Jump to a key chapter
Understanding Polymer Nanostructures: Fundamental Principles
Polymer nanostructures are an exciting field of study within materials science and engineering, offering promising applications across various industries. By manipulating polymers at the nanoscale, novel structures with unique properties are created, leading to breakthroughs in technology and manufacturing.
Definition and Basics of Polymer Nanostructures
Polymer Nanostructures: Structures made from polymers that are engineered at the nanometer scale to achieve specific properties.
At the nanoscale, materials exhibit different properties compared to their bulk counterparts. Polymer nanostructures take advantage of these unique features to enhance performance and functionality. These structures are typically measured in nanometers, where 1 nanometer equals one-billionth of a meter.
There are several key characteristics of polymer nanostructures:
- High surface area-to-volume ratio
- Customized chemical properties
- Enhanced mechanical strength
- Nano-scale optical and electronic behavior
The size of a polymer nanostructure is comparable to the width of a single strand of DNA.
Example of polymer nanostructures in application: Nanocomposites, used in automotive and aerospace industries, enhance material strength and reduce weight.
Formation Process of Polymer Nanostructures
The formation of polymer nanostructures relies on different techniques and processes that allow precise control over nanoscale features. Below are some common methods used in the creation of polymer nanostructures:
- Self-Assembly: Leveraging the natural tendency of molecules to organize into ordered structures. This process is driven by thermodynamic principles without external guidance.
- Nanoimprint Lithography: Employing molds to create nanoscale patterns on polymer surfaces. This technique is useful in fabricating uniform structures on a large scale.
- Electrospinning: A process where a polymer solution is spun into fibers with diameters ranging from tens to hundreds of nanometers.
The process of self-assembly is fascinating and involves a balance of entropic and enthalpic forces. During self-assembly, the system evolves towards a state that minimizes its free energy. This can be captured using the Gibbs free energy equation: \[\triangle G = \triangle H - T \triangle S\]where \(\triangle G\) represents the change in free energy, \(\triangle H\) is the change in enthalpy, \(\triangle S\) is the change in entropy, and \(\triangle T\) represents the temperature. These principles are crucial in guiding the formation of stable nanostructures, leading to potential innovations in material design.
Examples of Polymer Nanostructures in Engineering
Polymer nanostructures have been making a significant impact in the engineering field, leading to innovations and improvements in various applications. These structures are leveraged in diverse industries, from electronics to biomedical engineering, due to their unique properties conferred by the nanoscale manipulation of polymers.
Notable Case Studies in Engineering
Case studies in engineering provide concrete examples of how polymer nanostructures are applied to solve complex problems. Here are some noteworthy case studies:
- Nanostructured Coatings: Employed to enhance the durability and functionality of materials used in aerospace engineering. These coatings provide improved resistance to wear and environmental degradation.
- Water Filtration Systems: Utilizing polymer nanostructures to create advanced filtration membranes. These nanostructures remove contaminants at the molecular level, significantly improving water quality.
- Optoelectronic Devices: Implemented in the development of efficient solar cells. Polymer nanostructures help in maximizing light absorption and energy conversion efficiency.
Example of Polymer Nanostructures in Case Studies: A leading aerospace company developed a new aircraft wing material by incorporating polymer nanostructured coatings. This innovation resulted in a 20% increase in overall fuel efficiency due to reduced drag and improved material strength.
The field of water filtration has been revolutionized by polymer nanostructures. Traditional filtration systems often struggle to remove nanoscale pollutants. However, by using nanostructured polymer membranes, it becomes possible to filter these contaminants effectively. The membranes operate based on size exclusion, allowing water molecules to pass through while trapping larger, undesirable particles. This approach leads to higher purity levels in the filtered water and increased efficiency in the filtration process. Engineers continue to optimize these polymer nanostructures with innovative design tweaks to further enhance performance and longevity.
Innovative Uses in Modern Engineering
In modern engineering, polymer nanostructures are at the forefront of creating advanced materials with upgraded capabilities. These nanostructures push the boundaries of traditional engineering by enabling functionalities that were previously unattainable. Some of the innovative uses include:
- Biomedical Implants: Polymer nanostructures are used in implants to promote biocompatibility and enhance tissue regeneration.
- Self-Healing Materials: Engineered to repair themselves after damage, reducing maintenance costs and extending the lifespan of materials.
- Flexible Electronics: Innovations in polymer nanostructures have paved the way for bendable and stretchable electronic devices, improving durability and expanding their use in wearable technology.
