Jump to a key chapter
What is Shape-Selective Catalysis
Shape-selective catalysis is a fascinating aspect of catalysis that utilizes materials with specific pore sizes and shapes to selectively convert molecules in chemical reactions. This property is particularly valuable in industries such as petrochemicals and pharmaceuticals, as it enhances product specificity and efficiency.
Understanding Shape-Selective Catalysis
Shape-selective catalysis involves catalysts that have pores capable of distinguishing between molecules of different sizes or shapes. These catalysts can either accept or reject molecules based on their dimensions, leading to selective transformation of molecules. This concept is akin to a lock and key mechanism, where only the right-sized molecule can fit and react within the catalyst's pores.
This selectivity primarily arises from the use of zeolites and molecular sieves. Zeolites are crystalline aluminosilicates with a unique three-dimensional porous structure. They can host reactions based on the size and geometry of the reactants and products, thereby enabling specific catalytic processes.
The practical applications of shape-selective catalysis are numerous. By focusing on molecular shape and size, industries can improve outcomes in:
- Isomerization processes, where one isomer is preferentially transformed.
- Cracking of hydrocarbons into smaller, more valuable components.
- Production of specific petrochemical derivatives.
One classic example of shape-selective catalysis is the use of zeolite ZSM-5 in converting methanol to gasoline. Due to its pore size, ZSM-5 allows the formation of hydrocarbons that fit within its framework, effectively guiding the catalytic process towards higher yields of desired gasoline components.
Interestingly, the development of shape-selective catalysts is connected to the broader field of nanotechnology. Understanding how to manipulate materials at the nanoscale can lead to the design of even more efficient catalysts with specialized pores, further improving catalytic reactions. Advanced computational models now assist scientists in predicting how variations in pore size and shape can affect catalytic behavior, leading to rapid innovations in the field.
Explain Shape-Selective Catalysis
In defining shape-selective catalysis, it is crucial to highlight the role of the catalyst's structure in determining reaction pathways. Catalysts used in this process typically include zeolites and certain types of porous polymers, designed to accommodate particular substrate sizes. This structural feature is pivotal in dictating which molecules can enter the catalyst and undergo reactions.
At the molecular level, the pores act as a sieve, facilitating reactions that involve molecules of appropriate size while inhibiting those that are too large. This not only increases efficiency but also reduces unwanted by-products, which can be particularly important in refining processes where precision is vital.
Moreover, shape-selective catalysis can influence:
- Activity: The rate at which reactions proceed can be optimized.
- Stability: Enhancing the lifespan of catalysts by minimizing clogging with larger molecules.
- Consistency: Achieving uniform product distribution in chemical processes.
Shape-selective catalysis: A catalytic process where the physical structure of the catalyst allows only certain molecules to participate in a chemical reaction, based on their size and shape.
When studying shape-selective catalysis, remember that not all catalysts are created equal. The precision of catalytic action largely depends on the specific design and arrangement of the catalyst's pores.
Shape Selective Catalysis Mechanism
Shape-selective catalysis is a unique mechanism where the shape and size of the catalyst determine the progress of a chemical reaction. By using catalysts like zeolites, which have well-defined pore structures, you can achieve highly selective reactions.
How Shape Selective Catalysis Works
The efficiency of shape-selective catalysis is attributed to the geometric constraints of the catalyst's pores. When a chemical reaction occurs, only molecules that match the pore size and shape can effectively participate. This selectivity is crucial for processes where a high product yield is desired, minimizing unwanted by-products.
The process works in the following way:
- Molecules enter the catalyst's pores based on size compatibility.
- Reactions occur inside the pores; oversized molecules are excluded.
- Products are expelled, often in a more desirable form or concentration.
Property | Effect |
Size of pore | Determines selectivity |
Shape of pore | Guides molecular orientation |
Think of a shape-selective catalyst as a molecular-sized filter, capable of controlling reaction specificity with great precision.
An excellent example is the conversion of toluene to para-xylene using zeolites. The specific pore size of zeolites ensures only the para-isomer (a more useful and profitable form) is predominantly formed.
