Jump to a key chapter
Reaction Control Definition in Engineering
Reaction control is a significant concept in the field of engineering, particularly in areas like aerospace and mechanical engineering. This term often relates to the methods and systems used to manage and direct the movement or attitude of a vehicle or object. By controlling the reaction forces, engineers can achieve precise navigation and stability.
Understanding Reaction Control
In engineering, reaction control systems (RCS) are crucial for adapting and managing movement in various environments. Be it space vehicles, aircraft, or underwater technology, RCS come into play to adjust orientation and ensure stability. They are responsible for delivering thrust without necessarily moving the main engines, making them essential for fine-tuned adjustments and controlled movements.
- Allows for spatial orientation adjustments
- Important for small, precise maneuvers
- Utilizes small thrusters or directional jets
The Reaction Control System (RCS) refers to the group of thrusters on a spacecraft or vehicle that manage its orientation and position by expelling mass to create a reactive force in accordance with Newton's third law of motion.
A classic example of reaction control can be seen in spacecraft like the Space Shuttle. On its journey through space, it requires precisely calculated thrust from its RCS to turn, spin, and stabilize while outside the Earth's atmosphere. Without an atmosphere to provide aerodynamic forces, the RCS is vital for ensuring the spacecraft's desired trajectory.
Reaction control isn't limited to space exploration. Consider underwater ROVs (Remote Operated Vehicles) working in deep ocean environments. These vehicles rely on reaction control systems to navigate turbulent currents and maintain their positions as they perform tasks like sampling or repairs. Engineers use RCS in these contexts to ensure that tools and cameras are aimed accurately and movements are steady. The same principles apply for automated drones, which utilize reaction control systems to adjust for wind and pressure changes, allowing them to hover or move with precision over distances.
Thermodynamic Versus Kinetic Reaction Control
When exploring reaction control in engineering, two critical aspects are often discussed: thermodynamic control and kinetic control. These concepts are distinct yet intertwined in determining the outcomes of various chemical and physical processes.
- Thermodynamic Control: This involves processes where the final product is determined by the most stable (lowest energy) state.
- Kinetic Control: Here, the pathway with the fastest rate (even if not the most stable) determines the final product.
Thermodynamic control refers to the scenario in which the reaction reaches a state of minimum energy, leading to the most stable product.
Kinetic control focuses on reactions that yield products rapidly due to lower activation energy, despite not reaching the lowest energy state.
Mathematical Representation of Kinetic and Thermodynamic Control
To understand these concepts mathematically, you can examine the relationship between activation energy and the Gibbs free energy. For thermodynamic control, a common equation used is:
\[ \text{Gibbs Free Energy Change} (\text{ΔG}) = \text{ΔH} - T\text{ΔS}\]Where:
- \tΔH: Enthalpy change
- \tT: Temperature
- \tΔS: Entropy change
For kinetic control, the Arrhenius equation is often used:
\[ k = Ae^{-Ea/RT} \]Where:
- \tk: Reaction rate constant
- \tA: Pre-exponential factor
- \tE_a: Activation energy
- \tR: Universal gas constant
- \tT: Temperature in Kelvin
Consider a simple reaction where a molecule can transform into two different products: one is more stable, and the other is formed faster. If the reaction is conducted at low temperatures, the product formed with kinetic control typically prevails, as the activation energy requirement side-steps the stability consideration.
Conversely, at higher temperatures, reactions under thermodynamic control drive the system toward the more stable product, aligning with lower energy states.
Many catalytic processes aim to maintain both kinetic and thermodynamic controls to optimize yield and efficiency.
In real-world applications, like in petrochemical industries, understanding the balance between kinetic and thermodynamic controls is vital. Refining processes often adjust temperature and pressure to tip the scales towards desired pathways. For instance, cracking heavier hydrocarbons to gasoline relies on balancing these controls to maximize output, while minimizing unwanted byproducts.
Temperature plays a crucial role; raising it can speed up reactions (kinetic control) but can also allow more stable products to dominate over time (thermodynamic control). This dynamic demonstrates the necessity for fine-tuning in both laboratory and industrial settings.
