Jump to a key chapter
Molecular Orbitals and Molecular Orbital Theory
Understanding molecular orbitals and the associated theory is crucial for grasping how atoms combine to form molecules. These concepts help explain the behavior of electrons within molecules, ultimately influencing chemical properties and reactions.
Introduction to Molecular Orbital Theory
The Molecular Orbital Theory provides a method for describing the electronic structure of molecules. Unlike other models that focus solely on individual atoms, this theory extends the concept of atomic orbitals to molecules. This model allows you to visualize electrons as being associated with an entire molecule rather than localized around a single atom.Molecular orbitals are formed from the combination of atomic orbitals when atoms bond. These orbitals can be bonding, antibonding, or non-bonding:
- Bonding orbitals: Lower in energy than the original atomic orbitals, and enhance stability when occupied.
- Antibonding orbitals: Higher in energy and tend to decrease stability when occupied.
- Non-bonding orbitals: Do not significantly affect the energy of the molecule.
Consider the hydrogen molecule (H2). Each hydrogen atom has one 1s atomic orbital. When these hydrogen atoms bond, their atomic orbitals combine to form molecular orbitals. The results are a \sigma_s bonding orbital and a \sigma_s^* antibonding orbital. The \sigma_s orbital is lower in energy, and when occupied by two electrons, it stabilizes the molecule.
Principles and Concepts of Molecular Orbitals
In Molecular Orbital Theory, the combination of atomic orbitals must adhere to several principles to form molecular orbitals. These principles include:
- Linear Combination of Atomic Orbitals (LCAO): This is a mathematical method that suggests the formation of molecular orbitals is the result of linear combinations of atomic orbitals.
- Conservation of Orbitals: The number of molecular orbitals formed equals the number of atomic orbitals combined.
- Symmetry and Phase: Overlapping atomic orbitals must possess compatible symmetry and phases for effective bonding.
Bond Order: This term is a measure of the stability of a bond. It is calculated using the formula: \[ \text{Bond Order} = \frac{(\text{Number of electrons in bonding orbitals} - \text{Number of electrons in antibonding orbitals})}{2} \] In general, a higher bond order indicates a stronger, more stable bond.
Molecular Orbital Diagrams are essential tools that visually represent molecular orbital energy levels and can be used to deduce bond orders for molecules.
Molecular orbitals can also be understood using quantum mechanics. Schrödinger's equation, a foundational principle in quantum mechanics, helps derive the wave functions (Ψ) of electrons. When solving Schrödinger's equation for molecules, every electron's behavior is described in a molecular orbital, analogous to how atomic orbitals describe electrons in an atom.Mathematically, when atomic orbitals are combined, they can be represented by wave functions: \[ \Psi_{MO} = c_1 \Psi_{AO1} + c_2 \Psi_{AO2} \] where \( c_1 \) and \( c_2 \) are coefficients that adjust the weight of each atomic orbital's contribution.Moreover, molecular orbitals constructed by symmetrical combination (in-phase) of atomic orbitals result in increased electron density between nuclei, leading to bonding interactions. Antisymmetrical combination (out-of-phase) results in nodes between nuclei and antibonding interactions.These interactions fundamentally affect a molecule’s chemical and physical properties, including color, magnetism, and reactivity.
Molecular Orbitals: Bonding and Antibonding
Molecular orbitals are fundamental concepts in chemistry, providing insights into how atoms connect through electrons. Bonding orbitals and antibonding orbitals arise when atomic orbitals combine, affecting the resultant molecule's stability and properties.
Bonding Molecular Orbital Explanation
A bonding molecular orbital is formed by the constructive interference of atomic orbitals. This combination leads to an increase in electron density between the atomic nuclei, enhancing the attraction between them.The energy of a bonding orbital is lower than that of the original atomic orbitals. This stability is one of the main reasons molecules form.Consider an analogy where
- Atomic orbitals combine like musical notes harmonizing to produce a richer, more stable sound.
- Constructive interference acts like two waves overlapping to make a stronger, larger wave.
In the H2 molecule, the combination of 1s orbitals from two hydrogen atoms forms a bonding orbital: \( \sigma_{1s} \). It lowers the system's energy, which is why diatomic hydrogen exists naturally.
