Jump to a key chapter
Synthesis of Sulfonated Carbon Catalysts
Sulfonated carbon catalysts are an integral part of chemical reactions, particularly in catalytic processes. These catalysts are engineered through various synthesis methods, utilizing specific raw materials. Understanding how they are synthesized will help you grasp their applications in enhancing reaction efficiencies.
Methods for Synthesis
There are several methods to synthesize sulfonated carbon catalysts. Each method has its own set of procedures and requirements:
- Hydrothermal Carbonization: This technique involves the conversion of carbohydrates into carbon materials at high temperatures and pressure in the presence of water.
- Chemical Activation: In this method, carbon material is treated with chemicals like H2SO4 to introduce sulfonic acid groups.
- Direct Sulfonation: This involves directly treating carbon materials with sulfuric acid to incorporate sulfonic acid groups on their surface.
Example: In hydrothermal carbonization, utilizing glucose as a starting material can lead to a high yield of carbon catalysts due to its high carbon content.
Hydrothermal carbonization can sometimes result in larger particle sizes, which may affect the distribution of active sites.
Raw Materials Used in Synthesis
Raw materials for synthesizing sulfonated carbon catalysts vary widely. The choice of raw material can greatly influence the properties and efficiency of the catalyst produced. Some common materials include:
- Biomass: Biomass from agricultural waste like sugarcane bagasse, sawdust, or rice husks provides a renewable source.
- Fossil-based Precursors: Coal and petroleum coke are utilized due to their high carbon content.
- Carbohydrates: Simple carbohydrates like glucose or sucrose are easily converted into carbonaceous materials.
Deepdive: Biomass is an attractive alternative for synthesizing carbon catalysts due to its sustainability. By using waste materials, this approach not only reduces environmental impact but also provides a cost-effective source of raw material. In fact, the carbon skeleton of biomass can be easily retained during the sulfonation process, offering high surface area and a better distribution of catalytic sites. The resultant products are often more eco-friendly and can lead to the creation of functionalized catalysts that influence a diverse range of chemical reactions.
Mechanism of Sulfonated Carbon Catalysts
Understanding the mechanism of sulfonated carbon catalysts is crucial for comprehending their role in speeding up chemical reactions. These catalysts are characterized by their ability to provide specific sites for reaction, which can lead to enhanced reaction rates and selectivity.
Catalytic Mechanisms
Sulfonated carbon catalysts operate through the presence of active sites, typically sulfonic acid groups, which facilitate reactions. Here's how these mechanisms work:
- Proton Donation: The sulfonic acid group provides protons, making it crucial for acid-catalyzed reactions such as esterification and alkylation.
- Enhanced Surface Area: The porous structure of the carbon material offers a large surface area, providing ample room for reactants to adsorb and react.
- Stability: The strong carbon framework ensures that these catalysts maintain their integrity under diverse reaction conditions.
Deepdive: The porous architecture of sulfonated carbon catalysts not only enhances their catalytic activity but also offers unique advantages over traditional liquid acids. The distribution of pores can be meticulously controlled during synthesis to optimize catalyst performance for specific reactions. By tailoring pore size and volume, catalysts can offer better accessibility to reactants, thereby boosting the speed and efficiency of catalytic processes.
Sulfonated carbon catalysts are often reusable, making them environmentally friendly alternatives to traditional catalysts.
Role in Chemical Reactions
These catalysts play a significant role in accelerating chemical reactions. Their unique properties provide several benefits to various industrial sectors:
- Selectivity: They enable selective reactions, aiding in producing desired products with minimal by-product formation.
- Versatility: From biodiesel production to petrochemical processes, these catalysts are applicable across various chemical industries.
- Green Chemistry: Using sulfonated carbon catalysts supports sustainable practices by reducing the need for hazardous chemicals.
Example: In the esterification process, sulfonated carbon catalysts effectively enhance the reaction rate and yield of esters, often used in fragrance and flavor production.
