Jump to a key chapter
Fundamentals of Nanocatalysis in Engineering
Nanocatalysis is a branch of catalysis that involves catalysts at the nanoscale. This field is gaining attention due to its ability to drastically enhance reaction rates by providing an increased surface area and tailored reactive sites. These catalysts play a crucial role in engineering applications.
What is Nanocatalysis?
Nanocatalysis refers to the use of nanoscale materials as catalysts to accelerate chemical reactions by offering a greater surface area-to-volume ratio than bulk materials. This allows for increased interaction with reactants, leading to improved efficiency.
For instance, when a platinum nanoparticle is used as a catalyst, the reaction rate in processes like hydrogenation can be significantly enhanced compared to bulk platinum.
Role of Surface Area in Nanocatalysis
The increased surface area of nanocatalysts is a critical factor that contributes to higher catalytic activity. At the nanoscale, the number of active sites per unit area increases, allowing for more effective interactions with reactants. This principle can be understood through the formula for surface area to volume ratio: \[ \text{SA:V} = \frac{A}{V} \] As the size decreases, the surface area to volume ratio increases, thereby enhancing the catalyst's performance.
The effects of surface area on reactivity make nanoparticles unique compared to their bulk counterparts.
Applications in Engineering
Nanocatalysts have a wide range of applications in engineering, including:
- Chemical Synthesis: Accelerating reactions to produce chemicals more efficiently.
- Environmental Engineering: Aiding in pollution control and waste treatment by breaking down pollutants.
- Energy Conversion: Used in fuel cells and batteries to improve energy efficiency.
Challenges and Considerations
While nanocatalysis offers numerous advantages, there are challenges that engineers must address, such as:
- Stability: Nanocatalysts may agglomerate, reducing their effectiveness.
- Scalability: Producing nanocatalysts on a large scale can be challenging.
- Safety: Potential environmental and health impacts need careful assessment.
Nanocatalysis Synthesis and Applications
In the field of nanotechnology, nanocatalysis involves the creation of catalysts with nanometer size dimensions. This section will cover various techniques used in the synthesis of nanocatalysts and provide examples of their practical applications in real-world scenarios.
Techniques in Nanocatalysis Synthesis
Nanocatalyst synthesis employs a range of methods, each with its unique mechanism and advantages. Here are some prevalent techniques:
- Sol-Gel Method: Involves transitioning sol into gel to create nanoparticles, known for its control over particle size and homogeneity.
- Hydrothermal Synthesis: Utilizes high pressure and temperature in aqueous solutions. Suitable for creating well-crystallized nanocatalysts.
- Chemical Vapor Deposition (CVD): Deposits reactants on substrates to form thin films or coatings, used for high purity nanoparticle production.
- Biogenic Synthesis: Involves biological materials like plant extracts as reducing agents, providing an eco-friendly approach.
The sol-gel method is particularly favored for producing uniform and high surface area nanocatalysts.
A deeper look into the sol-gel method reveals its versatility. This technique allows control over the pore structure and composition of the catalyst. It's frequently used for materials like silica and titania due to its ability to produce materials with special chemical and thermal stability.
Examples of Nanocatalysis in Real-World Scenarios
Nanocatalysis finds extensive use across various industries due to its efficiency and environmental benefits. Here are some noteworthy examples:
- Automotive Industry: Catalytic converters employ nanocatalysts to transform harmful gases like CO and NOx into less harmful substances.
- Energy Sector: Fuel cells use platinum nanocatalysts to enhance the conversion of chemical energy into electrical energy, offering clean energy solutions.
- Pharmaceuticals: Nanocatalysts are involved in the asymmetric synthesis of complex organic molecules, improving drug production efficiency.
- Environmental Remediation: In water treatment, nanocatalysts help break down pollutants, providing greener and more effective solutions.
An example in the energy sector is the use of titania-supported gold nanoparticles as catalysts in solar energy harvesting, enhancing the solar-to-electrical energy conversion efficiency.
Heterogeneous Nanocatalysis for Energy and Environmental Sustainability
Heterogeneous nanocatalysis plays a vital role in bridging the gap between cutting-edge scientific research and practical engineering applications. This section will explore how these catalysts contribute to sustainable energy solutions and environmental conservation.
Role of Heterogeneous Nanocatalysis
A heterogeneous catalyst differs from homogeneous catalysts in that it exists in a different phase than the reactants, often as a solid interacting with liquid or gaseous reactants.