Apart from the typical sigma (\( \sigma \)) orbitals, another type is pi (\( \pi \)) orbitals. While \( \sigma \) orbitals overlap head-to-head, \( \pi \) orbitals overlap side-by-side. These interactions are prevalent in multiple-bonded systems like double bonds (as in ethene), where one \( \sigma \) and one \( \pi \) bond form.Mathematically, a \( \pi \) bond can be depicted as: \[ \Psi_{\pi} = c_1 \Psi_{2pA} - c_2 \Psi_{2pB} \]Notice the negative sign, indicating a different type of overlap and symmetry compared to \( \sigma \) bonding.
Antibonding Molecular Orbital
An antibonding molecular orbital arises from the destructive interference of atomic orbitals. This type of interference causes a node, or region of zero electron density, between atomic nuclei, reducing the favorable conditions for bond formation.Antibonding orbitals have higher energy levels than their constituent atomic orbitals, often reducing a molecule's stability when populated by electrons.The antibonding orbital can be represented as: \[ \Psi_{ab} = c_1 \Psi_{1sA} - c_2 \Psi_{1sB} \] where the subtraction symbolizes the out-of-phase overlap.Key attributes include:
- An increase in energy relative to atomic orbitals.
- A node where electron density is minimized.
Antibonding orbital energy: Refers to the energy level of electrons in the antibonding configuration, higher than that of individual atomic orbitals.
Symbols like \( \sigma^* \) and \( \pi^* \) indicate antibonding molecular orbitals, with the asterisk denoting an antibonding designation.
The significance of antibonding orbitals extends to molecular stability evaluations and spectroscopy.In spectroscopy, electronic transitions often involve movement between bonding and antibonding orbitals, influencing molecular absorption spectra. A classic example includes the UV-Vis spectroscopy of conjugated systems where \( \pi - \pi^* \) transitions absorb UV light.Understanding antibonding orbital interactions also sheds light on chemical reactivity patterns, especially in catalysis and coordination chemistry.A thorough comprehension of electron distribution assists in predicting reaction mechanisms and molecular geometry, leading to innovations in fields such as pharmaceutical design and material sciences.
Molecular Orbital Diagram
A molecular orbital diagram visually represents the energy levels of molecular orbitals, formed from the combination of atomic orbitals. These diagrams are essential tools in molecular chemistry, helping you understand how electrons are distributed in a molecule and predict the molecule's properties.
Understanding Molecular Orbital Diagram
The Molecular Orbital Diagram is a graphical representation that provides a map of the molecular structure and behavior by illustrating energy levels of molecular orbitals.To understand these diagrams, it's important to recognize the flow and interaction of atomic orbitals leading to the formation of molecular orbitals. The main components include:
- Atomic Orbitals: Depicted on the sides of the diagram.
- Molecular Orbitals: Placed centrally and horizontally.
- Bonding and Antibonding: Bonding orbitals are positioned lower, while antibonding orbitals are higher in energy.
Consider the diatomic nitrogen molecule (N2). Its molecular orbital diagram includes overlapping 2s and 2p atomic orbitals. Electrons populate the bonding orbitals such as \(\sigma_{2s}\) and \(\pi_{2px, 2py}\) before populating the antibonding orbitals. This results in a bond order of 3, indicating a triple bond.
Remember, in homonuclear diatomic molecules, like O2 or N2, the MO energy levels might adjust based on the periodic group, altering the position of \(\sigma\) and \(\pi\) orbitals.
In complex systems, Molecular Orbital Diagrams become multifaceted due to hybridization and interactions between different kinds of atomic orbitals, such as s-p mixing.Consider octahedral coordination complexes often found in transition metal chemistry. Here, molecular orbitals arise from d-orbitals of the metal and p-orbitals of ligands, forming \(\sigma\), \(\pi\), and \(\delta\) interactions. The energy of these MOs can influence properties like magnetism and color.Quantum Chemistry calculations often utilize computational methods, such as Density Functional Theory (DFT), to create complex MO diagrams to predict chemical reactions' outcomes, aiding in the design of new materials or pharmaceuticals.This blending of computational and theoretical chemistry underlines the diverse applications and expansive understanding possible through molecular orbital diagrams.
Electronic Configuration of Molecular Orbital
The electronic configuration of a molecular orbital is crucial for understanding the distribution and activity of electrons within molecules.Electronic configurations provide a systematic method of placing electrons in molecular orbitals based on increasing energy levels. The main rules followed include:
- Aufbau Principle: Electrons fill MOs from the lowest to the highest energy level.
