utilize ionic gels to release drugs at controlled rates. The concentration gradient, determined by Fick's Law, governs the diffusion: \[ J = -D \frac{dC}{dx} \] where
- J is the diffusion flux,
- D is the diffusion coefficient,
- C is the concentration,
- x is the spatial coordinate.
Temperature and pressure conditions are crucial when designing ionic materials, as they can significantly affect the stability and properties of the final product.
Innovations in Ionic Material Design
Advancements in ionic material design are largely driven by the need for sustainable solutions and enhancing performance in multiple sectors. Innovations often stem from interdisciplinary research bringing together chemistry, physics, and engineering. Some cutting-edge innovations include:
- Electrochemical Applications: Developing novel ionic liquids with low volatility and high thermal stability increases the efficiency of devices like supercapacitors.
- Smart Materials: Ionic materials that respond to environmental stimuli such as temperature or pH, enabling new technologies in sensors and actuators.
- Composite Development: Integrating ionic materials with polymers to create materials with enhanced mechanical properties and flexibility.
An exciting area of research involves ionic thermoelectrics, which convert waste heat to electricity using the Seebeck effect: \[ S = \frac{\text{d}V}{\text{d}T} \] where
- S is the Seebeck coefficient,
- dV is the voltage difference,
- dT is the temperature difference.
Applications of Ionic Materials in Engineering
Ionic materials play a crucial role in engineering, where their unique properties are harnessed across various applications. They are integral to industries such as energy storage, electronics, and environmental engineering. A deep understanding of their properties allows for innovation and advancement in these fields.
Ionic Materials for Batteries and Energy Storage
In the realm of energy storage, ionic materials are indispensable due to their ability to conduct ions effectively, facilitating energy conversion and storage. These materials are essential for designing batteries, particularly lithium-ion batteries, which are the backbone of many electronic devices.
In lithium-ion batteries, the electrolyte is often a liquid ionic material that facilitates the movement of lithium ions between the anode and cathode. This movement is crucial for the charging and discharging cycles, represented by the equation: \[ \text{LiC}_6 + \text{CoO}_2 \rightleftharpoons \text{C}_6 + \text{LiCoO}_2\] This equation shows the reversible reaction key to the function of lithium-ion batteries.
Solid state electrolytes, which are ionic, are being developed to replace liquid electrolytes for improved safety and efficiency in batteries.
An interesting area is the development of sodium-ion batteries as an alternative to lithium-ion batteries. These use sodium ions, creating potential for lower-cost and abundant energy storage solutions. The performance of these batteries can be highlighted with the Nernst equation, which calculates voltage based on ion concentration: \[ E = E^0 - \frac{RT}{nF} \ln Q\] where
- E is the cell potential,
- E^0 is the standard cell potential,
- R is the universal gas constant,
- T is the temperature,
- n is the number of moles of electrons exchanged, and
- F is Faraday's constant,
- Q is the reaction quotient.
Ionic Materials for Batteries
Ionic materials are central to the advancement of battery technology, offering unique properties that enhance performance and efficiency. These materials play a critical role in facilitating ion movement, which is essential for the operation of batteries across different applications.
Types of Ionic Materials in Battery Systems
Battery systems employ various types of ionic materials, each having specific advantages based on their chemical and structural properties. Here are some key types:
- Liquid Electrolytes: Common in traditional batteries, facilitating ion transport between electrodes through a liquid medium.
- Solid Electrolytes: Emerging in advanced batteries to enhance safety by eliminating leakage risks and improving stability.
- Polymer Electrolytes: Flexible materials that combine characteristics of liquids and solids, useful in flexible battery designs.
Solid Electrolytes are ionic materials that conduct ions while in a solid state, providing increased stability and safety in battery systems.
Solid electrolytes are garnering significant attention due to their potential in creating safer batteries. They conduct ions through a mechanism known as ion hopping, where ions move between fixed sites in a lattice structure. To quantify this, consider the formula for ionic conductivity: \[ \sigma = n q \mu \] where
- \sigma is the ionic conductivity,
- n is the charge carrier density,
- q is the charge of the ion,
- \mu is the mobility of the ions.
Advantages of Ionic Materials in Batteries
Ionic materials offer several advantages in battery technology, making them a preferred choice in modern energy solutions:
- High Ionic Conductivity: Allows rapid charge/discharge cycles, increasing the efficiency of batteries.
- Thermal Stability: Ionic materials can withstand high temperatures without degrading, enhancing battery lifespan.
- Safety: Materials like solid electrolytes reduce risks of leakage and fire hazards compared to liquid counterparts.
In lithium-ion batteries, a common type of ionic material used as the electrolyte is lithium hexafluorophosphate in an organic solvent. This combination offers high ionic conductivity and stability within desired operational temperature ranges.
Ionic liquid electrolytes are known for their non-flammability, making them attractive for application in high-safety battery designs.
Future of Ionic Materials in Battery Technology
The future of ionic materials in battery technology is promising, driven by ongoing research into new compositions and structures to meet growing energy demands. Researchers are exploring ways to enhance the energy density, scalability, and safety of these materials.
