Jump to a key chapter
What is Non-Equilibrium Thermodynamics?
Non-Equilibrium Thermodynamics is a branch of thermodynamics focused on systems that are not in thermodynamic equilibrium. This framework helps in understanding processes where traditional equilibrium assumptions fail.Instead of conserving energy as seen in equilibrium states, non-equilibrium thermodynamics involves energy transfer and transformations that do not obey equilibrium conditions. It finds applications in chemistry, physics, biology, and engineering.
Fundamental Concepts
In non-equilibrium thermodynamics, you'll encounter several fundamental concepts:
- Irreversible Processes: These processes occur when systems deviate from equilibrium, like chemical reactions and heat transfer.
- Entropy Production: A measure of irreversibility, often denoted as \[\frac{dS}{dt} > 0\], showing that entropy increases.
- Fluxes and Forces: Defined by the linear phenomenological laws, where flux is proportional to its driving force.
- Onsager's Reciprocal Relations: These relate cross-effects in thermodynamic fluxes, indicating symmetry in response.
Entropy Production is central to non-equilibrium thermodynamics. It represents the generation of entropy in an irreversible process.
Consider a simple heat conduction problem where heat flows from a hot to a cold reservoir. The heat flow is an irreversible process due to the temperature difference, and the entropy production \[\frac{dS}{dt}\] indicates this process's irreversibility. This exemplifies how non-equilibrium systems inherently generate entropy to reach equilibrium.
Mathematical Framework
Non-equilibrium thermodynamics employs mathematical models to express dynamics in systems:
- Phenomenological Coefficients: Relate thermodynamic fluxes \[(J_i)\] to forces \[(X_i)\] through the linear relation \[(J_i = \text{L}_{ij}X_j)\]. These coefficients \[(\text{L}_{ij})\] are determined experimentally.
- Balance Equations: Unlike equilibrium states, these equations consider time-dependent changes, for example, the balance equation for energy may be written as \[\frac{\text{d}U}{\text{d}t} = Q - W\], indicating energy change over time.
While Onsager's relations are grounded in linear response theory, their application extends far beyond. For instance, in biological systems, metabolic pathways demonstrate non-equilibrium states where these relationships help model the energy flow and biochemical reactions. Further, they are crucial in electrochemical reactions, often used in fuel cells, where non-equilibrium thermodynamics assist in optimizing energy efficiency.
Applications in Engineering
Non-equilibrium thermodynamics is influential in advancing technology across various sectors;
- Chemical Engineering: Optimizing reaction rates and selectivities under non-equilibrium conditions to improve yields.
- Mechanical Engineering: Analysis of thermal systems and heat exchangers to enhance energy transfer processes.
- Material Science: Understanding phase transitions and diffusion processes that are inherently non-equilibrial.
- Environmental Engineering: Modeling pollutant transport and climate systems, focusing on non-linear dynamics and energy balances.
Non-equilibrium thermodynamics is crucial not just in scientific fields but in practical problem solving across engineering disciplines, providing insights that cannot be garnered from equilibrium states alone.
Difference Between Equilibrium and Non-Equilibrium Thermodynamics
Thermodynamics plays a crucial role in understanding how systems exchange energy and matter. A key differentiator in the study of thermodynamics is whether a system is in equilibrium or non-equilibrium. Here, the focus is on exploring the contrasting aspects between these two states.
Equilibrium Thermodynamics
In equilibrium thermodynamics, systems are in a state where macroscopic properties like pressure, temperature, and composition do not change over time. Key characteristics include:
- Reversible Processes: Idealized processes that occur without any entropy production, denoted as \( \, \frac{dS}{dt} = 0 \, \).
- Thermal Equilibrium: No temperature gradients exist, making temperature uniform throughout the system.
- Phase Equilibrium: Phases, like liquid and gas, are stable and do not undergo transformation at equilibrium.
An equilibrium state is a condition in which a system's properties do not change with time, meaning it is at rest or processes are perfectly balanced.
