Jump to a key chapter
Polymer Electrolytes Explained
Polymer electrolytes are fascinating materials that combine the properties of polymers and electrolytes. They are widely used in various applications, including batteries, fuel cells, and sensors. In this section, you will learn about their unique characteristics, underlying chemistry, and practical uses.
Basic Concepts of Polymer Electrolytes
Polymer electrolytes are solid or semi-solid materials made by dissolving a salt in a polymer matrix. This matrix provides the mobility required for ionic conduction. A polymer electrolyte typically consists of:
- Polymer Matrix: Polymers such as polyethylene oxide (PEO) offer the structural framework.
- Conducting Ions: Result from the dissociation of a dissolved salt.
- Plasticizers: Optional additives that improve ionic conductivity by lowering the glass transition temperature.
The combination allows ions to move freely, making them key to the functionality of many electrochemical devices.
Polymer Electrolytes: Materials that conduct ions through a polymer-based medium. They exhibit both solid-like and liquid-like electrolyte properties used in various technological applications.
One common example of a polymer electrolyte is a PEO-LiTFSI blend, where PEO is the polymer and LiTFSI stands for lithium bis(trifluoromethanesulfonyl)imide. This combination is frequently used in lithium-ion batteries.
Polymer electrolytes can be either solid or gel-like, depending on their composition and intended use.
Mathematical Modeling of Ionic Conductivity
The ionic conductivity of polymer electrolytes is determined by the movement of ions through the polymer matrix. It is affected by several factors such as temperature, ion concentration, and the chemical nature of the polymer. To model this, the following equation is often used:
The ionic conductivity \( \sigma \ \) is given by:
\[ \sigma = n \cdot q \cdot \mu \]
\( \sigma \) | Ionic conductivity (S/cm) |
\( n \) | Number density of charge carriers (ions/cm3) |
\( q \) | Charge of the carriers (Coulombs) |
\( \mu \) | Mobility of the carriers (cm2/V·s) |
This formula illustrates the dependency of conductivity on ion concentration and mobility, which varies with temperature and polymer flexibility.
Advancements in polymer electrolytes focus on enhancing conductivity while maintaining mechanical stability. Research into block copolymers, polymer blends, and nano-composite electrolytes is ongoing. Block copolymers, for instance, can self-assemble into nanoscale morphologies, optimizing pathways for ion transport. They offer a promising route to enhance ionic transport without sacrificing structural integrity.
Solid Polymer Electrolyte
Solid polymer electrolytes expand the scope of polymer electrolytes by being entirely solid at room temperature while still effectively conducting ions. They are crucial in enhancing the performance and safety of batteries and other electrochemical devices. Let’s delve into the characteristics and functions of solid polymer electrolytes below.
Characteristics of Solid Polymer Electrolytes
Solid polymer electrolytes are distinguished by their unique blend of properties, which include:
- Mechanical Stability: They provide structural support to devices, preventing leaks and minimizing shorts.
- Ionic Conductivity: Conductivity that allows ions to migrate through the solid state.
- Thermal Stability: Capability of withstanding a wide range of temperatures, crucial for various applications.
Solid Polymer Electrolytes: Electrolytes formed from solid polymer matrices that maintain ionic conduction without requiring a liquid medium. They are integral to the advancement of solid-state technology.
Working Principle and Ion Transport
The operation of solid polymer electrolytes relies on their ability to facilitate ion transport through the polymer matrix. This movement is governed by mechanisms such as:
- Ion Dissolution: Ions dissociate from their salts within the polymer matrix.
- Segmental Motion: Movement of polymer chains which helps in ion transport.
\[ \sigma = \frac{{q^2 n D}}{{kT}} \]
\( \sigma \) | Ionic conductivity |
\( q \) | Charge of an ion |
\( n \) | Ion number density |
\( D \) | Diffusion coefficient |
\( k \) | Boltzmann constant |
\( T \) | Temperature (Kelvin) |
An example of a solid polymer electrolyte is the polymer electrolyte membranes used in lithium-ion batteries. The Li-ion conducting polymer often incorporates lithium perchlorate within a polyethylene oxide base, showcasing excellent conductivity and mechanical stability.
