Jump to a key chapter
What Is Microbial Fuel Cell?
A Microbial Fuel Cell (MFC) is an eco-friendly and innovative technology that converts chemical energy from organic compounds directly into electricity through the catalytic activity of microorganisms. It offers a promising solution for sustainable energy production and waste reduction.
How Do Microbial Fuel Cells Work?
Microbial Fuel Cells function through the metabolic processes of microorganisms. These microorganisms break down organic matter to produce electricity. Here's a simplified breakdown of how MFCs operate:
- Microorganisms are placed in an anode compartment where they consume organic material.
- During the metabolic process, electrons are released and transferred to the anode.
- The anode releases electrons which then travel through an external circuit to produce electricity.
- Electrons reach the cathode, and protons migrate through a separator to the cathode compartment where they combine with oxygen and electrons to form water.
For instance, if acetate (\text{CH}_3\text{COO}^-\text{H}^+) is used as the fuel, it will be oxidized by bacteria in the anode chamber, producing electrons, protons, and carbon dioxide:\[ \text{CH}_3\text{COO}^- + 2\text{H}_2\text{O} \rightarrow 2\text{CO}_2 + 7\text{H}^+ + 8e^- \]The reaction at the cathode could be:\[ \text{O}_2 + 4\text{H}^+ + 4e^- \rightarrow 2\text{H}_2\text{O} \]
The intricacies of Microbial Fuel Cells are fascinating. The efficiency and effectiveness of MFCs can be influenced by various factors including the type of microorganisms, the substrate used, and the design of the fuel cell. Substrates can range from simple sugars to more complex organic waste. Different bacteria have varying capabilities of transferring electrons either directly or through mediators. This electron transfer mechanism is a rich field of study with potential developments that could significantly boost MFC efficiency.Another point of interest in MFC research is the configuration of the cells. For example, in a two-chambered MFC, there's a distinct anode and cathode compartment separated by a proton exchange membrane. Conditions such as pH levels, temperature, and electrolyte conductivity can vary, drastically impacting performance.Ultimately, the potential of MFCs as a biotechnological tool for simultaneous wastewater treatment and energy production is immense, driven by ongoing research and development efforts.
It’s helpful to remember that different microbes have different capabilities affecting the efficiency of power generation in microbial fuel cells.
How Do Microbial Fuel Cells Work?
Microbial Fuel Cells (MFCs) operate by converting chemical energy in organic materials into electrical energy through the metabolic processes of microorganisms. This process holds significant potential for sustainable energy solutions. Understanding the fundamental operation of MFCs involves exploring several key steps and components.
An MFC primarily comprises of two electrodes - anode and cathode - separated by a proton exchange membrane. Here's how the process unfolds:
- Microorganisms in the anode compartment consume organic matter, releasing electrons and protons.
- The released electrons flow from the anode through an external circuit, generating electricity.
- Protons migrate through the membrane to the cathode compartment.
- At the cathode, electrons and protons combine with oxygen to form water.
Anode Reaction: | \[ \text{CH}_3\text{COO}^- + 2\text{H}_2\text{O} \rightarrow 2\text{CO}_2 + 7\text{H}^+ + 8e^- \] |
Cathode Reaction: | \[ \text{O}_2 + 4\text{H}^+ + 4e^- \rightarrow 2\text{H}_2\text{O} \] |
Consider the example of using glucose as the fuel in an MFC. During the metabolic activity, glucose is oxidized by bacteria at the anode, releasing electrons and protons:\[ \text{C}_6\text{H}_{12}\text{O}_6 + 6\text{H}_2\text{O} \rightarrow 6\text{CO}_2 + 24\text{H}^+ + 24e^- \]
Delving deeper into the electron transfer mechanisms within MFCs reveals a range of complexities. Microorganisms can transfer electrons directly or indirectly utilizing electron shuttles such as flavins or quinones. Understanding these mechanisms is crucial as they can impact the efficiency of electron transfer, defining the power output potential of a microbial fuel cell.Another interesting aspect is the variety of substrates that MFCs can utilize, from simple carbohydrates like glucose to complex organic wastes. The careful selection of substrates and microorganisms enables optimization for specific applications, such as wastewater treatment combined with electricity generation. Recent studies also explore the role of biofilms at the anode, providing insights into how these microbial communities aid in enhanced electron transfer.
