Jump to a key chapter
Metal-Organic Frameworks Catalysis Overview
Metal-organic frameworks catalysis is a pivotal field in chemistry, offering diverse applications and vast potential due to its unique properties.
Definition and Importance of Metal-Organic Frameworks Catalysis
Metal-organic frameworks (MOFs) are porous materials composed of metal ions coordinated to organic ligands. These structures create a lattice with a large surface area, ideal for catalysis purposes.
Metal-organic frameworks catalysis is the process of using MOFs as catalysts in chemical reactions. This approach leverages the high surface area, tunable structures, and the ability of MOFs to incorporate various functionalities. These characteristics enable MOFs to play a significant role in enhancing reaction rates and selectivity, making them valuable in fields like chemical synthesis, gas storage, and environmental remediation.
Why are MOFs Important? MOFs have a modular nature, allowing scientists to design and customize them at the molecular level. Some of the key advantages of using MOFs as catalysts include:
- High surface area: Provides more active sites for reactions.
- Thermal and chemical stability: Allows use in diverse conditions.
- Structure tunability: Facilitates specific catalyst design.
Through these properties, MOFs facilitate a variety of catalytic reactions, from traditional transformations to more sustainable and environmentally friendly processes.
MOFs can be synthesized to target specific reactants due to their customizable pore sizes!
An example of MOF application in catalysis is the use of ZIF-8, a zinc-based framework, in converting CO2 into valuable chemicals like methanol, illustrating the potential of MOFs in addressing environmental issues.
Historical Background of Metal-Organic Frameworks Catalysis
The concept of metal-organic frameworks began in the late 20th century. Early researchers discovered that linking metal clusters with organic ligands resulted in the formation of new structures with fascinating properties. As these initial studies progressed, the potential for using MOFs as catalysts became apparent.
During the 1990s, the development of MOFs took a crucial turn. Researchers like Omar M. Yaghi emphasized the importance of creating stable and highly porous materials, which could act as efficient catalysts in various reactions. The simplicity of MOF construction and the ability to modify these materials at a molecular level provided significant incentives for their use in catalysis.
The advent of computational methods further accelerated MOF research. Computational chemistry allowed scientists to predict the structures and behaviors of MOFs, leading to more targeted and efficient designs. By the early 2000s, MOFs had firmly established themselves in the scientific community as promising tools for catalysis and other applications.
In the realm of catalysis, MOFs have shown that they can dramatically enhance performance in reactions such as hydroamination, oxidation, and even in biocatalysis. The versatility of MOFs has enabled the development of cascades of reactions facilitated by these beautifully structured materials, resulting in more sustainable chemical processes, reduced waste, and improved energy efficiency. For example, platinum-modified MOFs have been utilized to create highly efficient electrocatalysts for fuel cell applications.
Techniques in Metal-Organic Frameworks Catalysis
Techniques in metal-organic frameworks catalysis are vital for unlocking the potential of MOFs in various chemical reactions. Understanding these techniques arms you with the tools to enhance reaction efficiency and selectivity.
Experimental Methods for Metal-Organic Frameworks Catalysis
Experimental methods are crucial in studying and utilizing metal-organic frameworks (MOFs) as catalysts. These methods help characterize MOFs, fine-tune their catalytic properties, and optimize reaction conditions for specific applications.
Common Experimental Techniques:
- Synthesis: Creating MOFs involves linking metal ions with organic ligands to form a porous network. Techniques such as solvothermal and hydrothermal synthesis are frequently used.
- Characterization: Techniques like X-ray diffraction (XRD) and scanning electron microscopy (SEM) provide insight into the crystalline structure and morphology of MOFs.
- Catalytic Testing: Evaluating the performance of MOFs in catalysis through setups like gas-phase reactors or liquid-phase batch reactors.
These methods form an integral part of MOF research, allowing precise design and testing of catalysts tailored for specific reactions.