In heterogeneous nanocatalysis, the stability and reusability of catalysts are key advantages. These catalysts enhance reactions by providing surface sites where reactants can adsorb and convert.Current applications include:
- Hydrogen Production: Nanocatalysts are used to break down water molecules for hydrogen fuel.
- Biomass Conversion: Transforming biomass into fuels using nano-scale catalysts increases energy yield.
- Pollutant Reduction: Helps in breaking down harmful emissions in industrial processes.
Heterogeneous nanocatalysts often require less energy to facilitate reactions compared to traditional catalysts.
In the field of hydrogen production, the use of nano-structured catalysts like platinum and palladium can significantly lower activation energy barriers. For example, the reaction: \[2H_2O(l) \rightarrow 2H_2(g) + O_2(g)\]requires efficient catalysis at reduced temperatures, which heterogeneous nanocatalysts can facilitate brilliantly.
Environmental Benefits of Nanocatalysis
Nanocatalysis offers substantial environmental benefits by enhancing the efficiency and sustainability of chemical processes. Here are some of the primary benefits:
- Waste Reduction: Increases selectivity of reactions, minimizing by-products.
- Energy Efficiency: Lowers the energy requirements of chemical reactions thereby reducing energy consumption.
- Pollution Control: Catalyzes the breakdown of pollutants such as nitrogen oxides and sulfur compounds.
An example of environmental benefit is the use of titanium dioxide nanocatalysts in water purification. They facilitate the breakdown of organic pollutants via photocatalytic processes.
Due to their reduced size, nanocatalysts can access pollutants more effectively in contaminated environments, providing a more thorough cleaning process.
Advanced Techniques in Nanocatalysis
The advancement of techniques in nanocatalysis has broadened the scope and application of catalysts at the nanoscale. These techniques are primarily focused on optimizing the size, shape, and composition of nanoparticles to enhance their catalytic properties.
Characterization Techniques
Characterization of nanoparticles is essential to understand their physical and chemical properties. Some of the advanced characterization techniques include:
- Electron Microscopy: Provides high-resolution images to analyze nanoparticle morphology and size distribution.
- X-ray Diffraction (XRD): Used to determine the crystallographic structure, phase, and crystallite size of the nanocatalysts.
- Dynamic Light Scattering (DLS): Measures particle size distribution by analyzing the scattering of light after passing through the nanoparticles.
- BET Surface Area Analysis: Utilizes gas adsorption to determine the surface area of nanoparticles, critical for catalytic activity.
For example, using electron microscopy, researchers can analyze the surface imperfections on a catalyst, which are critical for catalytic activity. Identifying these imperfections allows for the modification of nanoparticles to maximize reaction efficiency.
An intriguing aspect of X-ray Diffraction (XRD) is its ability to reveal the crystal structure of nanoparticles. By studying the diffraction patterns, the exact arrangement of atoms within the crystal lattice can be determined, which influences the electronic properties critical for catalytic processes. For instance, peaks in the diffraction pattern can be mathematically described by Bragg's Law: \[ n\lambda = 2d\sin\theta \] where \( n \) is an integer, \( \lambda \) is the wavelength, \( d \) is the distance between crystal planes, and \( \theta \) is the angle of incidence.
Synthesis Control Methods
Controlling the synthesis of nanocatalysts is critical to achieving the desired size and shape, which directly impacts their effectiveness. Some methods include:
- Liquid-Phase Synthesis: Involves the reduction or decomposition of precursors in solution, providing control over nanoparticle size and morphology.
- Template-Assisted Synthesis: Employs templates to guide the formation of nanoparticles into specific shapes and structures.
- High-Temperature Synthesis: Utilizes elevated temperatures to promote homogeneous nucleation and growth of nanoparticles.
- Electrochemical Reduction: Involves electrodeposition to generate nanoparticles, offering precise control over composition and structure.
Optimizing the shape of nanoparticles can significantly alter their catalytic properties, such as enhancing specific surface area and active sites exposure.
In the electrochemical reduction method, nanoparticles such as silver or gold can be precisely deposited on electrodes, forming specific patterns that amplify their catalytic capabilities, especially useful in electrocatalysis for applications in sensors and batteries.
nanocatalysis - Key takeaways
- Definition of Nanocatalysis: Utilizes nanoscale materials as catalysts to speed up chemical reactions by increasing surface area-to-volume ratio, enhancing efficiency.
- Surface Area's Impact: Increased surface area at the nanoscale boosts catalytic activity by providing more active sites for reactant interaction.