Properties of Sulfonated Carbon Catalysts
Sulfonated carbon catalysts possess distinct properties that make them exceptionally valuable in catalysis. Their unique attributes are categorized into physical and chemical properties, each contributing to their performance and applicability.
Physical Properties
Understanding the physical properties of sulfonated carbon catalysts is crucial. These catalysts offer a combination of characteristics that enhance their effectiveness:
- Surface Area: These catalysts exhibit a large surface area due to their porous nature, allowing more active sites for reactions.
- Porosity: The controlled pore distribution enhances catalyst interaction with different molecular sizes.
- Thermal Stability: They maintain structural integrity at elevated temperatures, essential for high-temperature reactions.
Example: The high surface area of sulfonated carbon catalysts was utilized in a study where it significantly increased the rate of esterification in converting fatty acids to esters.
Larger pore sizes can sometimes hinder catalyst effectiveness by reducing the concentration of active sites per unit area.
Chemical Properties
The chemical properties of sulfonated carbon catalysts are primarily defined by their surface functionalities and stability under reaction conditions:
- Acidity: The presence of sulfonic acid groups endows these catalysts with strong acidic properties, facilitating proton donation in reactions.
- Chemical Resistance: These catalysts resist chemical attack in acidic and basic environments, ensuring longevity.
- Reusability: Due to their robustness, sulfonated carbon catalysts can be regenerated and reused without significant performance loss.
Deepdive: The high density of sulfonic acid groups on the surface of these catalysts is critical for their performance. You can calculate the acidity level of a catalyst by the number of sulfonic groups per unit area, which affects the turnover frequency of the catalytic process. Using the formula \[\text{Turnover Frequency (TOF)} = \frac{\text{moles of product}}{\text{moles of active sites} \times \text{time}}\], the effectiveness of the catalyst in various reactions can be evaluated.
Engineering Applications of Sulfonated Carbon Catalysts
Sulfonated carbon catalysts have a significant impact on various engineering applications. From environmental technologies to industrial processes, these catalysts provide efficient and eco-friendly solutions for diverse challenges.
Environmental Applications
In environmental engineering, the use of sulfonated carbon catalysts has paved the way for numerous sustainable practices. These catalysts help address pollution and waste management issues effectively.
- Water Treatment: Sulfonated carbon catalysts play a vital role in removing organic contaminants from wastewater through advanced oxidation processes.
- Air Purification: They are used for air pollution control by facilitating reactions that reduce volatile organic compounds (VOCs) and other pollutants.
Using sulfonated carbon catalysts in water treatment can significantly reduce chemical consumption, making processes more sustainable.
Example: A study demonstrated the effectiveness of sulfonated carbon catalysts in the catalytic wet peroxide oxidation (CWPO) process, where they enhanced the degradation of phenolic compounds in wastewater.
Deepdive: In addressing environmental challenges, sulfonated carbon catalysts provide not just reactive surfaces but also a framework for adsorbing pollutants, thereby increasing contact time with reactive intermediates. Using the formula for the rate of reaction \ \[ r = k \cdot C_A \cdot C_B \] \ where \( r \) is the reaction rate, \( k \) is the rate constant, and \( C_A \), \( C_B \) are the concentrations of reactants. The catalysts help maintain high concentrations at the reactive sites, promoting faster degradation of contaminants.
Industrial Applications
Industrial applications of sulfonated carbon catalysts are vast and varied. These catalysts support various chemical productions with enhanced efficiency.
- Petrochemical Industry: They are used in processes like alkylation and isomerization to increase the yield of high-octane fuels.
- Biodiesel Production: Sulfonated carbon catalysts facilitate the transesterification process, converting oils into biodiesel efficiently.
- Pharmaceuticals: In the synthesis of complex organic compounds, these catalysts contribute to higher reaction rates and yields.