A successful example involves the synthesis of HKUST-1, a copper-based MOF, tested for catalyzing the oxidation of alcohols in a liquid-phase reactor. The experiment demonstrated enhanced reaction rates compared to traditional heterogeneous catalysts.
Paying attention to solvent choice during MOF synthesis can significantly impact the final structural properties!
In-depth Study: Advanced experimental methods such as in-situ spectroscopic techniques allow researchers to observe catalysis as it happens, providing detailed information on reaction pathways and intermediate states. For instance, the use of in-situ infrared spectroscopy can track real-time changes in MOFs during catalysis, shedding light on the dynamic transformations these materials undergo and helping refine the catalytic process for better outcomes.
Advanced Techniques in Metal-Organic Frameworks Catalysis
Advanced techniques in MOFs catalysis involve adopting cutting-edge approaches to enhance and understand catalytic processes further. These techniques take advantage of new technologies and scientific insights.
Emerging Techniques:
- Computational Modeling: Utilizes advanced algorithms to simulate the behavior of MOFs, providing predictive insights on their performance and interactions.
- Post-synthetic Modification: Involves altering MOFs after their synthesis to introduce new functionalities or improve catalytic performance.
- Machine Learning: Harnesses data-driven algorithms to identify patterns and optimize MOF design, accelerating the discovery of effective catalysts.
These advanced methods represent the frontier of MOF catalysis, pushing the boundaries of what these materials can achieve in diverse chemical processes.
Combining experimental data with computational models can streamline the discovery of novel MOF catalysts!
Exploring Innovations: Single-atom catalysis is a groundbreaking approach in MOF research, where single metal atoms are dispersed within a framework, maximizing metal utilization and enhancing catalytic efficiency. This method provides a new avenue for creating powerful catalysts with reduced material costs and improved reaction specificity. For instance, single-atom iron catalysts within a MOF structure have been shown to deliver exceptional activity in CO2 reduction reactions, highlighting their potential in sustainable chemical processes.
Applications in Separations and Catalysis
Metal-organic frameworks (MOFs) are renowned for their exceptional versatility, offering solutions across various domains of chemistry, particularly in separations and catalysis. These applications exploit the molecular structure of MOFs to achieve efficient and specific outcomes.
Metal Organic Frameworks Applications in Separations
Metal-organic frameworks serve crucial roles in separation processes due to their porous nature and selective adsorption properties. These features ensure that MOFs can effectively separate components in various industrial processes.
Key Applications in Separations:
- Gas Separation: MOFs are used to separate gases such as CO2 from N2 due to their tunable porosity.
- Liquid Separation: Used in purifying liquids by removing contaminants or specific molecules.
- Ion Exchange: Effective in water treatment by exchanging toxic ions with harmless ones.
These capabilities make MOFs indispensable in industries such as natural gas purification, carbon capture, and water desalination.
A notable example involves using the MOF known as MIL-101 to separate hydrogen from methane. This MOF exhibits preferential adsorption of larger methane molecules, making it an excellent candidate for hydrogen purification applications.
The effectiveness of MOFs in separations largely depends on their pore size and functional groups, which can be tailored for specific tasks.
Advanced Separation Techniques: MOFs can be engineered to perform chiral separation, where they selectively adsorb specific enantiomers of a chiral molecule. This is particularly useful in pharmaceuticals, where the purity of a specific enantiomer can significantly impact a drug's efficacy and safety. By customizing the framework's architecture, MOFs can achieve highly selective separations that traditional methods might struggle with.
Metal Organic Frameworks Applications in Catalysis
In catalysis, MOFs act as platforms that enhance the rate and sometimes the selectivity of chemical reactions. Their structured pores maximize the accessible surface area and active sites, making them highly effective catalysts.
Applications in Catalysis:
- Organic Reactions: MOFs catalyze various organic transformations, such as cycloadditions and Knoevenagel reactions.