- Synthesis Techniques: Includes sol-gel, hydrothermal synthesis, CVD, and biogenic synthesis to control nanocatalyst properties for specific applications.
- Examples in Application: Utilized in automotive catalytic converters, fuel cells, pharmaceutical synthesis, and environmental remediation.
- Heterogeneous Nanocatalysis: Crucial for energy (e.g., hydrogen production) and environmental sustainability by providing stable, reusable catalysts.
- Advanced Characterization Techniques: Involves methods like electron microscopy, XRD, DLS, and BET analysis to profile nanocatalysts for optimized performance.
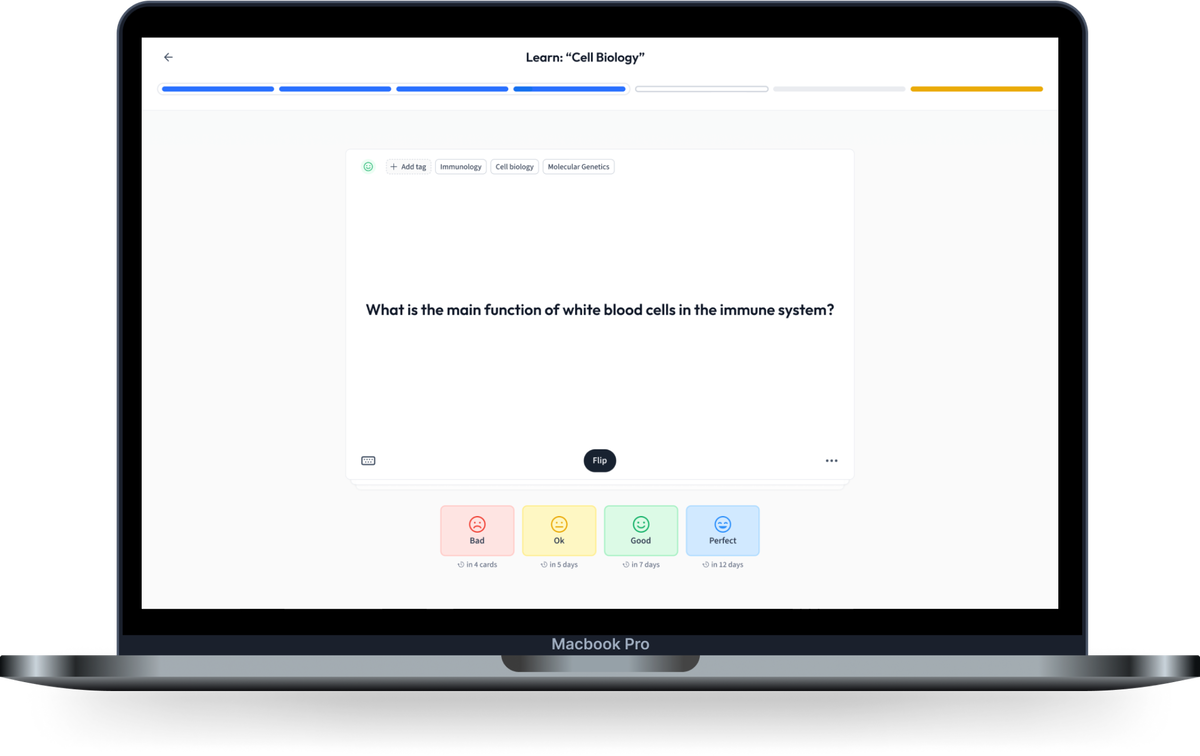
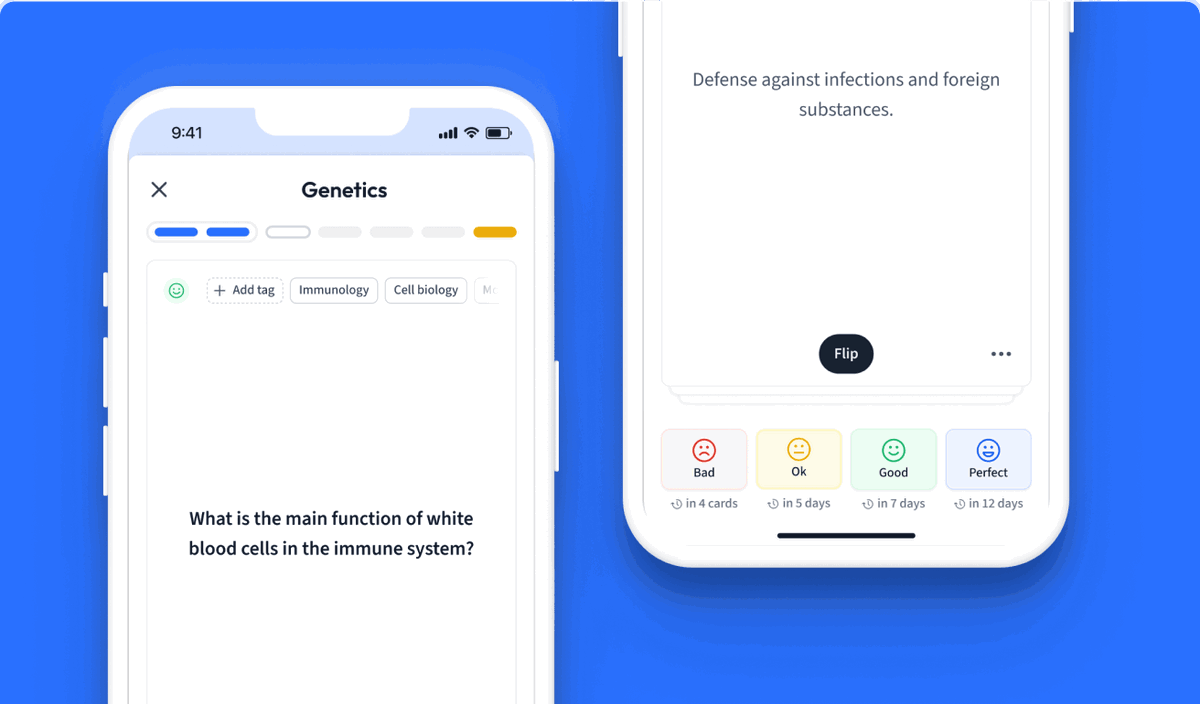
Learn with 12 nanocatalysis flashcards in the free StudySmarter app
Already have an account? Log in
Frequently Asked Questions about nanocatalysis
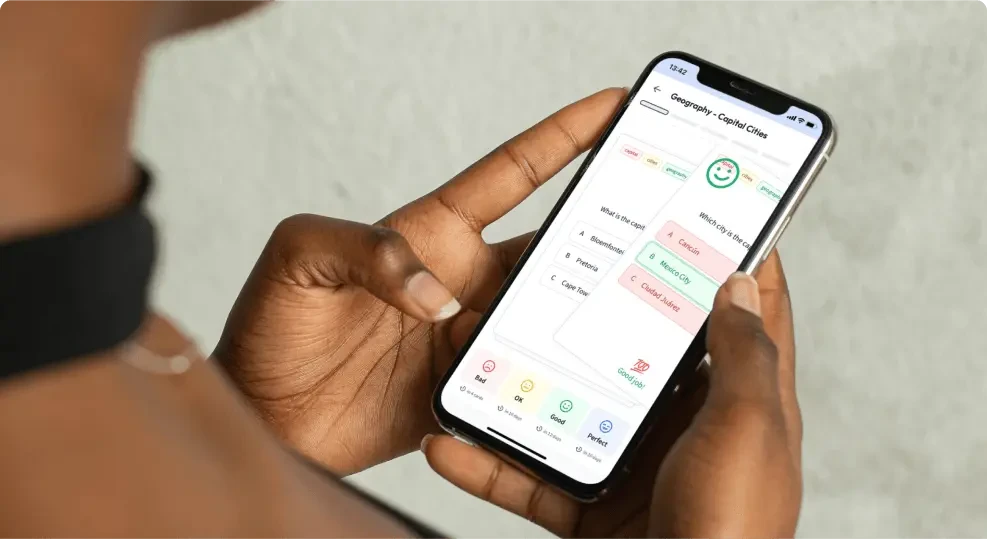
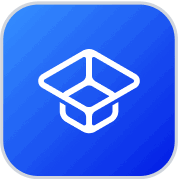
About StudySmarter
StudySmarter is a globally recognized educational technology company, offering a holistic learning platform designed for students of all ages and educational levels. Our platform provides learning support for a wide range of subjects, including STEM, Social Sciences, and Languages and also helps students to successfully master various tests and exams worldwide, such as GCSE, A Level, SAT, ACT, Abitur, and more. We offer an extensive library of learning materials, including interactive flashcards, comprehensive textbook solutions, and detailed explanations. The cutting-edge technology and tools we provide help students create their own learning materials. StudySmarter’s content is not only expert-verified but also regularly updated to ensure accuracy and relevance.
Learn more