Solid polymer electrolytes reduce the risk of leakage associated with liquid electrolytes, offering a safer alternative.
The development of single-ion conducting solid polymer electrolytes is a promising avenue in enhancing electrochemical stability. By immobilizing the anion component, these materials facilitate selective cation mobility, reducing polarization effects and enhancing overall battery performance. Research into alternative polymer backbones and the incorporation of nano-fillers aims to further improve mechanical properties and thermal stability while boosting ionic conduction.
Polymer Electrolyte Membrane and Its Applications
Polymer electrolyte membranes (PEMs) play a crucial role in modern electrochemical devices due to their unique ability to conduct protons while acting as insulators for electrons. They are instrumental in applications ranging from fuel cells to water electrolysis. Understanding their function helps in designing efficient energy systems.
Polymer Electrolyte Membrane Electrolysis
Polymer electrolyte membrane electrolysis is a process used to split water into hydrogen and oxygen by applying an electric current across a PEM-based electrolyzer. Here’s how it works:
- Electricity is applied, driving the electrolyte reaction.
- Water molecules at the anode are split into oxygen, protons, and electrons.
- The membrane allows protons to pass through to the cathode, while blocking electrons, which are forced to travel through an external circuit.
- At the cathode, protons and electrons recombine to form hydrogen gas.
Polymer Electrolyte Membrane (PEM): A semi-permeable membrane typically made from ionomer, which conducts protons and separates reactants in devices like fuel cells and electrolyzers.
A practical example of polymer electrolyte membrane electrolysis is its use in hydrogen production for fuel cell vehicles. In this setup, PEM electrolyzers are employed to produce clean hydrogen fuel, which is then used to power electric motors.
PEM electrolysis is particularly advantageous because it operates efficiently at low temperatures and has a compact design.
Recent advancements in polymer electrolyte membranes focus on enhancing durability and reducing costs. Innovations like the development of composite membranes that integrate nanoparticles to improve thermal and mechanical stability are being researched. Such enhancements not only prolong membrane life but also improve electrical efficiency.
The efficiency of PEM electrolysis can be quantified by examining the Faraday efficiency, which is expressed as:
\[ \eta_F = \frac{{z \cdot F \cdot n}}{{I \cdot t}} \]
\( \eta_F \) | Faraday efficiency |
\( z \) | Number of moles of electrons per mole of hydrogen |
\( F \) | Faraday's constant |
\( n \) | Amount of substance in moles |
\( I \) | Current in amperes |
\( t \) | Time in seconds |
Composite Polymer Electrolyte
Composite polymer electrolytes are an advanced class of materials that blend polymers with inorganic fillers or other additives to enhance their properties. These composites are gaining traction due to their improved mechanical strength, thermal stability, and ionic conductivity compared to conventional polymer electrolytes.
The integration of different materials allows composite polymer electrolytes to tailor their characteristics for specific applications, especially in high-performance batteries and fuel cells.
Properties and Benefits of Composite Polymer Electrolytes
Composite polymer electrolytes offer a range of enhanced properties:
- Improved Ionic Conductivity: The addition of fillers can create pathways that facilitate ion movement in the polymer matrix.
- Enhanced Mechanical Strength: Fillers such as nanoparticles reinforce the polymer, offering better structural support.
- Thermal and Chemical Stability: These composites can withstand higher temperatures and are more chemically robust.
These properties make composite polymer electrolytes suitable for next-generation electrochemical systems that require durability and high performance.
An example of a composite polymer electrolyte is the incorporation of silica nanoparticles into a polyethylene oxide-lithium salt matrix. This combination not only enhances ionic conductivity but also provides mechanical stability required for solid-state batteries.
Composite Polymer Electrolyte: A material system formed by integrating polymer electrolytes with inorganic or organic fillers to enhance performance characteristics.
One fascinating aspect of composite polymer electrolytes is how the particle size and distribution of fillers influence ionic conduction. By using nanoscale fillers, you can create a percolation network that enhances ion transport without significantly increasing the polymer matrix's weight or volume.