- Environmental Catalysis: They facilitate the breakdown of pollutants into less harmful substances.
- Electrocatalysis: MOFs support efficient energy conversion processes, crucial in fuel cells and batteries.
Through these applications, MOFs provide environmental benefits and energy efficiencies, underscoring their importance in green chemistry.
An innovative application of MOFs in catalysis is their use in CO2 hydrogenation, where MOFs enhance the conversion of CO2 into methanol, a valuable feedstock for industrial processes. The MOF structure ensures high dispersion and accessibility of metal active sites, leading to improved catalytic performance.
To optimize MOF catalysts for specific reactions, researchers often modify the metal centers or introduce functional groups into the organic linkers, significantly influencing catalytic activity.
Exploration of MOF Catalysis: Recent explorations include bimetallic MOFs which incorporate two different metal ions within the same framework. This approach combines the properties of different metals, potentially leading to new catalytic behaviors. Research has shown that bimetallic MOFs can enhance reactions such as the oxidation of hydrocarbons, combining features like increased robustness and dual catalytic sites, which make the frameworks more versatile and potent than their single-metal counterparts.
Computational Modeling of Catalysis in Metal Organic Frameworks
Computational modeling plays a pivotal role in advancing the understanding of catalysis in metal-organic frameworks (MOFs). By simulating molecular interactions within MOFs, these techniques provide insights that are crucial for the design and optimization of catalytic processes.
Role of Computational Modeling in Understanding Catalysis
Computational modeling bridges theoretical chemistry and practical application, enhancing our understanding of catalytic mechanisms in MOFs. These models simulate how MOFs interact with reactants at a molecular level, identifying active sites and predicting reaction pathways.
By enabling the visualization of complex interactions, modeling helps researchers:
- Identify the most active sites within a MOF structure.
- Predict how modifications to the framework affect catalytic efficiency.
- Evaluate the potential of new MOF materials before experimental synthesis.
Through computational modeling, you can streamline the development of more efficient and selective metal-organic framework catalysts, reducing the time and cost of laboratory testing.
Computational studies often employ methods like Density Functional Theory (DFT), allowing for electronic structure calculations and energy predictions. These methods can help in understanding the potential energy surfaces of catalytic reactions within MOFs. For instance, DFT has been used to model the hydrogenation of ethylene with a Palladium-based MOF, providing detailed insights into the activation barriers and reaction intermediates involved.
Computational Tools and Techniques
Utilizing various computational tools and techniques can significantly enhance the study of MOF catalysis. These methods allow for detailed analyses, from molecular interactions to macro-scale behaviors within the frameworks.
Main Computational Techniques:
- Molecular Dynamics (MD): Simulates the physical movements of atoms and molecules, providing insights into the dynamical properties of MOFs.
- Quantum Mechanics/Molecular Mechanics (QM/MM): Combines quantum and classical mechanics to study complex systems where electronic and structural properties influence catalysis.
- Machine Learning: Uses algorithmic approaches to predict the properties of new MOFs and optimize catalytic conditions by analyzing large datasets.
These computational techniques are essential for pioneering new innovations in catalysis through comprehensive and precise simulations.
Combining Molecular Dynamics with Machine Learning can enhance the predictive power of MOF studies, offering more accurate forecasts of catalytic behaviors.
Examples of Catalysis Using Metal-Organic Frameworks
Several studies illustrate the effective use of MOFs in catalysis, demonstrating their ability to catalyze a wide range of reactions.
Case Studies:
- Gas Phase Reactions: MOFs such as HKUST-1 have been employed in gas-phase oxidation reactions, showcasing enhanced selectivity and efficiency.
- Hydrogen Storage: MOFs like MOF-5 facilitate the adsorption and desorption of hydrogen, playing an essential role in hydrogen fuel applications.
- Environmental Catalysis: MOFs have been used to break down pollutants under mild conditions, proving beneficial in water purification processes.