Did you know? The efficiency of a microbial fuel cell largely depends on the type of microorganisms and the specific conditions of the fuel cell environment.
Bacteria Used in Microbial Fuel Cells
Bacteria play a crucial role in the efficiency of Microbial Fuel Cells (MFCs). These fuel cells leverage the unique capabilities of bacteria to convert organic matter into electricity, making it essential to understand which bacteria are most effective.
Types of Bacteria in MFCs
Certain bacteria have the unique ability to transfer electrons outside their cell membrane, a process pivotal for electricity production in MFCs. Here's a look at common types used:
- Geobacter spp. - These bacteria are known for their remarkable ability to transfer electrons to external surfaces, enhancing power output.
- Shewanella spp. - Shewanella can reduce a variety of substrates, making them versatile in degrading different organic compounds.
- Pseudomonas spp. - Known for their adaptability, these bacteria can metabolize a wide range of substrates, contributing to their application in diverse environments.
Electron Transfer: A critical function in MFCs, where bacteria transfer electrons from the oxidation of organic matter to the anode. This process fuels the conversion of chemical energy into electrical energy.
For example, let's consider Geobacter metallireducens. These bacteria are capable of oxidizing organic substances like acetate, producing electrons that contribute to electricity generation in MFCs:\[ \text{CH}_3\text{COO}^- + 2\text{H}_2\text{O} \rightarrow 2\text{CO}_2 + 7\text{H}^+ + 8e^- \]Electrons (\text{e}^-) produced are transferred to the anode, creating an electric current.
Diving deeper into the world of MFCs, the metabolic pathways utilized by bacteria play a substantial role in energy production efficiency. Some bacteria utilize direct electron transfer mechanisms, such as cytochromes, while others use intermediaries like shuttling molecules. The choice of bacteria can significantly affect the operational parameters of an MFC, including voltage output and substrate degradation rates.Biofilm formation on the anode surface is another fascinating aspect. Bacteria in biofilms are more stable and can enhance electron transfer rates due to closer proximity of cells to the anode. Research continues to explore optimal conditions like pH levels, temperature, and electron transfer mediators for maximizing the potential of these microbial communities.
Understanding the specific metabolic capabilities of different bacteria can improve the design and efficiency of MFCs.
Microbial Fuel Cell Construction
A Microbial Fuel Cell (MFC) is an apparatus designed to convert organic matter into electricity using microorganisms. The construction of an MFC is essential for its efficiency and involves several critical components and design considerations.
Basic Components of an MFC
The fundamental components involved in constructing a Microbial Fuel Cell include:
- Anode – where microorganisms oxidize organic matter, releasing electrons and protons.
- Cathode – where electrons combine with protons and oxygen to form water.
- Proton Exchange Membrane (PEM) – separates the anode and cathode while allowing protons to pass through.
- External Circuit – the path through which the electrons flow to generate electricity.
Proton Exchange Membrane: A semi-permeable membrane that separates the anode and cathode compartments, allowing protons to pass while blocking ions, crucial for maintaining charge balance.
Consider a two-chamber MFC design:
Chamber Type | Description |
Anode | Contains the microbes and substrate, facilitating oxidation. |
Cathode | Receives protons from the anode through the membrane. |
The architecture of MFCs can significantly affect their performance. For instance, in a single-chamber MFC, the air cathode is more accessible to oxygen which can improve reactions, although it may compromise the structural integrity compared to dual-chamber systems. Another consideration is the use of activated carbon or platinum-coated electrodes which can enhance conductivity and overall efficiency.Another factor is the scaling of MFCs; scalability is a challenge because larger systems require more robust structural components to handle increased loads and maintain efficiency over long periods. Researchers are experimenting with various materials for electrodes and membranes and investigating how reactor size and flow rates impact the energy production.
