Jump to a key chapter
Definition of Polymer Catalysis
Polymer catalysis involves the use of polymers as catalysts to accelerate chemical reactions. This intersection of polymer science and catalysis is an innovative area within engineering, focusing on enhancing reaction processes using polymer-based materials. The complexity of polymers offers opportunities to fine-tune catalytic properties for specific applications.
What is Polymer Catalysis?
Polymer catalysis refers to the use of polymers as catalysts in chemical reactions. These polymers can be either synthetic or natural and are designed to facilitate reactions by lowering the activation energy. Unlike conventional catalysts, polymer catalysts can be engineered to have unique characteristics, such as tailored pore sizes or functional groups.Polymer catalysts can be viewed as a hybrid between homogeneous and heterogeneous catalysts. They often offer the advantages of both types:
- Control over reaction environment: Due to their structure, polymer catalysts can provide a controlled microenvironment for chemical reactions.
- Reusability: Similar to heterogeneous catalysts, many polymer catalysts can be reused, making them economically viable.
- Versatility: Polymers can be designed with an array of chemical functionalities, allowing them to participate in different types of reactions.
Polymer catalysts are especially useful in industries where reusability and reaction specificity are vital.
Detailed Explanation of Polymer Catalysis
The study of polymer catalysis focuses on a few key principles that make it distinct from traditional catalysts. Here are some of the fundamental aspects:
- Polymer Support: In many cases, catalysts are anchored onto polymer backbones, providing a large surface area and stabilizing the catalytic site.
- Functional Group Modification: Through chemical modification, polymers can be equipped with specific functional groups, enabling targeted catalysis.
- Swelling Properties: Some polymer catalysts exhibit swelling in specific solvents, allowing substrates to diffuse and react more efficiently.
Reaction Type | Usage Examples |
Acid-Base Catalysis | Solid polymer acids for proton transfer reactions. |
Redox Reactions | Polymers with metallic nanoparticles for oxidation and reduction. | Biotransformations | Biopolymer catalysts for enzyme-like due to their functional mimicry. |
Example: An example of polymer catalysis in action is the use of polystyrene sulfonic acid as a catalyst for esterification reactions. This polymer catalyst is reusable and provides high reaction specificity without needing harsh reaction conditions.
The introduction of smart polymers into the field of catalysis presents new opportunities. Smart polymers, which respond to external stimuli such as temperature or pH, are being researched for applications where reaction conditions can be dynamically controlled. This adaptability is particularly useful in biological systems where in situ changes can occur.Additionally, advancements in nanotechnology have allowed for the development of nanostructured polymer catalysts, enhancing their efficiency and selectivity. For instance, the incorporation of nanoparticles into polymer matrices has led to significant improvements in catalytic activity and reaction rates.As research progresses, the synergy between polymer science and catalysis continues to grow, offering new possibilities for industrial applications and sustainable processes.
Mechanisms of Polymer Catalysis
Polymer catalysis encompasses various mechanisms through which polymers aid in accelerating chemical reactions. Understanding these mechanisms is essential for employing polymer catalysts effectively in different chemical processes. Below, we explore some of the key mechanisms and molecular processes involved in polymer catalysis.
Key Mechanisms in Polymer Catalysis
Polymer catalysts function through several distinct mechanisms. These include:
- Surface Catalysis: Here, reactions occur on the surface of the polymer. The polymer's surface may provide active sites where substrates can bind and undergo chemical transformation.
- Encapsulation: Polymers can encapsulate catalytic species, creating a microenvironment that facilitates reaction. This mechanism is akin to the action of enzymes in biological systems.
- Pore Diffusion: In porous polymers, reactants diffuse into the polymer matrix where the catalytic sites reside, enhancing reaction rates.
Polymer support mechanisms can adjust the physical environment around a reaction, enhancing control over reaction rate and selectivity.
Molecular Processes Involved
At the molecular level, polymer catalysis involves intricacies that contribute to its efficacy. Some important processes include:
- Electronic Interactions: Polymers can interact electronically with catalytically active sites, stabilizing intermediates and transition states of a reaction.
- Hydrophobic Effects: The hydrophobic properties of polymers can help concentrate organic substrates, improving reaction rates in aqueous solutions.
- Dynamic Structure: Polymers can adapt their conformation in response to environmental changes, affecting their catalytic properties.
Consider a polymer catalyst that is used for a reduction reaction involving hydrogen gas. The polymer's porous structure allows hydrogen molecules to diffuse into the polymer, where they interact with catalytic sites, facilitating the conversion of a substrate into a desired product.