Self-healing materials leverage the unique properties of polymer nanostructures, enabling them to autonomously repair minor damages, similar to how skin heals.
Mechanical Properties of Polymers Based on Nanostructure and Morphology
The mechanical properties of polymers are significantly influenced by their nanostructure and morphology. By engineering these properties at the nanoscale, polymers can be designed to meet specific requirements for various applications, ensuring they perform optimally under different conditions.
Analysis of Mechanical Properties
To understand how polymer nanostructures affect mechanical properties, it is crucial to consider various factors such as tensile strength, elasticity, and impact resistance. These allow engineers to tailor polymer materials to specific needs.For instance, \textbf{tensile strength} is affected by the molecular arrangement within the polymer, often enhanced through the strategic placement of nanostructures. Elasticity, on the other hand, benefits from the flexibility provided by the polymer's nanoscale architecture. A thorough mechanical analysis would typically involve mathematical modeling, such as:Ultrasonic testing methods evaluating Young's modulus can be mathematically expressed by:\[E = \frac{\text{stress}}{\text{strain}}\]where \(E\) denotes Young's modulus, illustrating the stiffness of a given polymer structure.
The elasticity of a polymer is directly proportional to its nanostructure's flexibility.
Example of Mechanical Property Analysis: A polymer used in flexible electronics was engineered with specific nanostructures that increased its tensile strength by 30%, allowing for greater durability and wear resistance over time.
In-depth analysis of polymer mechanics reveals fascinating interactions between nanostructures. For example, the incorporation of carbon nanotubes in polymer matrices significantly affects the modulus of elasticity. This is expressed in the rule of mixtures formula for composite materials:\[E_c = V_mE_m + V_fE_f\]where:
- \(E_c\) is the modulus of the composite.
- \(V_m\) and \(V_f\) are the volume fractions of the matrix and the fibers, respectively.
Effect of Nanostructure on the Properties of Polymers
The impact of nanoscale modifications on polymer properties is profound. Understanding how these nanostructures influence mechanical behaviors is key for developing advanced materials. Specific structural configurations at the nanoscale can affect:
- Toughness: Improved through cross-linking at nanoscale junctions between polymer chains.
- Thermal Stability: Enhanced by integrating heat-resistant nanostructures that help manage temperature variations.
- Surface Hydrophobicity: Altered by the arrangement of polymer nanostructures to repel water efficiently.These modifications adjust the polymer's functionalities, allowing designers to customize materials for a wide range of engineering applications. By utilizing appropriate mathematical models, these characteristics can be predicted and optimized for various usage scenarios.
Nanostructural adjustments can make polymers suitable for extreme environments, such as high-temperature applications.
The effect of nanostructure on polymer properties can also be quantified through simulations and molecular dynamics studies. These models often utilize quantum mechanics principles to predict material behavior. Molecular dynamics simulations involve calculating force fields and potential energy surfaces, expressed by solving Schrödinger's equation:\[H\Psi = E\Psi\]where \(H\) is the Hamiltonian operator, \(\Psi\) is the wave function, and \(E\) represents the energy levels. Such approaches provide insights into atoms' and molecules' movement within the polymer matrix, offering a comprehensive picture of how nanoscale morphology contributes to macroscale polymer properties.
Applications of Polymer Nanostructures in Biomedical Engineering
Polymer nanostructures have become crucial in the field of biomedical engineering due to their versatile properties and ability to interface seamlessly with biological systems. Their applications are diverse, ranging from drug delivery systems to tissue engineering, offering innovative solutions to longstanding medical challenges.
Polymer Nanostructures in Drug Delivery Systems
In drug delivery systems, polymer nanostructures serve as carriers to enhance the delivery and efficacy of therapeutic agents. They offer controlled release, targeted delivery, and enhanced solubility of drugs, improving treatment outcomes. These nanostructures can be engineered to respond to specific biological stimuli such as pH or temperature, allowing for precise drug release at the desired site.
Example of Drug Delivery System: A nanostructured polymer micelle was developed to deliver chemotherapy drugs directly to tumor cells, minimizing side effects and enhancing drug concentration at the cancer site.
Polymer nanostructures can improve the blood circulation time of drugs due to their stealth properties, avoiding recognition by the immune system.