Non-Equilibrium Thermodynamics
Conversely, non-equilibrium thermodynamics deals with systems that are in states of flux. Characteristics include:
- Irreversible Processes: Processes that generate entropy \((\frac{dS}{dt} > 0)\) due to non-zero driving forces.
- Spatial and Temporal Gradients: Non-uniform properties, such as temperature and concentration, throughout the system.
- Reaction Kinetics: Analyzes how chemical reactions progress in non-equilibrium conditions.
Imagine a cup of hot coffee left on a table. Initially, the coffee is at a higher temperature than the surrounding air. Over time, the coffee cools down because heat flows to the surrounding air—a classic non-equilibrium process. During this process, the entropy of the coffee-air system increases, and eventually, thermal equilibrium is achieved.
Non-equilibrium thermodynamics also plays a vital role in understanding natural processes, such as the Earth's climate system. The atmosphere and oceans present complex interactions that involve energy transfer, often deviating from equilibrium. By modeling these systems using non-equilibrium principles, it is possible to gain insight into climate change, predicting weather patterns, and understanding ecological impacts.
Non-equilibrium states can often be observed in everyday life, such as the process of an ice cube melting in a warm room, showcasing ongoing change until equilibrium is reached.
Understanding Non-Equilibrium Thermodynamics
Understanding Non-Equilibrium Thermodynamics involves exploring how systems evolve when they are not in a state of thermodynamic equilibrium. This field expands on classical thermodynamics principles to address energy and matter transfer processes that occur far from equilibrium.
Entropy Production in Non-Equilibrium Thermodynamics
In non-equilibrium thermodynamics, entropy production is a critical concept that signifies the „disorder“ generated within a system as it evolves. Entropy production is primarily associated with irreversible processes like heat conduction and chemical reactions.
Entropy production measures the dissipation of energy due to non-equilibrium processes and is expressed as \[ \frac{dS_{gen}}{dt} > 0 \], indicating that entropy increases over time.
Consider a solid object at a high temperature placed in a cooler environment. The heat flow from the object to the environment is an irreversible process, characterized by the entropy production within the system \( (\Delta S = \frac{Q}{T}) \). This process exemplifies how spontaneous heat exchanges between bodies at different temperatures generate entropy.
Entropy production is not just confined to thermal processes but is also applicable in a variety of fields, including engineering and biology. In living organisms, metabolic pathways illustrate intricate networks where chemical reactions far from equilibrium produce entropy. These insights help in quantifying energy efficiency and losses in biological systems, as well as in industrial processes, contributing to the development of sustainable technologies.
Remember, the greater the entropy production, the further a system is from equilibrium.
Key Concepts of Non-Equilibrium Thermodynamics for Engineers
Engineers frequently utilize principles from non-equilibrium thermodynamics to innovate and improve technology. Here are some key concepts:
- Flux-Force Relations: These emphasize the reliance of mass, heat, and electrical fluxes on their respective driving forces, often represented by gradients.
- Onsager's Reciprocal Relations: Indicate the interdependence and symmetry of molecular interactions in non-equilibrium states.
- Linear and Non-Linear Regimes: In linear regimes, simple proportional relations like \( J_i = L_{ij}X_j \) apply, while non-linear regimes demand more complex approaches.
A practical engineering application is the design of heat exchangers, wherein the configuration follows non-equilibrium principles to maximize thermal efficiency. Considering flux-force relations, the system is engineered to maintain optimal gradients that drive heat exchange, ensuring energy recovery and efficiency.
Applications of non-equilibrium thermodynamics extend into the realm of renewable energy technologies. In solar panels, for example, understanding how photons are absorbed and converted to electricity outside equilibrium allows for innovations in energy conversion efficiency. Similarly, in batteries, particularly those using novel chemistries like lithium-air, non-equilibrium processes are essential in optimizing charge and discharge cycles.
Using non-equilibrium models, engineers can predict and control material behavior under dynamic conditions, a crucial step towards sustainable industrial practices.