Example: In biodiesel production, sulfonated carbon catalysts have demonstrated successful conversion of waste cooking oils into biodiesel, helping to recycle materials and reduce waste.
Examples of Sulfonated Carbon Catalysts
Sulfonated carbon catalysts are utilized in a variety of chemical reactions due to their effectiveness and versatility. These catalysts are particularly important in enhancing reaction mechanisms and efficiencies.
Carbon-Based Sulfonated Catalyst Examples
In exploring examples of carbon-based sulfonated catalysts, several specific configurations stand out, each allowing for diverse applications and reaction improvements:
- Graphene-based Catalysts: Known for their high surface area and electron mobility, these catalysts are effective in electrochemical reactions.
- Sugar-derived Carbon Catalysts: These are synthesized from carbohydrates, offering sustainable and economical options for various reactions.
- Activated Carbon: This type, when sulfonated, provides substantial acidic functions for reactions like esterification and transesterification.
Definition: A catalyst is a substance that speeds up a chemical reaction without being consumed in the process. It provides an alternative reaction pathway with a lower activation energy.
Example: A recent application involved the use of graphene oxide-based sulfonated catalysts for improving the rate of the Fischer–Tropsch synthesis, a critical process for converting carbon monoxide and hydrogen into hydrocarbons.
Deepdive: One fascinating example of sugar-derived carbon catalysts involves the use of sucrose. Sucrose can be transformed into carbonaceous material through hydrothermal treatment, followed by sulfonation with concentrated sulfuric acid. This produces a catalyst with a high density of sulfonic acid groups, making it highly effective in processes such as biodiesel synthesis. The sulfonic acid groups provide strong proton-donating capabilities, enhancing the transesterification rates considerably, which can be calculated using the reaction rate equation \[ r = k \cdot [A][B] \, \text{where } \[ r \text{ is the rate and } \ [A] \text{ and } \[ B \text{ are reactant concentrations.}
Graphene-based catalysts can be more expensive due to the high costs involved in graphene synthesis.
Comparative Studies with Other Catalysts
When studying sulfonated carbon catalysts against other types of catalytic materials, there are notable comparisons in terms of performance and application.
- Acidic Zeolites: While providing strong acidity and high thermal stability, they often lack the reusability and environmental benefits seen with sulfonated carbons.
- Metal Oxides: These are efficient in specific processes like oxidation but may suffer from leaching and are not as eco-friendly.
- Homogeneous Catalysts: Although effective, these pose challenges in separation from reaction mixtures and pose questions about environmental impact.
Example: A comparative study demonstrated that sulfonated carbon catalysts surpassed the activity of traditional zeolites in the dehydration of bio-alcohols, showing both a higher yield and selectivity.
Sulfonated carbon catalysts are often chosen in green chemistry applications due to their minimal environmental impact and effective performance.
sulfonated carbon catalysts - Key takeaways
- Sulfonated carbon catalysts: Used in catalytic processes to enhance reaction efficiencies.
- Mechanism of sulfonated carbon catalysts: Operates through active sites like sulfonic acid groups for proton donation and offers high surface area and stability.
- Engineering applications: Used in industries such as petrochemical, biodiesel production, and pharmaceuticals for efficient and sustainable processes.
- Synthesis methods: Include hydrothermal carbonization, chemical activation, and direct sulfonation, influencing catalyst properties such as porosity and stability.
- Properties: Highlight attributes like large surface area, porosity, thermal stability, acidity, chemical resistance, and reusability.
- Examples: Include graphene-based catalysts, sugar-derived carbon catalysts, and sulfonated activated carbon for various industrial applications.
Learn faster with the 10 flashcards about sulfonated carbon catalysts
Sign up for free to gain access to all our flashcards.