Mathematical models help in understanding the effectiveness of the shape-selectivity mechanism. The kinetic equation for the rate of reaction, considering pore size, can be expressed as: \[r = k(T) \times f(\text{pore size})\times [A]\] where \(r\) is the reaction rate, \(k(T)\) is the reaction rate constant as a function of temperature, \(f(\text{pore size})\) is a function representing the dependency on pore size, and \([A]\) is the concentration of the reactant.
Factors Affecting Shape Selective Catalysis
Several factors influence the efficiency and selectivity of shape-selective catalysis:
- Pore size and distribution: The catalyst's pore dimensions must match those of the target molecules to ensure selectivity.
- Temperature: Elevated temperatures can affect reaction rates and selectivity, as it influences the movement of molecules.
- Catalyst composition: The materials and chemical makeup impact the strength and functionality of the catalyst.
These factors interact differently with a catalyst's properties. For example, an increase in temperature often increases reaction speeds, but may also affect the selectivity by causing undesirable reactions to occur. The interplay between these factors can be mathematically represented by:
The overall yield for a reaction taking place within a porous catalyst can be represented by: \[Y = \frac{f(\text{selectivity}, T)}{g(\text{diffusion}, \text{pore size})}\]
where \(Y\) is the yield, \(f\) is a function of selectivity and temperature, and \(g\) is a function related to diffusion and pore size.
Shape Selective Catalysis by Zeolites
In the realm of shape-selective catalysis, zeolites stand out as exemplary catalysts due to their unique structure. The structured nature of zeolites makes them particularly suitable for offering size-selective reactions, essential for optimizing chemical processes in various industries such as refining and petrochemicals.
Role of Zeolites in Shape Selective Catalysis
Zeolites are aluminosilicate minerals known for their intricate crystalline structure that provides them with a significant advantage in catalysis. Their role in shape-selective catalysis involves selectively transforming reactants by exploiting their pore sizes, which can be aligned with the molecular size of the reactants. This selectivity is central to enhancing reaction efficiency and specificity.
Zeolites facilitate reactions by:
- Providing a suitable environment for reactants to interact efficiently.
- Ensuring only specific reactants enter and participate in the reaction.
- Stabilizing transition states to favor particular reaction paths.
The mathematically modeled reaction rates within zeolites can be expressed as follows: \[R = k \times [A]^m \times [B]^n\] where \(R\) represents the rate of reaction, \([A]\) and \([B]\) are concentrations of reactants, and \(m\) and \(n\) are the reaction orders specific to those reactants.
A classic example involves the catalytic cracking of long-chain hydrocarbons using zeolite catalysts. Here, zeolites help break down large molecules into smaller, more useful fragments efficiently by selectively allowing only certain molecules to diffuse through their pore structures.
Exploring deeper into zeolite chemistry reveals their hydration-dehydration cycles which alter pore volume and surface area. This dynamic structural adaptability means that zeolites can be fine-tuned to achieve maximum efficiency for different chemical reactions. The total effective surface area that a zeolite offers can be computed, contributing to research in catalytic efficiency: \[S = p \times V\] where \(S\) is the surface area, \(p\) is the pore size distribution, and \(V\) is the accessible volume.
Structural Properties of Zeolites
The structural properties of zeolites are integral to their function in shape-selective catalysis. These properties include a three-dimensional lattice and channels that contribute to their ability to act as molecular sieves.
Key characteristics include:
- Pore size and shape: Determining factors for selectivity and efficiency of the catalytic process.
- Chemical composition: Influences the acidity and other chemical properties that aid in catalysis.
- Thermal stability: Allows zeolites to withstand high temperatures without degrading.
Structural Feature | Importance |
Cavity volume | Accommodates large reactants |
Channel connectivity | Facilitates efficient molecular transport |
The specific structural arrangement allows zeolites to act efficiently even under varying temperature and pressure conditions, making them robust and highly versatile catalysts in industrial applications.