- Hund's Rule: Electrons occupy degenerate orbitals singly before pairing.
- Pauli Exclusion Principle: Each molecular orbital can hold a maximum of two electrons with opposite spins.
For an O2 molecule, the electronic configuration following the above rules is: \[(\sigma_{1s})^2(\sigma_{1s}^*)^2(\sigma_{2s})^2(\sigma_{2s}^*)^2(\pi_{2p_x})^2(\pi_{2p_y})^2(\sigma_{2p_z})^2(\pi_{2p_x}^*)^1(\pi_{2p_y}^*)^1\]This leads to a bond order of 2, indicating a double bond, and the presence of unpaired electrons results in paramagnetism.
Using the molecular orbital theory, the order of filling levels impacts the molecular geometry and potential energy, a vital concept in chemical kinetics.
Going deeper into the implications of electronic configurations, one finds fascinating phenomena such as:- In organo-metallic compounds, electrons are spread over a vast area, impacting reactivity and catalyst design, crucial in industrial applications.- High-energy electronic transitions, often observed in spectroscopy, result from electrons moving between molecular orbitals of different configurations, influencing observed chemical emissions and absorptions.This expansive view presented by electronic configurations underscores the interplay between molecular orbitals and practical applications, ranging from materials science to biochemical processes.Modern computational tools allow researchers to simulate electronic configurations of large molecules, aiding in drug discovery and the development of new materials with tailored properties. These simulations utilize quantum mechanics to predict how modifications at the atomic level influence electronic distributions and subsequent molecular behaviors.
Linear Combination of Atomic Orbitals
The Linear Combination of Atomic Orbitals (LCAO) is a fundamental concept in chemistry used to describe how atomic orbitals combine to form molecular orbitals. This method translates the complex interplay of atomic interactions into a manageable mathematical model, helping to define molecular structures and their electronic configurations.
Role in Molecular Orbitals
The role of LCAO in forming molecular orbitals is significant as it helps predict the electronic distribution across molecules. Utilizing LCAO, atomic orbitals of participating atoms combine and form molecular orbitals. These molecular orbitals can be classified into bonding and antibonding types based on their energy levels and electron density distribution.The linear combination approach follows certain principles and rules, primarily:
- Conservation of Orbitals: The total number of molecular orbitals equals the number of atomic orbitals combined.
- Symmetry Considerations: Only atomic orbitals with similar energy levels and compatible symmetry will effectively combine.
- Phase Compatibility: Constructive interference for bonding (in-phase overlap) and destructive interference for antibonding (out-of-phase overlap).
Linear Combination of Atomic Orbitals (LCAO): A method used to approximate molecular orbitals by combining atomic orbitals linearly, preserving the number of orbitals and emphasizing symmetry and phase.
LCAO is a valuable tool for visualizing the transition from atomic to molecular orbitals, linking quantum mechanics to molecular geometry.
In quantum chemistry, the application of LCAO extends beyond simple molecules to larger systems. For instance:- In biological systems like DNA, LCAO aids in understanding how nucleotide bases interact via hydrogen bonds at the molecular level.- LCAO also applies to transition metal complexes in coordination chemistry, where atomic orbitals from metals and ligands combine to form intricate patterns of interaction and bonding.Advanced computational methods involving LCAO allow researchers to predict a molecule’s energy states and properties accurately. This capability is crucial in nanotechnology for designing materials at the atomic scale with desired electronic or magnetic properties. The expansion of LCAO into these diverse scientific fields highlights its adaptability and relevance to both theoretical and applied chemistry.
Example of Linear Combination
An exemplary use of the LCAO method is the description of molecular orbitals in a simple molecule such as hydrogen fluoride (HF). The formation of HF involves the combination of:
- 1s orbital from hydrogen.
- 2p orbitals from fluorine.
molecular orbitals - Key takeaways
- Molecular Orbital Theory: Describes the electronic structure of molecules by extending atomic orbitals to the whole molecule, unlike models focusing on individual atoms.
- Molecular Orbitals: Formed by the combination of atomic orbitals, and can be bonding (lower energy), antibonding (higher energy), or non-bonding.
- Bonding Molecular Orbitals: Formed by constructive interference, leading to increased electron density between atomic nuclei, thus stabilizing the molecule.
- Antibonding Molecular Orbitals: Result from destructive interference causing higher energy levels and nodes between nuclei, reducing molecular stability when occupied.