These case studies confirm the versatility and efficiency of MOFs in catalysis, paving the way for advancements in sustainable chemical processes.
An example of effective MOF catalysis is the use of UiO-66 for the conversion of dioxygen to water in organic synthesis, which demonstrated not only high efficiency but also impressive stability under reaction conditions.
Metal Organic Frameworks for Heterogeneous Basic Catalysis
Metal-organic frameworks provide a promising platform for heterogeneous basic catalysis due to their unique structure and functionality.
Characteristics of Heterogeneous Basic Catalysis with MOFs:
- Tunable Acidity/Basicity: MOFs can be designed to have specific acidic or basic sites, enhancing their catalytic efficiency in base-catalyzed reactions.
- Reusability: MOFs are easily recoverable and reusable, offering significant advantages over traditional catalysts.
- Surface Area and Porosity: The large surface area of MOFs increases the number of active sites available for reactions, improving overall reaction rates.
Through these features, MOFs serve as potent catalysts in various reactions requiring basic environments, offering both performance and economic benefits.
Catalysis and Photocatalysis by Metal Organic Frameworks
Metal-organic frameworks are advancing the field of photocatalysis, utilizing their structure to harness and convert light energy for catalysis.
Advantages in Photocatalysis:
- Light Absorption: MOFs can be engineered with light-absorbing components, enabling them to facilitate light-driven reactions.
- Charge Separation: Their structure supports effective charge separation, a critical step in photocatalytic processes.
- Sustainability: Photocatalytic MOFs offer environmentally friendly solutions by utilizing light, often solar, to drive reactions.
Applications of MOFs in photocatalysis include solar energy conversion, water splitting, and organic contaminant degradation.
Through their efficiency and adaptability, MOFs are revolutionizing photocatalytic processes, laying the foundation for sustainable energy solutions.
Exploring Photocatalytic Mechanisms: Recent advances involve the incorporation of semiconductor materials with MOFs to enhance photocatalytic efficiency. For example, integrating titanium dioxide nanoparticles within a MOF has been shown to significantly boost light absorption and improve the degradation rates of organic pollutants in water. This synergy between MOF architecture and semiconductor properties opens new avenues for high-performance photocatalysis.
metal-organic frameworks catalysis - Key takeaways
- Metal-organic frameworks (MOFs): Porous materials made of metal ions coordinated to organic ligands, providing high surface area ideal for catalysis.
- Metal-organic frameworks catalysis: Utilizing MOFs as catalysts to enhance reaction rates and selectivity, important in chemical synthesis, gas storage, and environmental remediation.
- Key techniques in MOFs catalysis: Synthesis, characterization, and catalytic testing are essential for optimizing MOFs' catalytic properties and applications.
- Computational modeling: Advances the understanding of catalysis in MOFs by simulating interactions and predicting reactions, using methods like Molecular Dynamics and Machine Learning.
- Examples of MOF catalysis: Applications include hydrogen storage, environmental catalysis, and the use of bimetallic MOFs for improved catalytic behaviors.
- Photocatalysis by MOFs: MOFs engineered for light absorption and charge separation, enhancing photocatalytic processes for sustainable energy solutions.