Applications of Shape-Selective Catalysis
Shape-selective catalysis plays a crucial role across various industries due to its ability to enhance the selectivity and efficiency of chemical reactions. By tailoring catalysts to specific molecular shapes and sizes, industries can optimize processes, reduce waste, and achieve higher purity in products.
Industrial Applications of Shape Selective Catalysis
In the industrial sector, shape-selective catalysis is indispensable, particularly for refining and petrochemical processes. The ability to favor specific pathways in a complex reaction mixture is invaluable for producing high-value chemicals efficiently. Here are some of the key industrial applications:
- Hydrocracking: Utilized in the petroleum industry to convert heavy hydrocarbons into lighter, more valuable fractions like gasoline and diesel.
- Alkylation: The petrochemical industry uses this process to produce high-octane fuels by combining small alkene molecules with isobutane.
- Isomerization: Essential for the transformation of n-paraffins into branched isomers useful as fuel components.
The mathematical representation of catalytic efficiency in these processes can be expressed by the reaction kinetics equation: \[R = k \times C^n \] where \(R\) is the rate of reaction, \(k\) is the rate constant, \(C\) is the concentration of the substrate, and \(n\) is the reaction order.
An example in the oil refining industry involves using shape-selective zeolite catalysts in the catalytic cracking process. Zeolites aid in breaking down longer hydrocarbon chains into gasoline, improving both yield and quality by selectively cracking specific isomers.
Remember, the effectiveness of a shape-selective catalyst in industrial applications largely depends on its ability to sustain high temperatures and pressures typical in industrial settings.
Emerging Applications in Chemical Engineering
Shape-selective catalysis is beginning to influence innovative fields within chemical engineering, extending beyond traditional industrial applications. New research explores these systems to synthesize advanced materials and contribute significantly to green chemistry by making environmentally friendly processes more viable.
- Biomimetic Processes: Researchers develop catalytic systems mirroring biological pathways to achieve efficiency in artificial settings.
- Pharmaceutical Synthesis: The production of active pharmaceutical ingredients (APIs) benefits from selective catalytic processes to produce high-purity compounds efficiently.
- Green Catalysis: Shape-selective mechanisms enable environmentally benign reactions, minimizing harmful by-products.
The equation depicting catalyst turnover can be represented as: \[\text{Turnover Frequency} = \frac{\text{Number of Molecules Converted}}{\text{Catalyst Amount} \times \text{Time}} \]
Future advancements in shape-selective catalysis may be propelled by nanotechnology and molecular engineering. These emerging sciences aid in designing catalysts with even more precise and customizable pore dimensions, potentially improving both reaction specificity and efficiency. Efforts such as computational modeling of catalyst structures and processes could lead to breakthroughs in catalyst design.
shape-selective catalysis - Key takeaways
- Shape-selective catalysis: Involves using catalysts with specific pore sizes and shapes to selectively process molecules based on size and shape, enhancing specificity and efficiency, especially in petrochemical and pharmaceutical industries.
- Zeolites in catalysis: Zeolites are aluminosilicates with unique porous structures, making them ideal for shape-selective catalysis by allowing specific reactants to enter based on molecular size and shape.
- Mechanism: Shape-selective catalysis functions through a lock-and-key mechanism; only molecules that match the pore size can partake in reactions, minimizing unwanted by-products and increasing efficiency.
- Applications: Shape-selective catalysis is key in processes like hydrocracking, alkylation, and isomerization, facilitating the production of valuable chemical derivatives while optimizing the chemical processes.
- Key Factors: Efficiency depends on factors like pore size and distribution, temperature, and catalyst composition, dictating reaction speeds and selectivity.
- Emerging applications: Innovations in this field cater to green catalysis, pharmaceutical synthesis, and biomimetic processes, with potential enhancements from nanotechnology and molecular engineering.
Learn faster with the 12 flashcards about shape-selective catalysis
Sign up for free to gain access to all our flashcards.