The effectiveness of ion transport in composite electrolytes often depends on the tortuosity and connectivity within the polymer-filler matrix. This can be modeled using:
\[ D_{eff} = D_0 \times \frac{1}{\tau} \]
\( D_{eff} \) | Effective diffusion coefficient |
\( D_0 \) | Intrinsic diffusion coefficient |
\( \tau \) | Tortuosity of the path |
Advantages of Polymer Electrolytes in Engineering
Polymer electrolytes bring a variety of advantages to the field of engineering, especially in developing energy storage and conversion technologies. Their unique properties open doors to innovations and improvements in efficiency, safety, and sustainability.
In this section, you'll explore how polymer electrolytes enhance engineering applications, particularly in batteries, fuel cells, and sensors.
Enhanced Safety in Batteries
Polymer electrolytes offer significant safety benefits over traditional liquid electrolytes:
- Reduced Risk of Leakage: As solid materials, they minimize the chance of spills and leakage, preventing potential hazards associated with corrosive liquids.
- Flame Resistance: Many polymer electrolytes are less flammable compared to liquid counterparts, reducing fire risks.
- Stable under Repeated Charging Cycles: They maintain their structural integrity over repeated charge and discharge cycles, enhancing battery longevity and reliability.
These properties make polymer electrolytes an excellent choice for safer, more reliable battery technologies.
An example where enhanced safety is crucial is in electric vehicles, where polymer electrolytes help prevent thermal runaway – a potentially dangerous chain reaction occurring due to overheating in lithium-ion batteries.
High Ionic Conductivity for Efficient Energy Devices
The efficiency of energy devices, like batteries and fuel cells, significantly improves with the high ionic conductivity of polymer electrolytes:
- Fast Ion Transport: Polymer electrolytes facilitate quicker ion movement, resulting in faster charge and discharge rates.
- Better Performance at Lower Temperatures: They provide significant performance benefits at lower operational temperatures, crucial for applications in cold environments.
Understanding the mathematics of ionic conductivity, the relationship is characterized by:
\[ \sigma = n \cdot q \cdot \mu \]
\( \sigma \) | Ionic conductivity |
\( n \) | Number of charge carriers |
\( q \) | Charge of each carrier |
\( \mu \) | Mobility of carriers |
This equation highlights how polymer electrolytes enhance energy storage devices' performance by maximizing these variables.
Polymer electrolytes are also being investigated for use in next-generation solid-state batteries, promising higher energy densities.
Flexibility and Lightweight Nature
The inherent flexibility and lightweight nature of polymer electrolytes provide significant advantages for engineering lightweight portable devices:
- Conformability: Their flexibility allows integration into flexible electronics and wearable devices.
- Reduction in Overall Weight: Contributing to less heavy device designs, crucial for mobile and portable applications.
Researchers are developing gel polymer electrolytes that combine the stability of solid substances with the flexibility typically seen in gels. These innovations can achieve conductivity levels approaching those of liquid electrolytes while retaining the benefits of polymer-based systems.
One additional advantage is their potential role in reducing environmental impact. By selecting biodegradable polymer materials and sustainable manufacturing processes, the ecological footprint of such electrolytes can be minimized, aligning with global sustainability goals and green engineering practices.
polymer electrolytes - Key takeaways
- Polymer Electrolytes: Materials that conduct ions through a polymer-based medium, exhibiting both solid-like and liquid-like properties for various applications like batteries and sensors.
- Solid Polymer Electrolyte: Electrolytes created from solid polymer matrices that conduct ions efficiently without a liquid medium. Key for enhancing battery safety and performance.
- Polymer Electrolyte Membrane (PEM): Semi-permeable membranes that conduct protons while separating reactants, used in fuel cells and electrolyzers.
- Composite Polymer Electrolyte: Materials integrating polymers with fillers like nanoparticles to improve mechanical strength, ionic conductivity, and thermal stability.
- Polymer Electrolyte Membrane Electrolysis: A process using a PEM-based electrolyzer to split water into hydrogen and oxygen, highlighting its role in sustainable energy systems.
- Advantages in Engineering: Include improved safety, high ionic conductivity, flexibility, and lightweight properties, making polymer electrolytes ideal for advanced energy devices.
Learn faster with the 10 flashcards about polymer electrolytes
Sign up for free to gain access to all our flashcards.