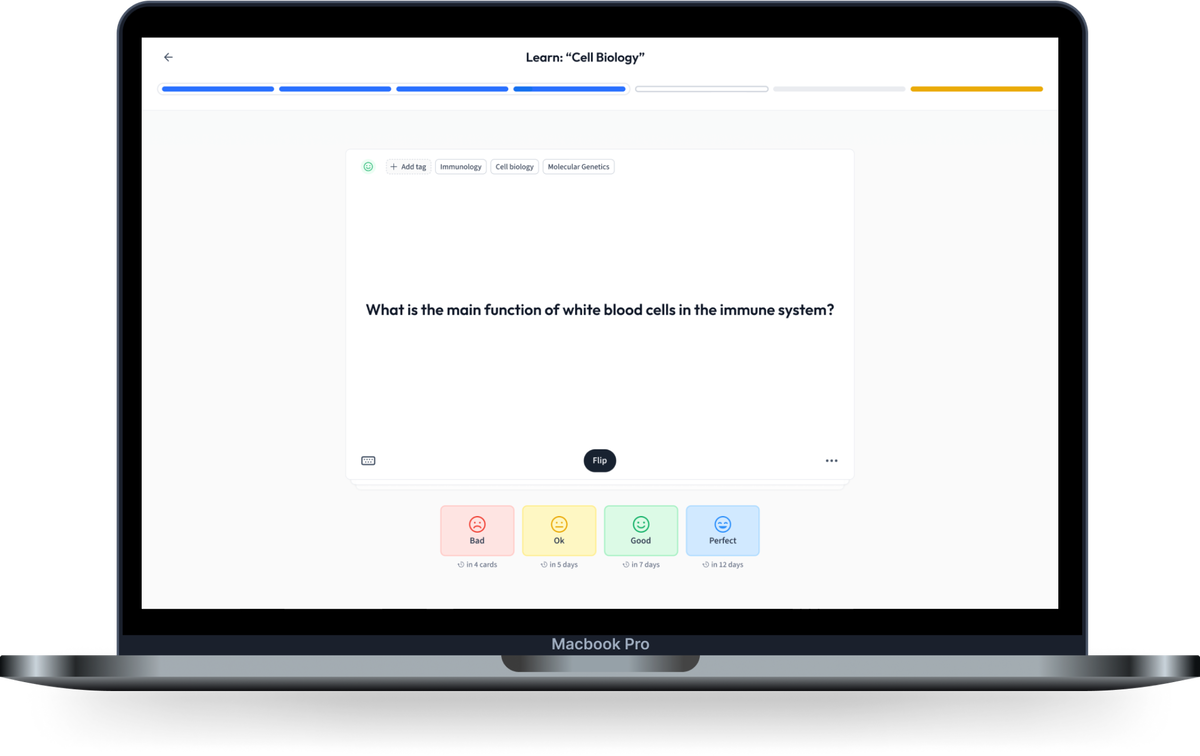
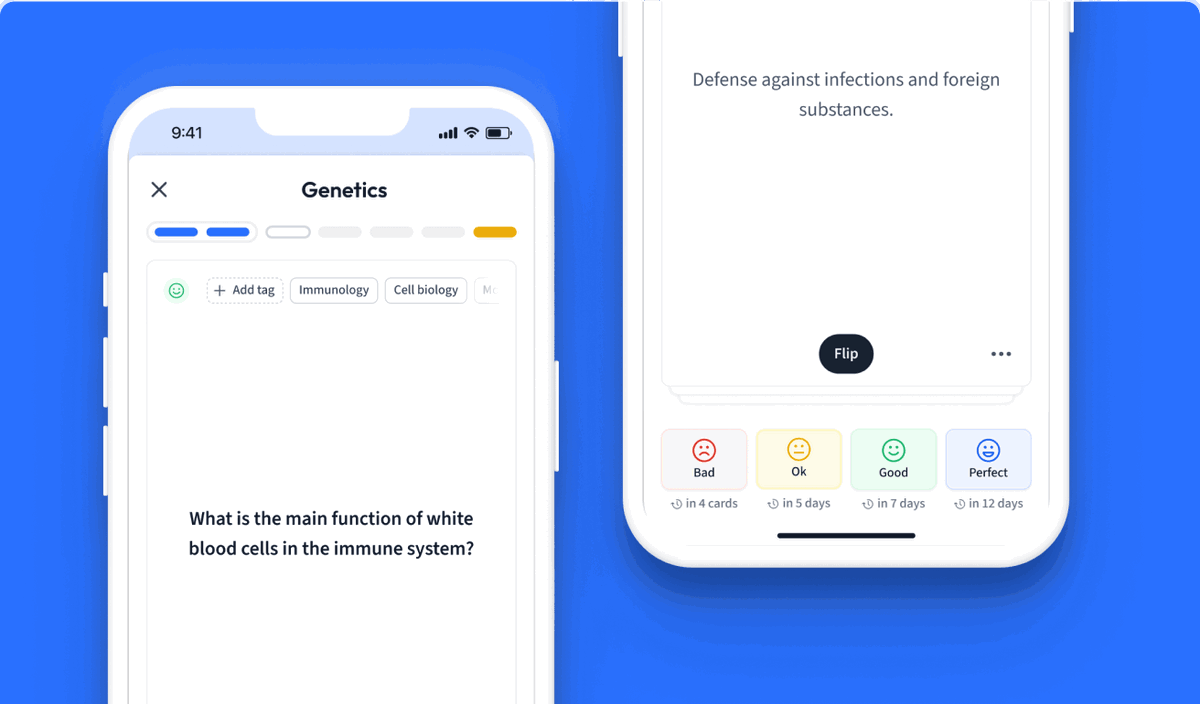
Learn with 12 metal-organic frameworks catalysis flashcards in the free StudySmarter app
Already have an account? Log in
Frequently Asked Questions about metal-organic frameworks catalysis
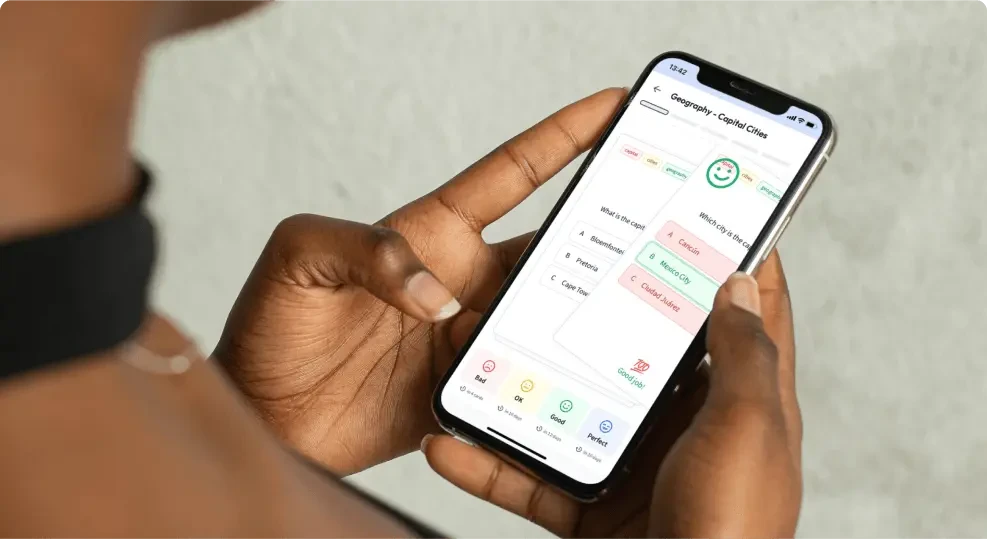
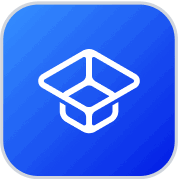
About StudySmarter
StudySmarter is a globally recognized educational technology company, offering a holistic learning platform designed for students of all ages and educational levels. Our platform provides learning support for a wide range of subjects, including STEM, Social Sciences, and Languages and also helps students to successfully master various tests and exams worldwide, such as GCSE, A Level, SAT, ACT, Abitur, and more. We offer an extensive library of learning materials, including interactive flashcards, comprehensive textbook solutions, and detailed explanations. The cutting-edge technology and tools we provide help students create their own learning materials. StudySmarter’s content is not only expert-verified but also regularly updated to ensure accuracy and relevance.
Learn more