Research into the molecular processes of polymer catalysis has unveiled insights into the synergistic effect. This effect occurs when multiple functional groups within the polymer work together, resulting in enhanced catalytic activity. For instance, certain block copolymers have been engineered to exhibit dual catalytic functionalities, addressing both oxidation and reduction processes concurrently.Furthermore, smart polymer systems that respond to stimuli, such as temperature and pH, are being increasingly investigated. These systems offer potential for catalytic applications that require precise control over reaction conditions. Incorporating stimuli-responsive moieties into a polymer matrix can enable these materials to act differently under varying external conditions, making them viable for use in complex environments, such as living organisms or dynamic industrial processes.
Techniques in Polymer Catalysis
In the field of polymer catalysis, several techniques are utilized to harness polymers' unique properties for improving chemical processes. These techniques range from traditional methods that effectively incorporate polymers into reactions, to novel approaches that push the boundaries of catalytic applications. Understanding these techniques is crucial for employing polymers effectively in a variety of industrial and research settings.
Common Techniques in Polymer Catalysis
Numerous techniques have been developed and refined over time to utilize polymers in catalysis. Some of the most prevalent methods include:
- Supported Polymer Catalysts: In this technique, polymers act as a support matrix for catalytic metals or active species, enhancing stability and reusability.
- Micellar Catalysis: Utilizes the ability of polymers to form micelles, which can increase the solubility of reactants and improve reaction rates.
- Cross-linked Polymer Catalysts: These are typically insoluble polymers with a three-dimensional network, providing a high surface area and robust stability for catalytic reactions.
Example: An example of a common technique is using poly(acrylic acid) as a catalyst support in the polymerization of vinyl chloride, where the polymer's functional groups aid in stabilizing the active species.
Micellar catalysis is particularly advantageous in aqueous reactions, as it leverages polymers' ability to self-assemble in water.
Cutting-Edge Tools and Methods
Research in polymer catalysis is continually evolving, leading to the development of cutting-edge tools and methods that expand the capabilities of polymer catalysts. Some of these advanced techniques include:
- Response-Driven Catalysis: Involves smart polymers that change their catalytic activity in response to environmental stimuli like temperature or pH.
- Hybrid Nanostructured Catalysts: Combines polymers with nanomaterials to enhance activity and specificity, providing new pathways for catalysis.
- Click Chemistry in Polymer Synthesis: Utilizes click reactions to precisely control polymer architecture, enhancing catalytic performance.
One of the most exciting developments in polymer catalysis is the use of stimuli-responsive polymers. These smart materials adapt to changes in their environment, such as light, magnetic fields, or biological signals, offering dynamic control over catalytic activity.Additionally, the integration of data science and machine learning aids in the optimization of polymer catalysts. By analyzing vast datasets on reaction parameters and conditions, researchers can now predict and design catalysts with enhanced efficiency and selectivity.The combination of traditional chemical techniques with modern computational tools points towards a future where polymer catalysis can lead to breakthroughs in sustainable chemistry and green technologies, minimizing waste and reducing environmental impact.
Applications of Polymer Catalysis in Engineering
Polymer catalysis has emerged as a transformative element in engineering, offering novel solutions and efficiencies in various sectors. By leveraging the unique properties of polymers, industries are able to innovate and improve processes significantly. The applications extend across a spectrum of fields, showcasing both traditional and cutting-edge techniques.
Industrial Applications of Polymer Catalysis
Polymer catalysis plays a critical role in a variety of industrial applications, enhancing efficiency and sustainability. Here are some key areas:
- Petrochemical Industry: Polymer catalysts are employed in cracking processes to break down large hydrocarbon chains, improving fuel production efficiency.
- Pharmaceutical Manufacturing: They enable more precise and selective synthesis of complex molecules, critical in drug development.
- Environmental Engineering: Used in pollution control processes, such as catalytic converters which reduce emissions from vehicles.
Polymer Catalysis: A mechanism in which polymers act as catalysts in chemical reactions, offering efficiencies in various industrial processes.
In the pharmaceutical industry, using polymer catalysts can significantly reduce the number of steps required in synthesizing complex drugs.
Examples of Polymer Catalysis Reactions in Real-World Scenarios
To understand the practical impact of polymer catalysis, consider these real-world scenarios where they have been pivotal:
- Polylactic Acid (PLA) Production: Used in hydrophobic catalysts to synthesize biodegradable plastics as a sustainable alternative to traditional plastics.
- Water Treatment: Polymer-based catalysts facilitate the removal of contaminants through advanced oxidation processes, making water reuse more feasible.
- Fine Chemical Synthesis: Employed in the production of fine chemicals where selectivity and purity are crucial.
An example of polymer catalysis in action is the use of cross-linked polystyrene as an acid catalyst in esterification reactions. This allows for the production of esters, which are essential in fragrances and flavorings.