Applications of Non-Equilibrium Thermodynamics in Engineering
The field of non-equilibrium thermodynamics provides invaluable insights into numerous engineering applications. Understanding the behavior of systems away from equilibrium can significantly enhance the efficiency and performance of engineering processes. Below, we will explore real-world examples and the benefits this branch of thermodynamics brings to engineering design.
Real-World Examples of Non-Equilibrium Thermodynamics in Engineering
Non-equilibrium thermodynamics is applied in various engineering fields:
- Chemical Engineering: The optimization of reactor designs, particularly in processes like polymerization and combustion.
- Mechanical Engineering: Enhancing heat transfer systems, such as heat exchangers and cooling systems, by focusing on maximizing thermodynamic efficiency under dynamic conditions.
- Environmental Engineering: Addressing issues like pollutant dispersion in atmosphere and water bodies using non-equilibrium models for accurate predictions.
- Biomedical Engineering: Designing systems for drug delivery that rely on non-equilibrium principles to ensure precise dosing and release.
In engineering, an irreversible process is a process that cannot be reversed without leaving changes in the system or surroundings, usually characterized by entropy production.
Consider a catalytic converter in a vehicle exhaust system. Non-equilibrium thermodynamics is applied to design converters that efficiently reduce emissions by examining the diffusion and reaction rates of gases across catalyst surfaces.
A fascinating application of non-equilibrium thermodynamics is in the field of nanotechnology. Engineers use these principles to manipulate heat and mass transfer at the nanoscale, which is essential in fabricating new materials with unique properties. For example, by understanding and harnessing non-equilibrium processes, engineers can design nanoparticles for efficient thermal management in electronic devices, potentially revolutionizing cooling technologies.
Benefits of Using Non-Equilibrium Thermodynamics in Engineering Design
Applying non-equilibrium thermodynamics in engineering design results in several advantages:
- Increased Efficiency: Systems can be optimized to operate more efficiently by understanding non-equilibrium process dynamics.
- Enhanced Predictability: Non-equilibrium models help predict how systems behave under different conditions.
- Innovative Solutions: Enables the development of novel technologies, particularly in renewable energy sectors.
- Sustainability: Promotes environmentally sustainable practices by minimizing energy losses and optimizing resource usage.
In the design of gas turbines, non-equilibrium thermodynamics is used to model combustion processes. By optimizing these processes, engineers can significantly increase the turbine's thermal efficiency, leading to more energy output with less fuel consumption.
By leveraging non-equilibrium principles, engineers can improve the reliability and lifespan of systems, reducing maintenance costs and downtime.
non-equilibrium thermodynamics - Key takeaways
- Non-Equilibrium Thermodynamics: Study of systems not in thermodynamic equilibrium, involving energy transfers that deviate from equilibrium conditions. Used in chemistry, physics, biology, and engineering.
- Entropy Production: Indicates irreversibility in non-equilibrium processes with entropy increasing over time (\( \frac{dS}{dt} > 0 \)).
- Difference Between Equilibrium and Non-Equilibrium: Equilibrium systems have no net change in macroscopic properties, while non-equilibrium involves irreversible processes and entropy production.
- Applications in Engineering: Includes optimizing reaction rates in chemical engineering, enhancing heat transfer in mechanical engineering, and understanding pollutant transport in environmental engineering.
- Key Concepts for Engineers: Flux-force relationships, Onsager's Reciprocal Relations, and distinguishing between linear and non-linear regimes in designing systems.
- Mathematical Framework: Uses phenomenological coefficients and balance equations to model non-equilibrium systems, aiding in the understanding of complex processes.
Learn faster with the 12 flashcards about non-equilibrium thermodynamics
Sign up for free to gain access to all our flashcards.