Thermodynamically Controlled Reaction
In the realm of chemical reactions, thermodynamically controlled reactions are governed by the stability of the final product rather than the speed at which it is formed. This principle is particularly important in systems where the reaction conditions allow the reactants to reach equilibrium, leading to the most stable product being favored.
In these reactions, the product that forms is often the one with the lowest Gibbs free energy, indicating maximum stability.
Pathway | Control Type | Product Stability |
Faster Pathway | Kinetic Control | Less Stable |
Equilibrium Pathway | Thermodynamic Control | More Stable |
A thermodynamically controlled reaction is one in which the final product is determined by the most stable state, typically achieved by allowing the reaction mixture to reach equilibrium.
Examples of Thermodynamically Controlled Reactions
Understanding examples of thermodynamically controlled reactions can bring clarity to this concept. Consider the conversion of graphite to diamond. Although energetically this is favorable, the process is slow due to kinetic barriers. However, given enough time and the right conditions, the diamond will eventually form as it represents the more stable arrangement of carbon atoms under high pressure.
- Graphite to Diamond: The diamond is the thermodynamically stable form at high pressures.
- Enol to Keto form: In tautomeric shifts, the keto form is generally more stable and preferred in equilibrium.
Consider the esterification of acetic acid with ethanol to form ethyl acetate. In this reaction, the formation of ethyl acetate is thermodynamically controlled. Given time for equilibrium, most acetic acid molecules convert to the more stable ester product, especially under catalytic conditions where equilibrium is established efficiently.
In reactions where two or more products are possible, thermodynamic control means the dominant product is the one with the lowest energy.
Moving beyond simple reactions, in organic chemistry, the thermodynamic control is often applied in designing synthetic pathways. By manipulating reaction conditions such as temperature and pressure, chemists aim to favor the formation of preferred products. For example, the reversible formation of cyclic and linear molecules can be affected by temperature changes, where high temperatures might favor the thermodynamically stable product by overcoming potential energy barriers.
This understanding extends to material science, where thermodynamically controlled processes are critical in creating materials with optimal properties. This includes the production of stable alloys where the constituent elements are chosen based on expected stability under operating conditions. By applying principles of thermodynamic control, scientists can predict and harness the conditions most likely to yield desired outcomes.
Kinetic Control Reaction
In chemical engineering, the concept of kinetic control refers to reactions where the final product formation is dictated by the reaction pathway that has the fastest rate of transformation. Unlike thermodynamically controlled processes, kinetic control prioritizes the speed of achieving a product even if this doesn't represent the lowest energy outcome.
- Occurs at lower temperatures
- Involves higher activation barriers
- Results in rapid, less stable product formation
Kinetic control in a reaction refers to the dominance of the product pathway that involves the lowest activation energy, even if other more stable products are possible.
Consider a simple (A + B) system where two possible products C and D can be formed. Under kinetic control conditions, if product C has a lower activation energy barrier than product D, C will form quicker regardless of D potentially being more stable.
- Low temperature favors: Faster pathways
- High temperature allows: More stable products to form
reaction control - Key takeaways
- Reaction control in engineering involves managing and directing the movement or attitude of vehicles using systems like reaction control systems (RCS).
- Reaction control systems are crucial for adjusting orientation and ensuring stability without moving the main engines, often used in spacecraft, aircraft, and underwater vehicles.
- Thermodynamic control of a reaction results in the formation of the most stable product at equilibrium, determined by the lowest Gibbs free energy.
- Kinetic control reaction refers to the situation where the reaction pathway with the fastest rate of transformation determines the product, often occurring at lower temperatures.
- A thermodynamically controlled reaction is one where the end product is the most stable, achieved by allowing the reaction mixture to reach equilibrium.
- Kinetic and thermodynamic control of reactions are distinguished by the rate of product formation and stability, with kinetic favoring speed and thermodynamic favoring stable products.