Experimenting with different materials for the electrodes in an MFC can lead to significant improvements in efficiency and durability.
Microbial Fuel Cell Applications
Microbial Fuel Cells (MFCs) offer a range of exciting and innovative applications due to their ability to convert waste into valuable energy. By leveraging the power of microorganisms, MFCs can contribute significantly to sustainable technologies and environmental remediation.
Wastewater Treatment
One of the most promising applications of MFCs is in wastewater treatment. MFCs can treat wastewater while simultaneously generating electricity, offering a two-fold benefit. This process effectively reduces the organic load and contaminant levels in sewage.During this process, microorganisms metabolize and degrade the organic compounds. The conversion of this chemical energy is represented in the cathodic reaction:\[ \text{Organic\text{ }Matter} + \text{Microbes} \rightarrow \text{CO}_2 + \text{H}_2\text{O} + \text{Electricity} \]
For instance, in practical applications, treating municipal sewage with an MFC may achieve both the removal of biochemical oxygen demand (BOD) and the recovery of electrical energy. This dual function enhances the sustainability of wastewater treatment plants.
Remote Power Supply
Microbial Fuel Cells are particularly useful for providing power in remote or inaccessible environments where traditional energy sources are impractical. In areas such as deep-sea exploration and space missions, MFCs can serve as a reliable power source.These cells utilize available organic or synthetic substrates to provide a continuous power supply. The compact nature and low operational requirements make MFCs an attractive option for powering sensors and monitoring devices.
Microbial fuel cells' capability to function in remote areas makes them ideal for long-term environmental monitoring.
Bioenergy and Bioremediation
Beyond just energy production, MFCs also play an essential role in bioremediation, a process that uses microorganisms to degrade environmental pollutants. This dual functionality ensures that MFCs can convert hazardous chemicals into non-toxic byproducts while generating energy.The effectiveness of this approach depends on several factors including the type of microorganism and the pollutant target. Mathematical models are used to optimize these conditions:\[ P = \frac{V^2}{R} \]where P is the power output, V is the voltage, and R is the resistance, illustrating basic electrical principles applied in MFC optimization scenarios.
The role of MFCs in bioenergy is deeply intertwined with efforts towards a green economy. By integrating MFC systems with algal bioreactors, further efficiencies can be achieved through the simultaneous production of electricity and biomass. This integration aims to interconnect waste treatment, energy production, and agriculture, paving the way for novel sustainable solutions. The field is continually evolving with research focusing on enhanced microbial pathways, better electrode materials, and optimized configurations to maximize the full potential of MFC technology. Innovative approaches like microbial electrosynthesis also unlock new possibilities for the synthetic production of valuable chemical compounds using electricity produced by MFCs.
microbial fuel cells - Key takeaways
- Definition of Microbial Fuel Cells: An eco-friendly technology that converts chemical energy from organic compounds into electricity using microorganisms.
- How Microbial Fuel Cells Work: Involves microorganisms breaking down organic matter, releasing electrons and protons, generating electricity as electrons pass from the anode to cathode through an external circuit.
- Bacteria Used in Microbial Fuel Cells: Key bacteria include Geobacter, Shewanella, and Pseudomonas, which are effective in electron transfer crucial for electricity generation in MFCs.
- Microbial Fuel Cell Construction: Involves components like anode, cathode, proton exchange membrane, and external circuit, critical for the cell's operation.
- Microbial Fuel Cell Applications: Used for wastewater treatment, providing remote power supply, and bioenergy/bioremediation by converting waste into energy.
- Efficiency Factors: Efficiency influenced by microorganism type, substrate selection, cell design, pH levels, and temperature conditions.
Learn faster with the 10 flashcards about microbial fuel cells
Sign up for free to gain access to all our flashcards.