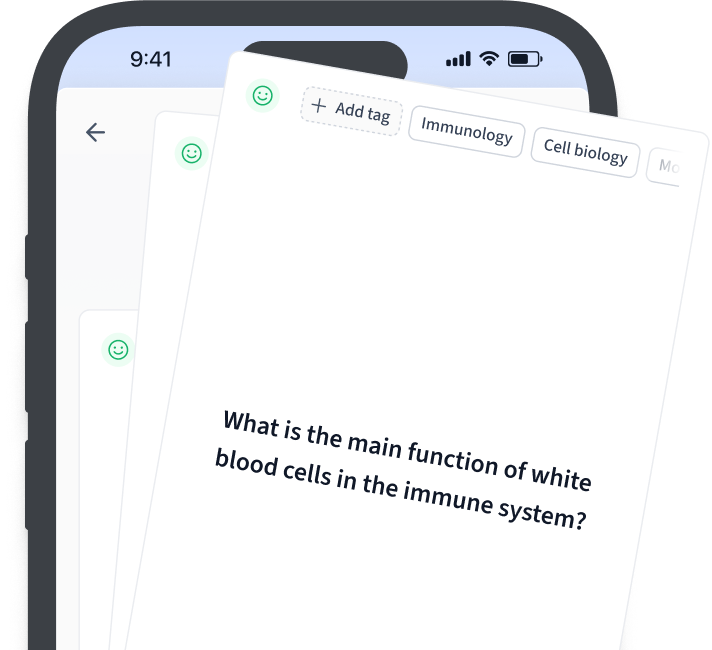
Frequently Asked Questions about non-equilibrium thermodynamics
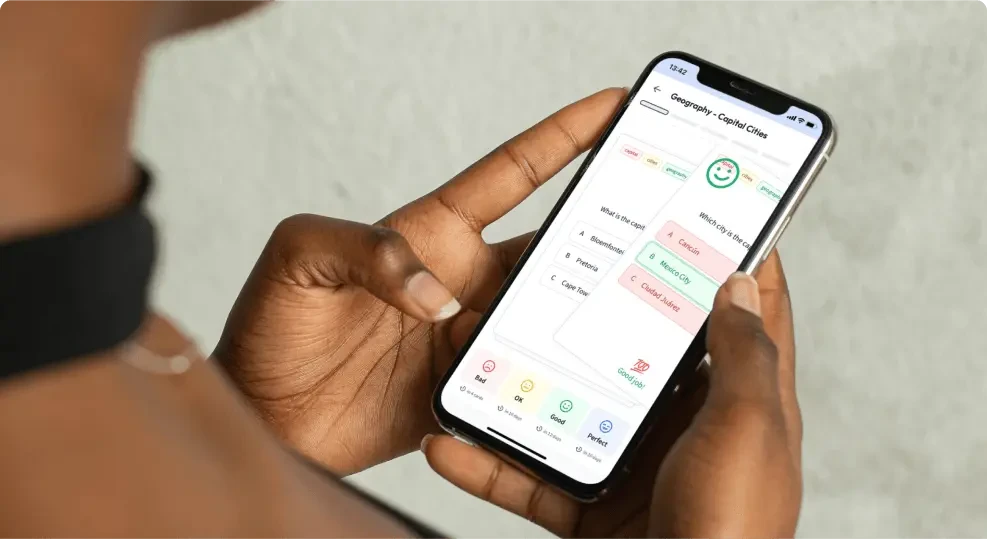
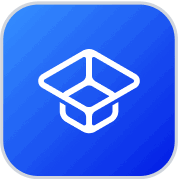
About StudySmarter
StudySmarter is a globally recognized educational technology company, offering a holistic learning platform designed for students of all ages and educational levels. Our platform provides learning support for a wide range of subjects, including STEM, Social Sciences, and Languages and also helps students to successfully master various tests and exams worldwide, such as GCSE, A Level, SAT, ACT, Abitur, and more. We offer an extensive library of learning materials, including interactive flashcards, comprehensive textbook solutions, and detailed explanations. The cutting-edge technology and tools we provide help students create their own learning materials. StudySmarter’s content is not only expert-verified but also regularly updated to ensure accuracy and relevance.
Learn more