In the context of drug delivery, the design of polymer nanostructures often involves amphiphilic block copolymers. These copolymers form self-assembled micelles in aqueous environments with hydrophobic cores and hydrophilic shells. This self-assembly provides a stable environment for hydrophobic drugs, enhancing their delivery potential. Furthermore, the surface chemistry of these structures can be modified with targeting ligands or polyethylene glycol (PEG) to improve biocompatibility and specificity. This strategic engineering allows for the development of personalized medicine approaches, where drug delivery systems are customized according to patient-specific needs.
Advancements in Tissue Engineering with Polymer Nanostructures
Polymer nanostructures are transforming the field of tissue engineering by providing scaffolding that can support cell growth and tissue regeneration. Their nanoscale properties allow for the mimicry of the extracellular matrix, promoting cell adhesion, proliferation, and differentiation. This is essential for developing functional tissues that can be used in regenerative medicine.
Example in Tissue Engineering: Researchers have created a biodegradable polymer nanostructured scaffold that aids in the repair of damaged cartilage tissue, showing promise for patients suffering from joint disorders.
Tissue Engineering: A field of regenerative medicine focused on developing biological substitutes to restore, maintain, or improve tissue function.
Polymer nanostructures can incorporate growth factors within their matrix to enhance tissue regeneration.
The development of polymer nanostructures in tissue engineering goes beyond traditional scaffolding techniques. Advanced approaches involve nano-biomimetic designs that leverage 3D printing and electrospinning technologies, allowing precise control over the scaffold's spatial architecture. This precision enables the creation of gradient structures that transition in composition and porosity, closely resembling the natural tissue environment. Moreover, the integration of bioactive molecules and nanoparticles into these scaffolds can provide additional functionalities, such as promoting vascularization or delivering antimicrobial agents. This interdisciplinary innovation is driving the future of successful tissue implants and regenerative therapies.
Dynamics Mechanics and Multi-Scale Modeling of Polymer Nanostructures
In the realm of polymer nanostructures, understanding the dynamics mechanics and multi-scale modeling are crucial for advancing materials science applications. These approaches provide insights into how polymer nanostructures behave under various conditions and facilitate the prediction and design of new materials with desired properties.
Techniques in Multi-Scale Modeling
Multi-scale modeling techniques are integral to deciphering the complexities of polymer nanostructures. These techniques bridge the gap between the nano and macroscale, enabling a detailed understanding of materials' behaviors and properties. Common techniques used in multi-scale modeling include:
- Atomistic Modeling: Involves simulating the interactions at the atomic level to predict molecular behaviors and properties.
- Coarse-Grained Modeling: Reduces the complexity of simulation by grouping atoms into larger units, balancing detail with computational efficiency.
- Continuum Modeling: Applies continuum mechanics principles for the entire material system, useful for studying large-scale behaviors.
Integrating multi-scale modeling with experimental data can greatly enhance accuracy and reliability. For example, quantum mechanics and molecular mechanics (QM/MM) methods allow accurate simulation of electronic behavior in polymers while employing fewer computational resources. This technique defines a hybrid potential energy surface based on:\[E_{total} = E_{QM} + E_{MM} - E_{\text{overlap}}\]where \(E_{total}\) is the total energy of the system, \(E_{QM}\) is the quantum mechanical energy, \(E_{MM}\) denotes the molecular mechanical energy, and \(E_{\text{overlap}}\) represents energy correction for overlapping regions. This nuanced approach allows researchers to accurately predict polymer properties, crucial for the design of next-generation materials.
Understanding Dynamics Mechanics in Polymer Nanostructures
The study of dynamics mechanics in polymer nanostructures provides a fundamental understanding of how these materials respond under various mechanical stresses and environmental conditions. This knowledge is essential for tailoring polymers for specific applications that require high performance and resilience. Key factors considered in dynamics mechanics include:
- Stress and Strain Analysis: Understanding how internal forces affect polymer deformation and strength is crucial. Stress-strain relationships guide the design of durable polymers.
- Viscoelastic Behavior: Describes the time-dependent strain a polymer experiences under constant stress, significant for applications requiring flexibility and damping.
- Thermomechanical Analysis: Evaluates polymer behavior with temperature changes, essential for applications in varying thermal environments.
Example of Dynamics Mechanics in Action: A polymer nanostructure is engineered for use in aerospace components. By analyzing stress-strain data, researchers adjusted the polymer's composition to enhance its fatigue resistance, prolonging its lifespan under cyclic loading conditions.
polymer nanostructures - Key takeaways
- Polymer Nanostructures: Structures engineered from polymers on the nanometer scale to achieve specific properties.
- Fundamental Principles: Includes techniques like self-assembly, nanoimprint lithography, and electrospinning for creating precise polymer nanostructures.