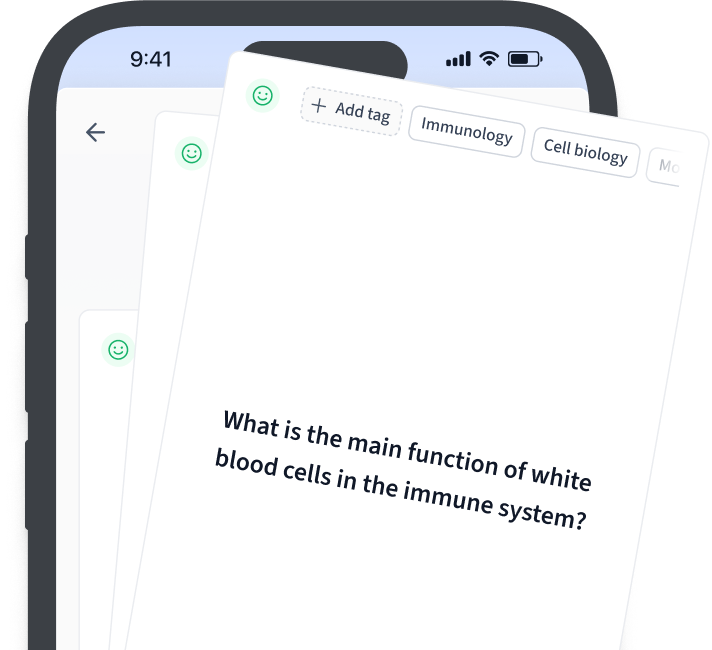
Frequently Asked Questions about shape-selective catalysis
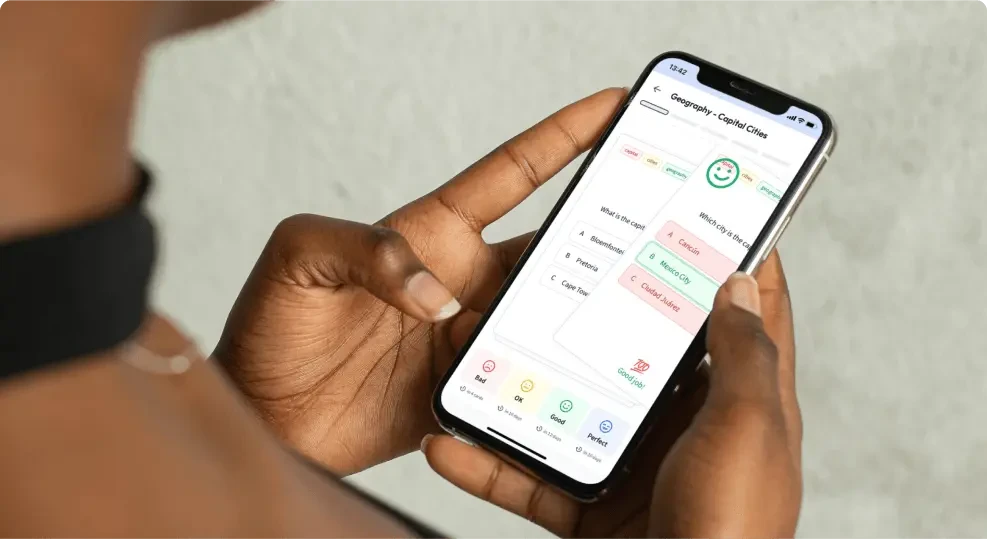
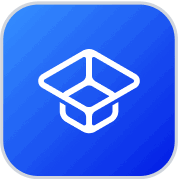
About StudySmarter
StudySmarter is a globally recognized educational technology company, offering a holistic learning platform designed for students of all ages and educational levels. Our platform provides learning support for a wide range of subjects, including STEM, Social Sciences, and Languages and also helps students to successfully master various tests and exams worldwide, such as GCSE, A Level, SAT, ACT, Abitur, and more. We offer an extensive library of learning materials, including interactive flashcards, comprehensive textbook solutions, and detailed explanations. The cutting-edge technology and tools we provide help students create their own learning materials. StudySmarter’s content is not only expert-verified but also regularly updated to ensure accuracy and relevance.
Learn more