Advances in ionic materials could lead to:
- Next-Generation Batteries: Development of batteries with higher capacities and faster charging times.
- Environmental Sustainability: Utilization of materials that are more environmentally friendly and easier to recycle.
- Integration with Renewables: Improved compatibility with renewable energy storage systems, facilitating efficient energy management.
One of the exciting prospects in the future of ionic materials is the development of quantum batteries. These batteries theoretically leverage quantum states to provide charge significantly faster than conventional methods. The underlying physics involves exotic ionic material interactions occurring at quantum levels, potentially transforming battery technology.
ionic materials - Key takeaways
- Ionic materials: Composed of ions that are charged particles due to gain or loss of electrons, forming ionic compounds through electrostatic interactions.
- Fundamentals of ionic materials in engineering: Includes understanding their electrostatic force, crystal lattice structure, and ionic bond strength, all crucial for stability and properties.
- Ionic material properties: High melting/boiling points, electrical insulators when solid, become conductive in liquid, rigid and brittle structures, often showing polymorphism.
- Applications of ionic materials in engineering: Vital in batteries, fuel cells, and thermal energy storage, exploiting properties like ion conductivity and compression strength.
- Ionic materials for batteries: Used as electrolytes in lithium-ion and sodium-ion batteries, essential for ion transport, with types including liquid, solid, and polymer electrolytes.
- Ionic material structure and design: Lattice structures affect properties; design involves chemical composition and arrangement optimized for enhancements in mechanical and thermal behavior.
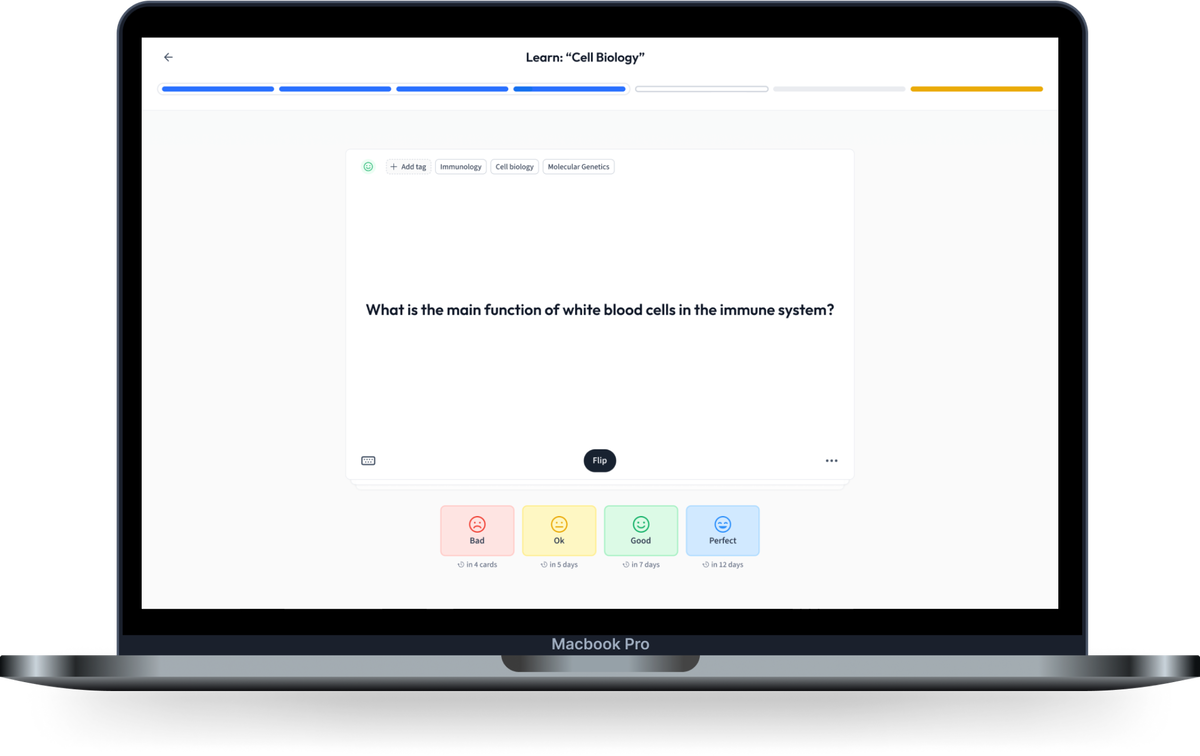
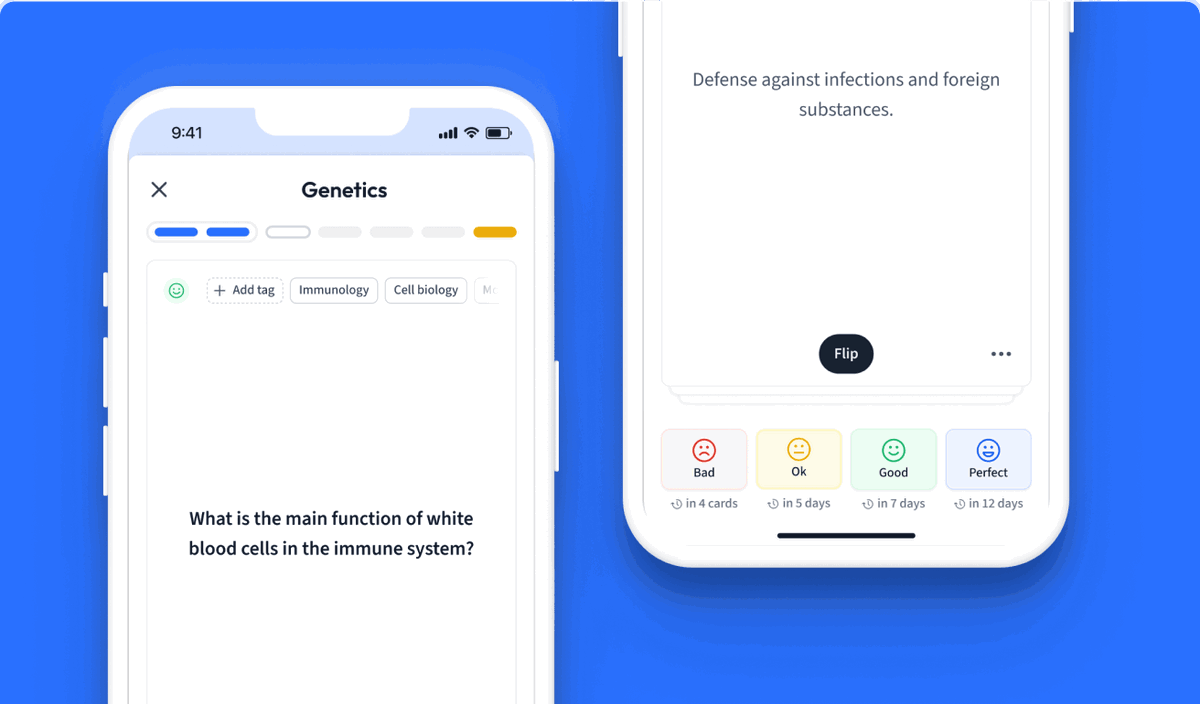
Learn with 12 ionic materials flashcards in the free StudySmarter app
Already have an account? Log in
Frequently Asked Questions about ionic materials
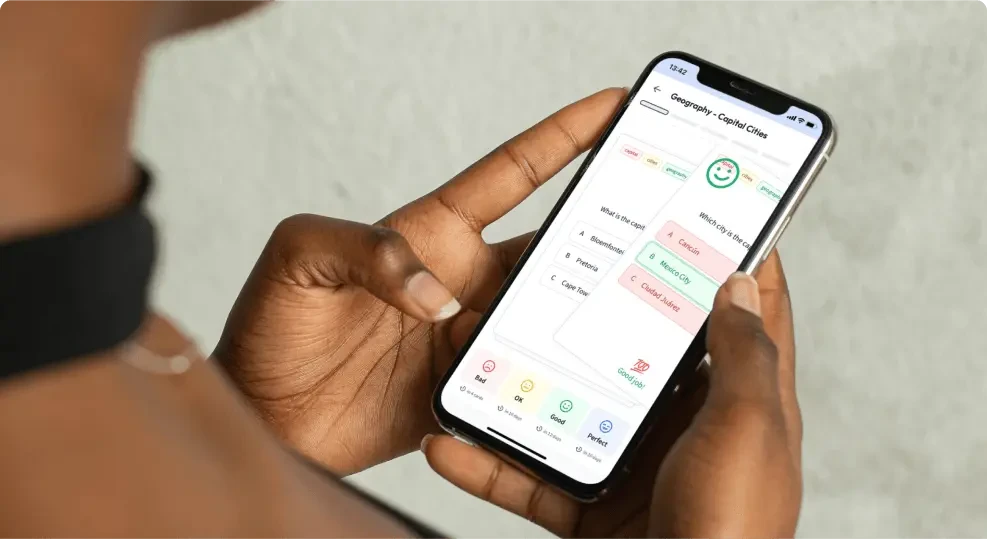
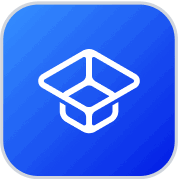
About StudySmarter
StudySmarter is a globally recognized educational technology company, offering a holistic learning platform designed for students of all ages and educational levels. Our platform provides learning support for a wide range of subjects, including STEM, Social Sciences, and Languages and also helps students to successfully master various tests and exams worldwide, such as GCSE, A Level, SAT, ACT, Abitur, and more. We offer an extensive library of learning materials, including interactive flashcards, comprehensive textbook solutions, and detailed explanations. The cutting-edge technology and tools we provide help students create their own learning materials. StudySmarter’s content is not only expert-verified but also regularly updated to ensure accuracy and relevance.
Learn more