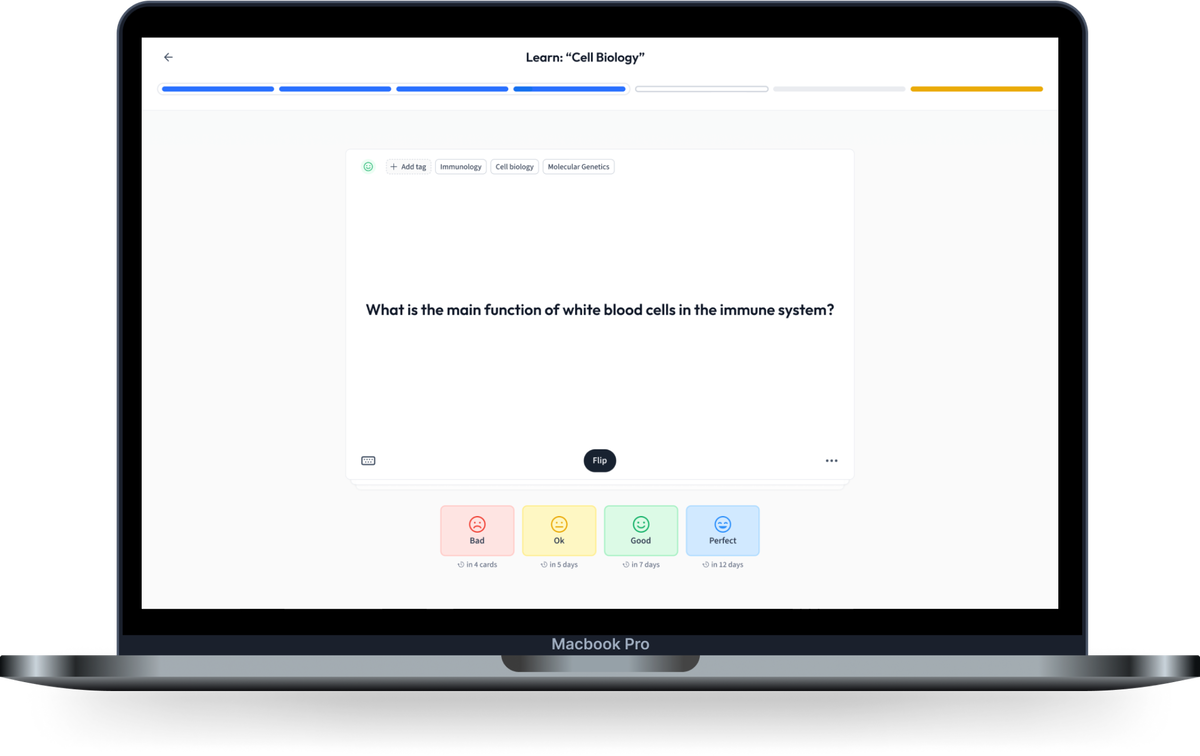
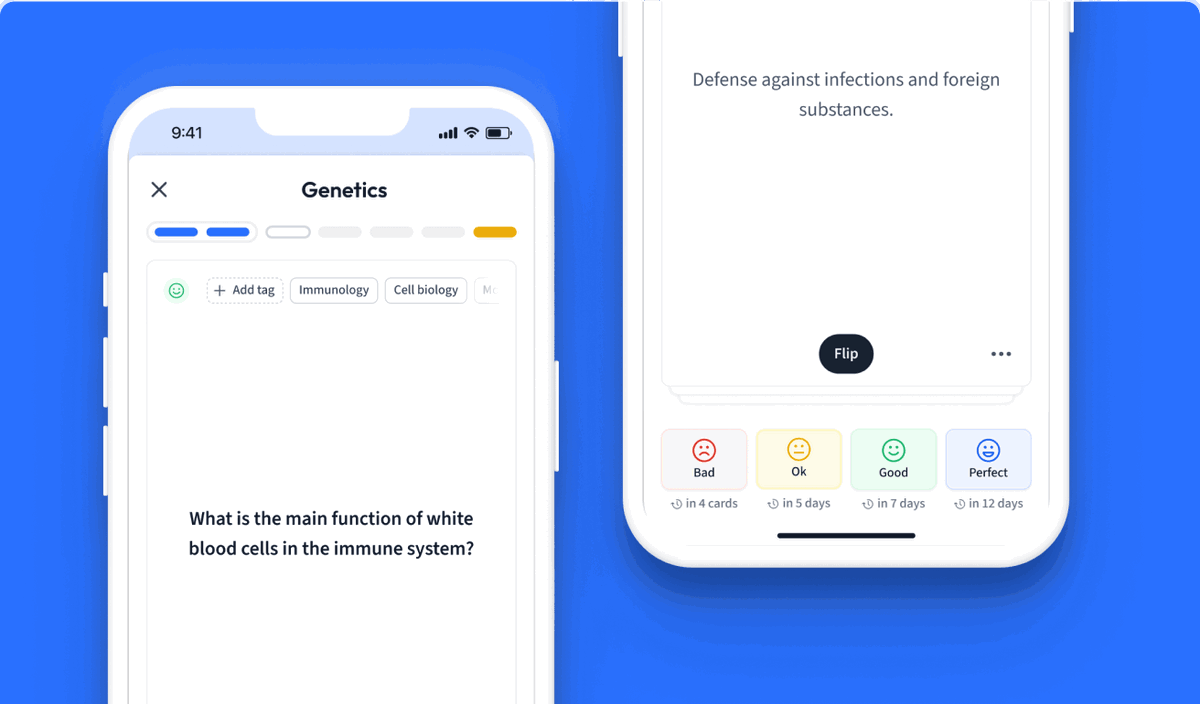
Learn with 12 reaction control flashcards in the free StudySmarter app
Already have an account? Log in
Frequently Asked Questions about reaction control
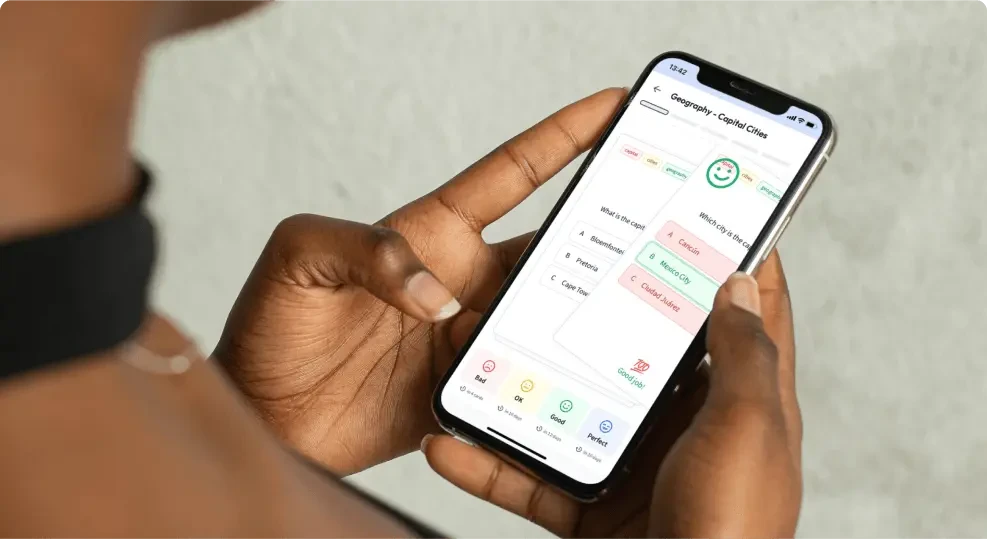
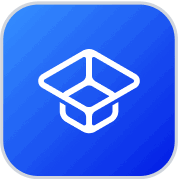
About StudySmarter
StudySmarter is a globally recognized educational technology company, offering a holistic learning platform designed for students of all ages and educational levels. Our platform provides learning support for a wide range of subjects, including STEM, Social Sciences, and Languages and also helps students to successfully master various tests and exams worldwide, such as GCSE, A Level, SAT, ACT, Abitur, and more. We offer an extensive library of learning materials, including interactive flashcards, comprehensive textbook solutions, and detailed explanations. The cutting-edge technology and tools we provide help students create their own learning materials. StudySmarter’s content is not only expert-verified but also regularly updated to ensure accuracy and relevance.
Learn more