Jump to a key chapter
State Functions Definition
When studying thermodynamics and engineering, you often come across the term state functions. These are properties that depend only on the current state of a system, not on the path taken to reach that state. Understanding state functions is crucial in analyzing thermodynamic processes.
Characteristics of State Functions
State functions have distinguishing features that set them apart from other quantities. Here are some key characteristics:
- **Path Independence**: State functions are independent of the process path. Only initial and final states matter.
- **Exact Differential**: Mathematically, state functions are represented as exact differentials.
- **Inherent in the System**: Depend solely on system properties, such as temperature and pressure.
An example of a state function is enthalpy (H), a measure of total energy of a thermodynamic system, expressed as:\(H = E + PV\)where:
- \(E\): Internal energy
- \(P\): Pressure
- \(V\): Volume
Common State Functions
Several properties in thermodynamics are classified as state functions. Here are some of them:
- Internal Energy (U): Total energy contained in a system.
- Enthalpy (H): As explained before, it comprises internal energy and the energy to displace its environment.
- Entropy (S): Measure of disorder or randomness in a system.
- Gibbs Free Energy (G): Energy associated with a system that can be used to do work, given by: \(G = H - TS\)
where \(T\) is the temperature and \(S\) is the entropy.
Consider a gas in a piston with initial state variables of pressure \(P_1\), volume \(V_1\), and temperature \(T_1\). If the gas undergoes compression to \(P_2\), \(V_2\), and \(T_2\), the changes in state functions like internal energy depend only on these variables, not the compression path.
Remember, work and heat are not state functions, because they depend on the path taken in a process.
In thermodynamics, you distinguish between two main types of functions: state functions and path functions. While state functions depend solely on the current state, path functions vary with the process path. For instance, consider two routes from point A to B—a straight line and a zigzag. The elevation change is a state function (same for both), while distance is a path function (varies between routes).
What Are State Functions?
Understanding state functions is essential in fields like thermodynamics and chemistry. These functions describe physical systems in thermal equilibrium based on their current state, rather than on the path taken to reach this state.
Definition of State Functions
State Functions: A property of a system that determines the system's condition, independent of how this condition was achieved. State functions are typically represented through variables like temperature, pressure, and volume. They are also known as state variables.
How State Functions Work
State functions operate under specific principles:
- Depend only on the initial and final states of the system, making them path-independent.
- Useful for determining systems' properties without considering energy exchanges.
- Include primary variables such as internal energy (U), enthalpy (H), entropy (S), and Gibbs free energy (G).
\[ U = Q - W \]
where:- \(Q\) is the heat exchanged.
- \(W\) is the work done on the system.
Imagine a gas confined in a cylinder. As the gas is compressed or expanded, the state functions (such as pressure and volume) will change but will not consider the path of compression or expansion. For a complete cycle, the internal energy change is zero because the system returns to its original state.
Applications of State Functions
State functions are fundamental in analyzing thermodynamic processes:
- Enthalpy changes are used to measure heat transfer in chemical reactions, given by: \[ \Delta H = \Delta U + \Delta(PV) \]
- Entropy is key in understanding the second law of thermodynamics—how energy disperses and spreads in a system.
- Gibbs Free Energy helps predict the feasibility of reactions, determined by: \[ G = H - TS \]
Remember, while state functions depend on initial and final states, path functions like work and heat rely on the process route.
State functions derive from the fundamental axiom that equilibrium states can be completely defined by a set number of variables. Their path independence means they simplify the analysis of complex systems, providing exact information through changes in measurable properties.
Properties of State Functions
The study of thermodynamics introduces a variety of properties that are crucial for understanding system behavior. Among these properties, state functions hold a special significance. They provide essential insights into system states without being dependent on the process path followed.
Defining Characteristics of State Functions
- **Path Independence**: The value of state functions depends solely on the initial and final states of a system. The process path between these states is irrelevant.
- **Exact Differential**: Mathematically, state functions are expressed as exact differentials, which means their change is independent of the path.
- **System-Dependent Properties**: They rely only on the properties of the system at a particular moment, such as pressure, volume, and temperature.