A remarkable application of polymer catalysis is in the field of green chemistry. By using renewable polymer sources and eco-friendly catalytic processes, industries can significantly reduce their carbon footprint. For example, the development of biopolymers tailored as enzymatic mimics offers renewable pathways for biodegradable materials.Additionally, the integration of catalysts in 3D printing materials has opened new doors for rapid prototyping and manufacturing, where polymer catalysts enhance the mechanical and thermal properties of printed products.
polymer catalysis - Key takeaways
- Definition of Polymer Catalysis: Use of polymers as catalysts to accelerate chemical reactions, combining polymer science and catalysis.
- Mechanisms of Polymer Catalysis: Includes surface catalysis, encapsulation, and pore diffusion, providing a controlled microenvironment.
- Techniques in Polymer Catalysis: Involves supported polymer catalysts, micellar catalysis, and cross-linked polymer catalysts for industrial use.
- Applications in Engineering: Used in petrochemical industry, pharmaceutical manufacturing, and environmental engineering for efficiency and sustainability.
- Examples of Polymer Catalysis Reactions: Polystyrene sulfonic acid for esterification; PLA production and water treatment as sustainable applications.
- Detailed Explanation: Focus on polymer support, functional group modification, and swelling properties for versatile reaction catalysis.
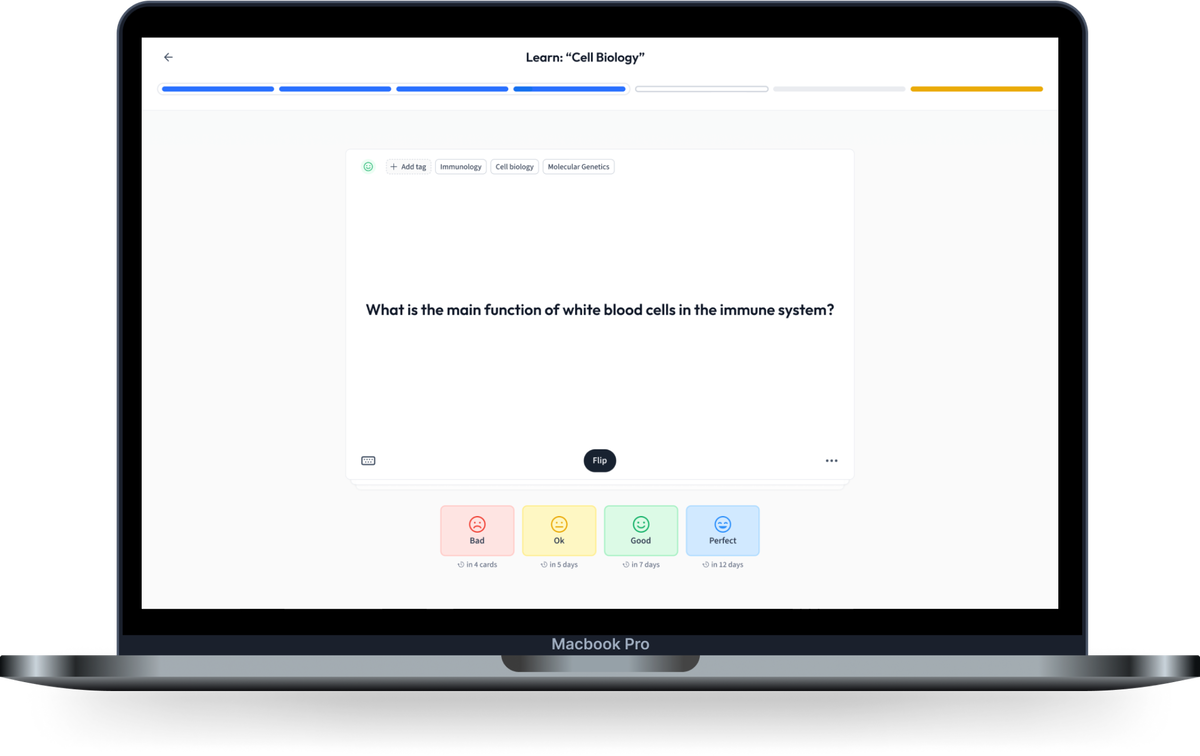
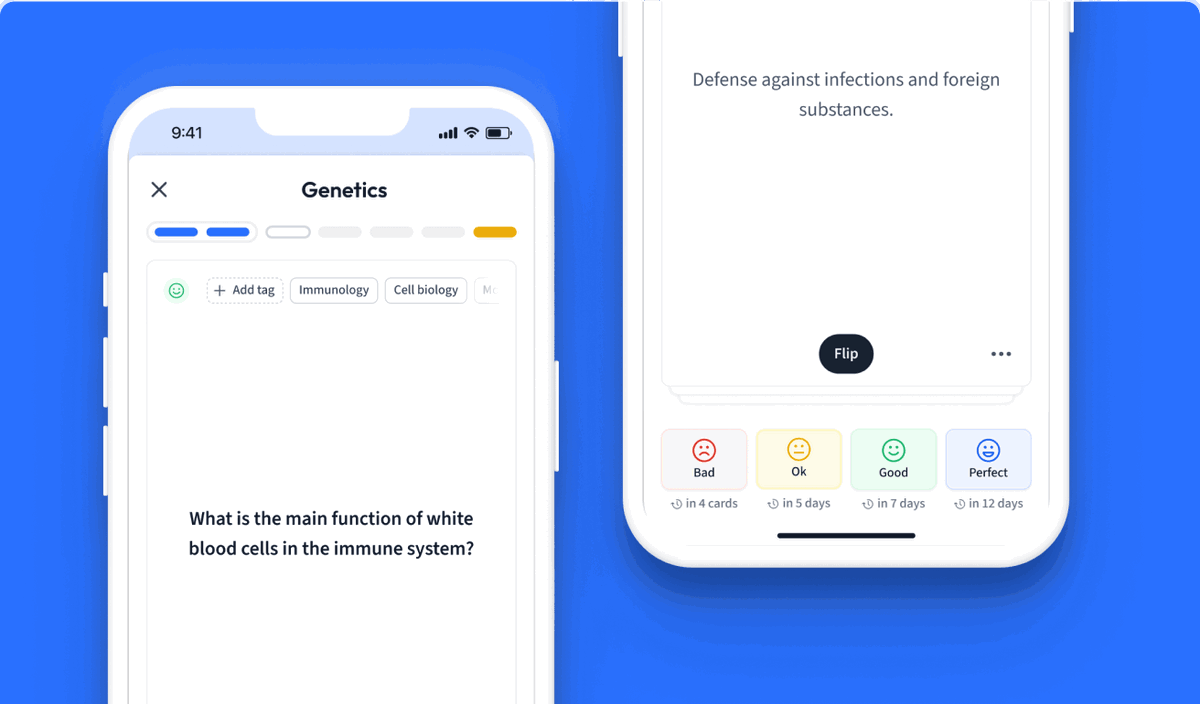
Learn with 12 polymer catalysis flashcards in the free StudySmarter app
We have 14,000 flashcards about Dynamic Landscapes.
Already have an account? Log in
Frequently Asked Questions about polymer catalysis
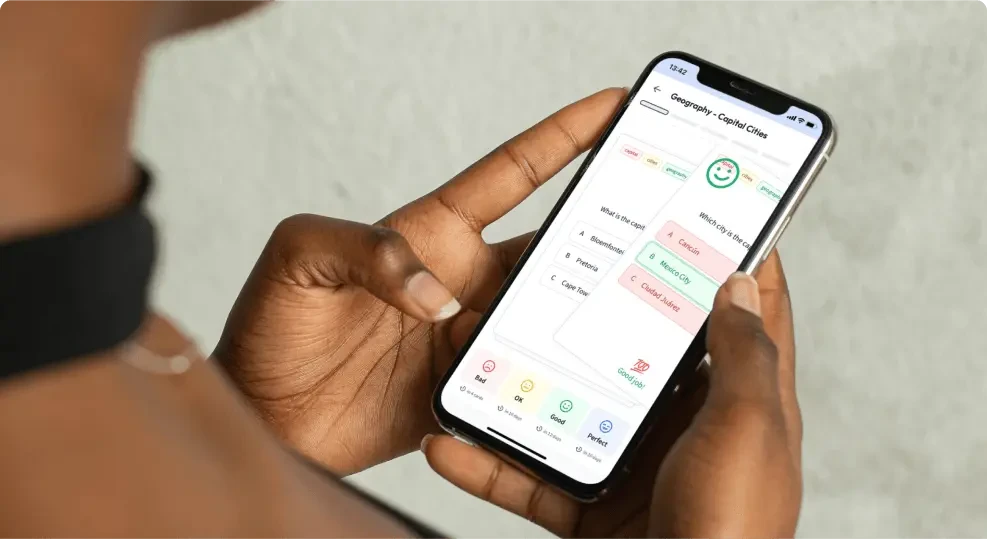
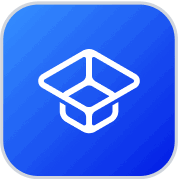
About StudySmarter
StudySmarter is a globally recognized educational technology company, offering a holistic learning platform designed for students of all ages and educational levels. Our platform provides learning support for a wide range of subjects, including STEM, Social Sciences, and Languages and also helps students to successfully master various tests and exams worldwide, such as GCSE, A Level, SAT, ACT, Abitur, and more. We offer an extensive library of learning materials, including interactive flashcards, comprehensive textbook solutions, and detailed explanations. The cutting-edge technology and tools we provide help students create their own learning materials. StudySmarter’s content is not only expert-verified but also regularly updated to ensure accuracy and relevance.
Learn more