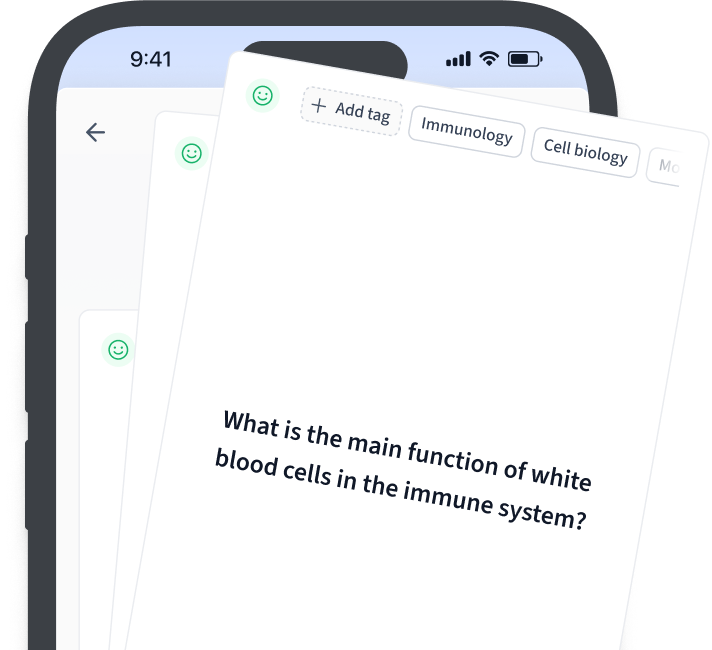
Frequently Asked Questions about polymer electrolytes
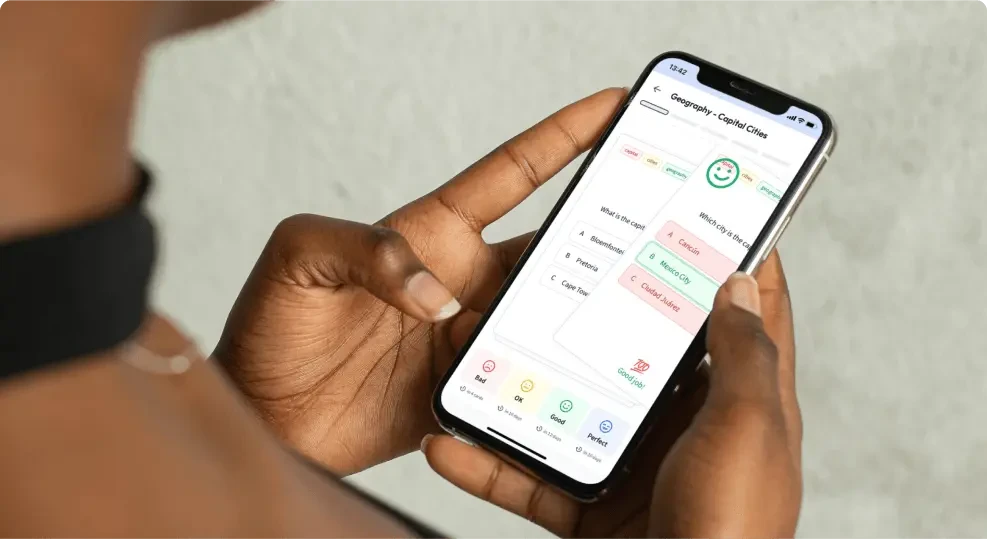
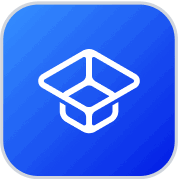
About StudySmarter
StudySmarter is a globally recognized educational technology company, offering a holistic learning platform designed for students of all ages and educational levels. Our platform provides learning support for a wide range of subjects, including STEM, Social Sciences, and Languages and also helps students to successfully master various tests and exams worldwide, such as GCSE, A Level, SAT, ACT, Abitur, and more. We offer an extensive library of learning materials, including interactive flashcards, comprehensive textbook solutions, and detailed explanations. The cutting-edge technology and tools we provide help students create their own learning materials. StudySmarter’s content is not only expert-verified but also regularly updated to ensure accuracy and relevance.
Learn more