Consider the internal energy (U): A key state function representing the total energy contained within a system. It incorporates both kinetic and potential energy of the molecules.
Significant Examples of State Functions
**Internal Energy**: If a gas undergoes expansion from an initial state \(P_1, V_1, T_1\) to a final state \(P_2, V_2, T_2\), the change in internal energy \(\Delta U\) is path-independent and determined by the states:
\[\Delta U = U_2 - U_1\]
Other critical state functions include:
- Enthalpy (H): Represents the heat content of a system at constant pressure, defined by:\[H = U + PV\]
- Entropy (S): A measure of disorder or randomness in the system.
- Gibbs Free Energy (G): Used to predict the directions of chemical processes, given by:\[G = H - TS\]
The concept of state functions originates from the fact that the equilibrium states of systems can be completely characterized by a finite set of variables. These specific functions are invaluable in simplifying the analysis of highly intricate systems since they provide a clear view of the measurable property changes, without the need to account for process specifics.Understanding the linear combination of state functions can aid in deciphering complex systems where pressure, volume, or temperature interplays in dynamic equilibrium, ensuring accurate predictions of system changes.
State functions are contrasted with path functions, such as work and heat, which rely heavily on the specific pathway or process taken.
Thermodynamic State Functions Examples
To grasp the concept of state functions in thermodynamics, it's helpful to look at specific examples. These properties play a crucial role in describing the equilibrium states of systems without relying on the process path.By focusing on differences between initial and final states, state functions simplify the study of changes within systems.
Internal Energy Change Example
Consider a gas that expands from an initial state \((P_1, V_1, T_1)\) to a final state \((P_2, V_2, T_2)\). The change in internal energy is given by:
\[\Delta U = U_2 - U_1\]
This value is independent of the path taken during expansion.
Enthalpy in Chemical Reactions
The change in enthalpy (H) during chemical reactions is a common application of state functions, used to measure heat transfer at constant pressure:
- Enthalpy change is expressed as: \[\Delta H = H_2 - H_1\]
- If a reaction occurs at constant pressure, heat transfer can be directly calculated using enthalpy change.
Entropy and Disorder
Entropy (S) is another vital state function, reflecting the disorder or randomness of a system:
- Entropy change is noted as: \[\Delta S = S_2 - S_1\]
- An increase in entropy indicates higher randomness, following the second law of thermodynamics.
Each state function interacts uniquely within thermodynamic processes. Consider the role of Gibbs Free Energy (G):
It's defined as:\[G = H - TS\]where:
- \(H\) is enthalpy,
- \(T\) is temperature,
- \(S\) is entropy.
This function is used to discern whether a reaction will occur spontaneously at constant pressure and temperature. By understanding how Gibbs Free Energy varies, you can assess a system's potential to perform work.
State functions help distinguish between spontaneous and non-spontaneous processes without detailing the process path.
state functions - Key takeaways
- State Functions Definition: Properties of a system that depend solely on its current state, not on the path taken to reach that state, often represented by variables like temperature, pressure, and volume.
- Characteristics of State Functions: Path independence, exact differentials, and dependence only on system properties such as temperature and pressure.
- Examples of State Functions: Internal Energy (U), Enthalpy (H), Entropy (S), and Gibbs Free Energy (G), all crucial in thermodynamic analysis.
- Properties of State Functions: These include path independence, exact differential nature, and reliance on system-dependent properties like pressure, volume, and temperature.
- Applications of State Functions: Used in thermodynamics to simplify analysis of system changes and predict outcomes of chemical reactions without considering process paths.
- Thermodynamic State Functions: Essential for understanding equilibrium states in systems and include functions like internal energy change, enthalpy in reactions, and entropy reflecting disorder.