- Mechanical Properties: Influenced by nanostructures; includes tensile strength, elasticity, and impact resistance.
- Engineering Applications: Used in nanocomposites, coatings, filtration systems, and optoelectronics for enhanced material properties.
- Biomedical Applications: Include drug delivery systems, tissue engineering scaffolds with enhanced biocompatibility and functionality.
- Dynamics and Modeling: Multi-scale modeling techniques (atomistic, coarse-grained, continuum) for predicting properties and designing advanced materials.
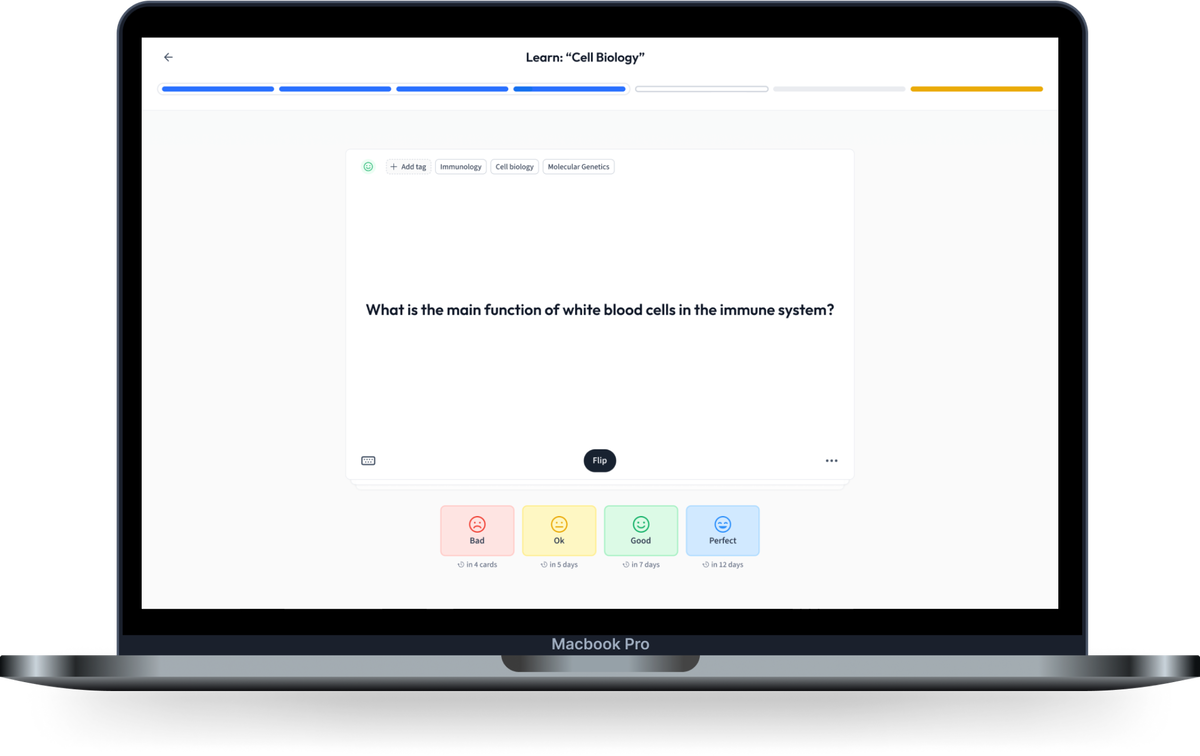
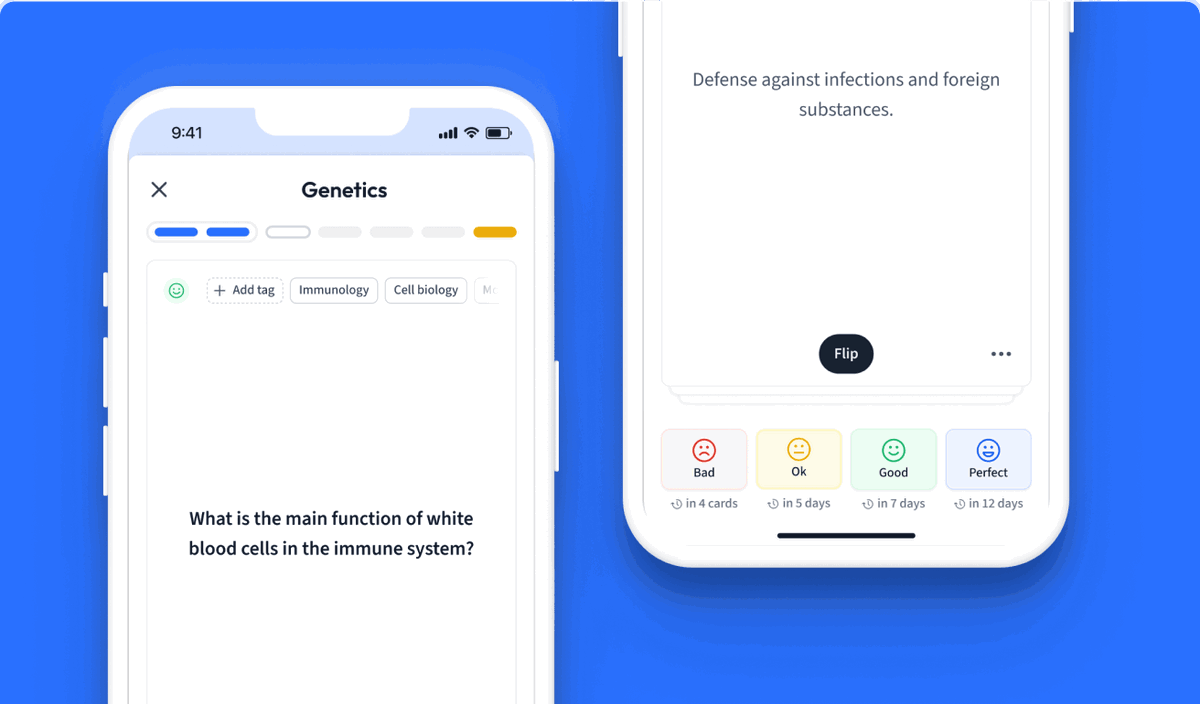
Learn with 10 polymer nanostructures flashcards in the free StudySmarter app
Already have an account? Log in
Frequently Asked Questions about polymer nanostructures
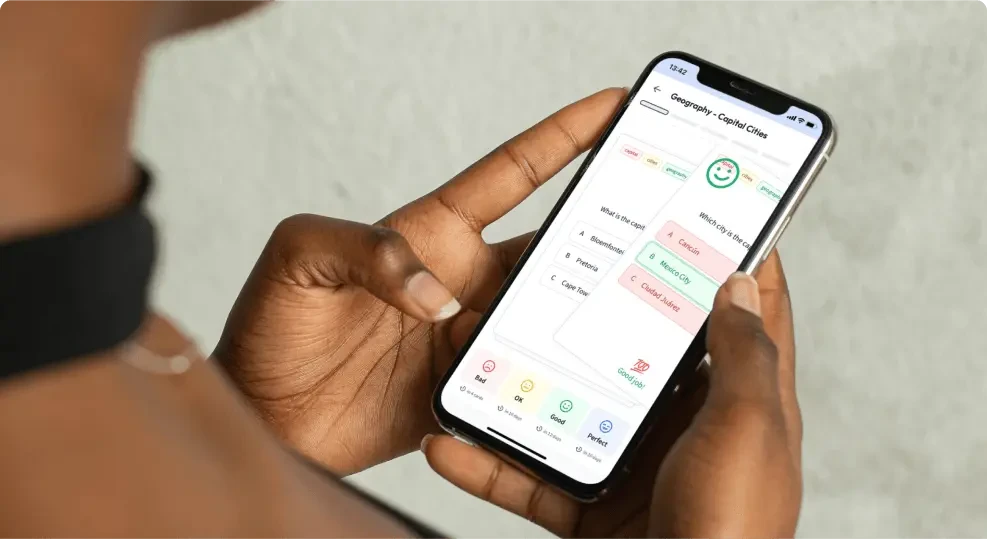
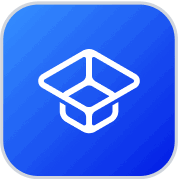
About StudySmarter
StudySmarter is a globally recognized educational technology company, offering a holistic learning platform designed for students of all ages and educational levels. Our platform provides learning support for a wide range of subjects, including STEM, Social Sciences, and Languages and also helps students to successfully master various tests and exams worldwide, such as GCSE, A Level, SAT, ACT, Abitur, and more. We offer an extensive library of learning materials, including interactive flashcards, comprehensive textbook solutions, and detailed explanations. The cutting-edge technology and tools we provide help students create their own learning materials. StudySmarter’s content is not only expert-verified but also regularly updated to ensure accuracy and relevance.
Learn more