- Electronic Configuration of Molecular Orbitals: Arranges electrons in molecular orbitals following principles like Aufbau and Hund's rule to predict molecular properties.
- Linear Combination of Atomic Orbitals (LCAO): A method that predicts molecular orbital formation by combining atomic orbitals for molecules, maintaining conservation of orbitals and considering symmetry and phase.
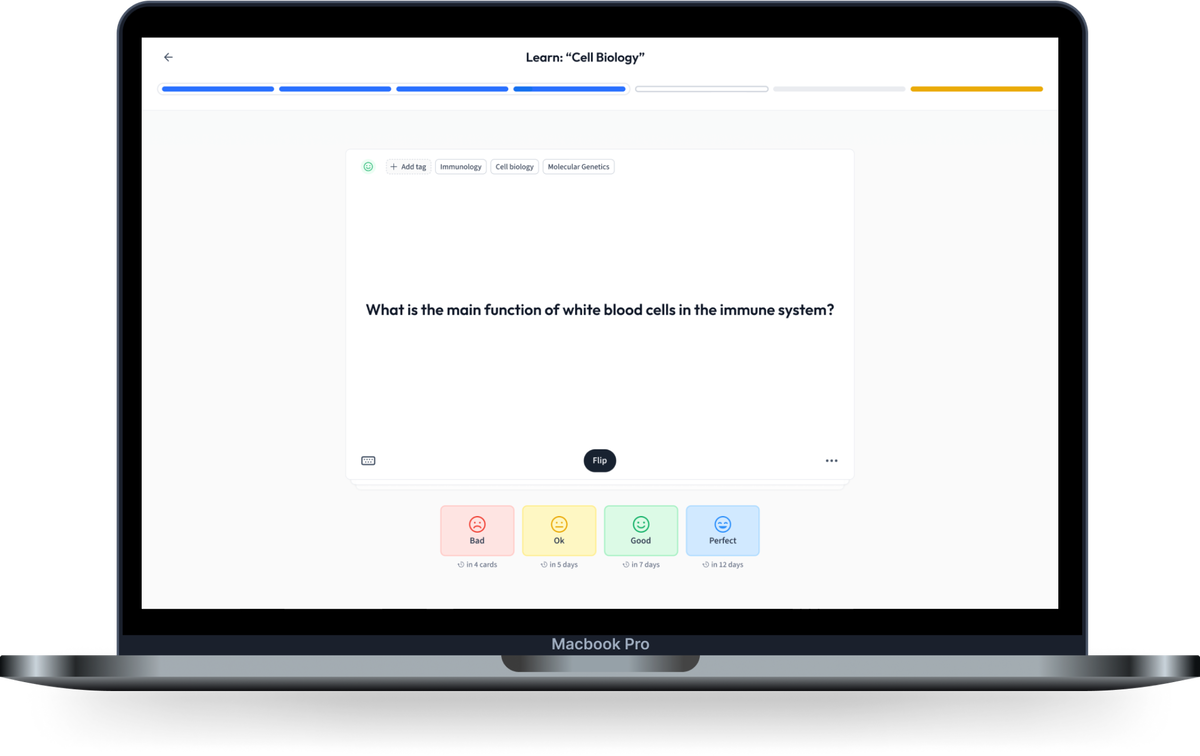
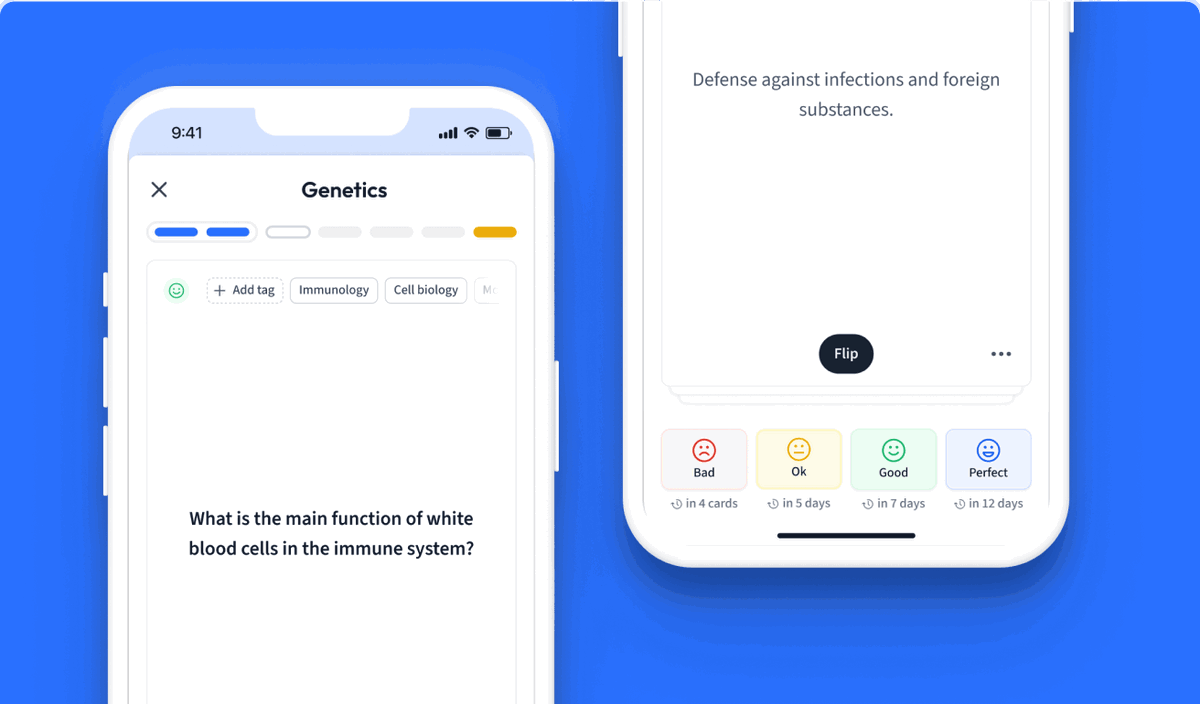
Learn with 12 molecular orbitals flashcards in the free StudySmarter app
Already have an account? Log in
Frequently Asked Questions about molecular orbitals
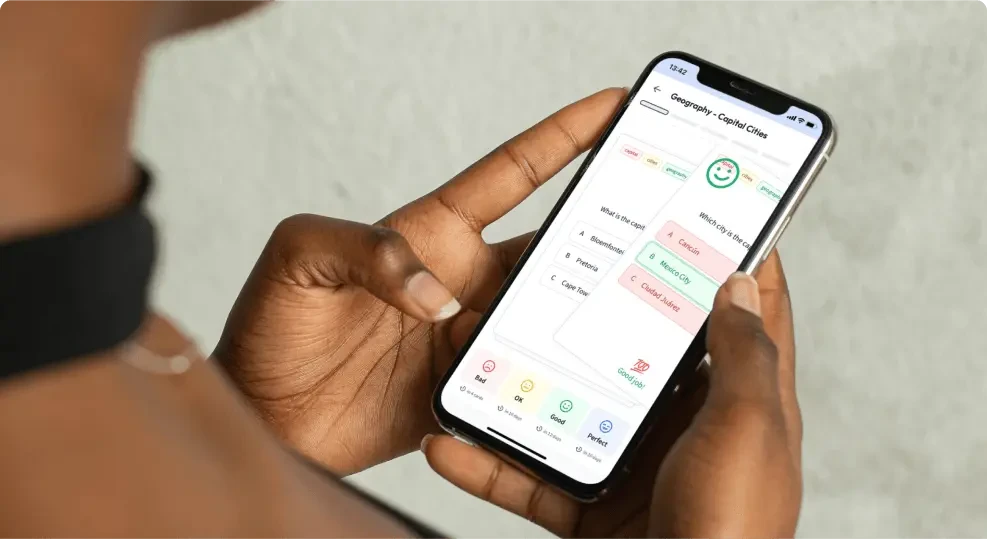
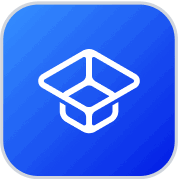
About StudySmarter
StudySmarter is a globally recognized educational technology company, offering a holistic learning platform designed for students of all ages and educational levels. Our platform provides learning support for a wide range of subjects, including STEM, Social Sciences, and Languages and also helps students to successfully master various tests and exams worldwide, such as GCSE, A Level, SAT, ACT, Abitur, and more. We offer an extensive library of learning materials, including interactive flashcards, comprehensive textbook solutions, and detailed explanations. The cutting-edge technology and tools we provide help students create their own learning materials. StudySmarter’s content is not only expert-verified but also regularly updated to ensure accuracy and relevance.
Learn more