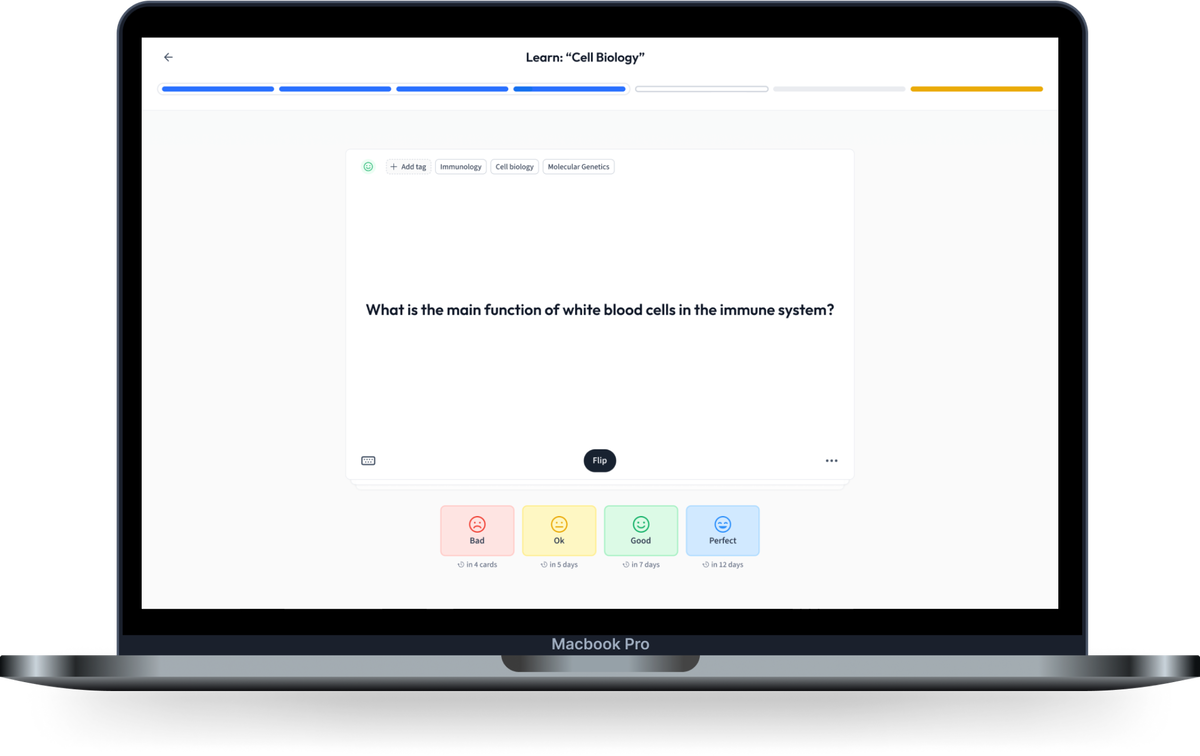
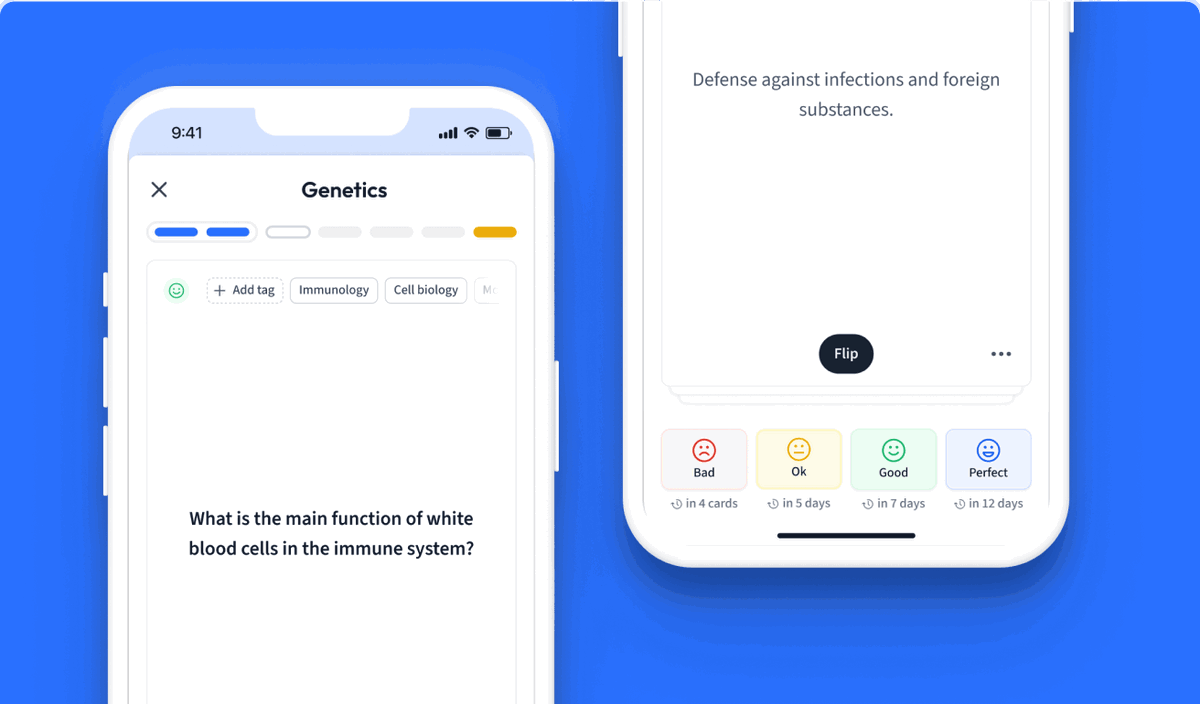
Learn with 12 state functions flashcards in the free StudySmarter app
Already have an account? Log in
Frequently Asked Questions about state functions
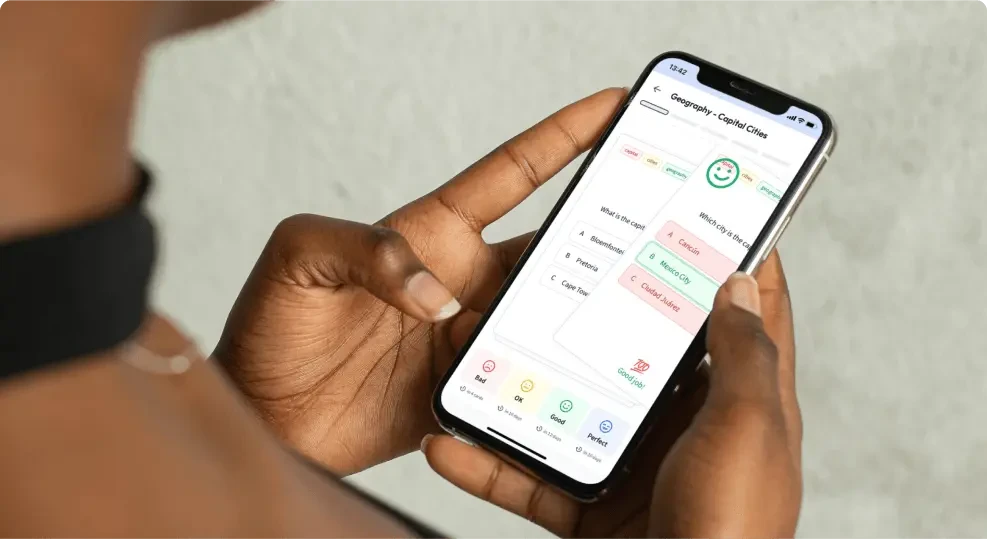
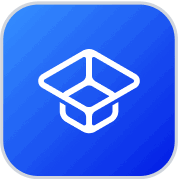
About StudySmarter
StudySmarter is a globally recognized educational technology company, offering a holistic learning platform designed for students of all ages and educational levels. Our platform provides learning support for a wide range of subjects, including STEM, Social Sciences, and Languages and also helps students to successfully master various tests and exams worldwide, such as GCSE, A Level, SAT, ACT, Abitur, and more. We offer an extensive library of learning materials, including interactive flashcards, comprehensive textbook solutions, and detailed explanations. The cutting-edge technology and tools we provide help students create their own learning materials. StudySmarter’s content is not only expert-verified but also regularly updated to ensure accuracy and relevance.
Learn more