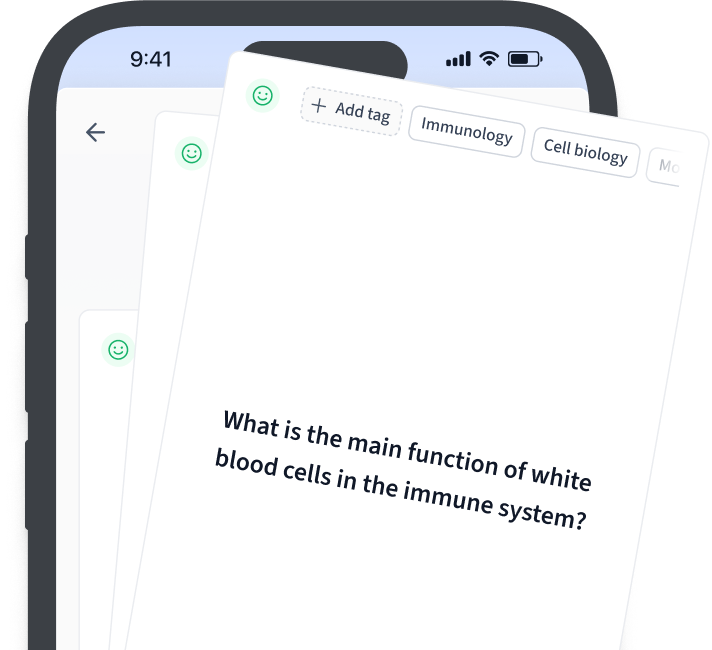
Frequently Asked Questions about microbial fuel cells
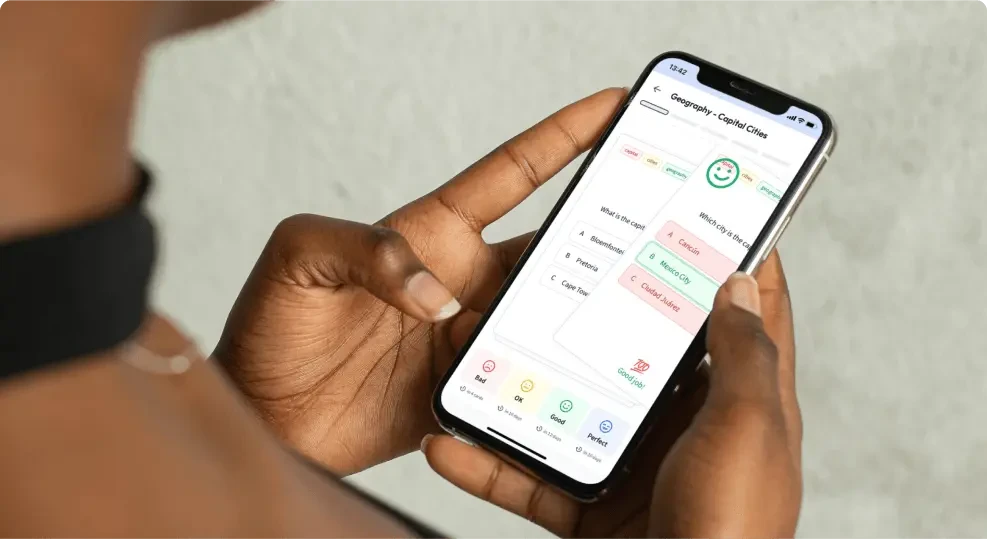
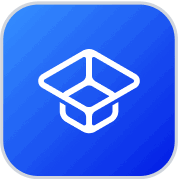
About StudySmarter
StudySmarter is a globally recognized educational technology company, offering a holistic learning platform designed for students of all ages and educational levels. Our platform provides learning support for a wide range of subjects, including STEM, Social Sciences, and Languages and also helps students to successfully master various tests and exams worldwide, such as GCSE, A Level, SAT, ACT, Abitur, and more. We offer an extensive library of learning materials, including interactive flashcards, comprehensive textbook solutions, and detailed explanations. The cutting-edge technology and tools we provide help students create their own learning materials. StudySmarter’s content is not only expert-verified but also regularly updated to ensure accuracy and relevance.
Learn more