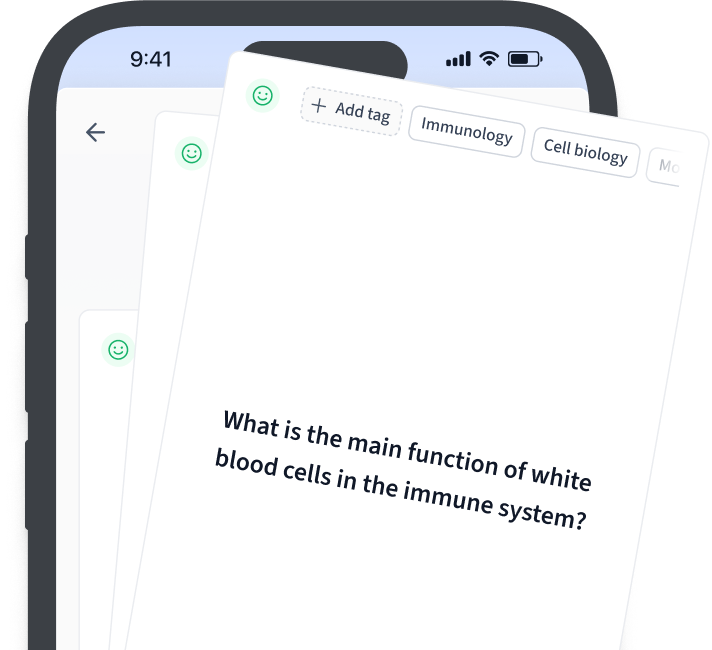
Frequently Asked Questions about sulfonated carbon catalysts
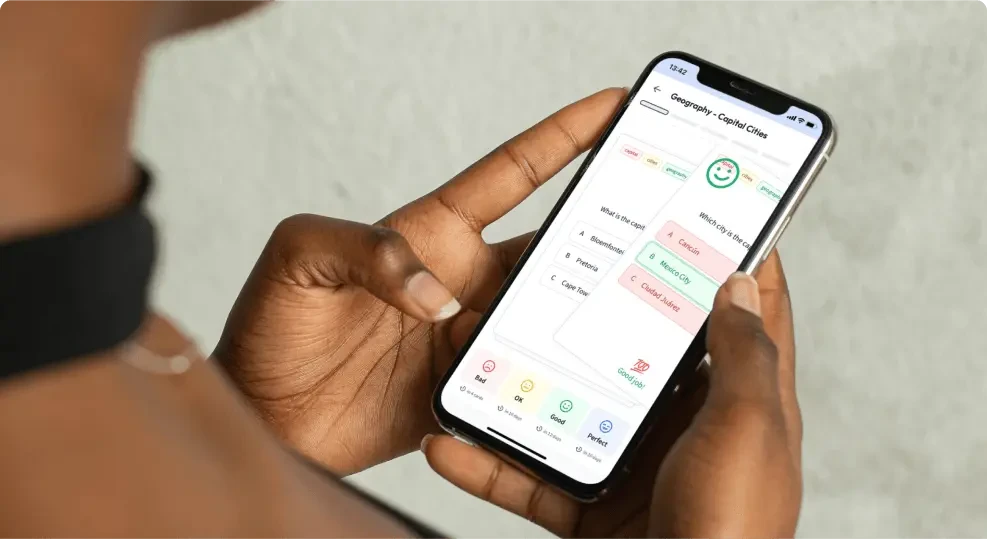
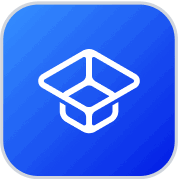
About StudySmarter
StudySmarter is a globally recognized educational technology company, offering a holistic learning platform designed for students of all ages and educational levels. Our platform provides learning support for a wide range of subjects, including STEM, Social Sciences, and Languages and also helps students to successfully master various tests and exams worldwide, such as GCSE, A Level, SAT, ACT, Abitur, and more. We offer an extensive library of learning materials, including interactive flashcards, comprehensive textbook solutions, and detailed explanations. The cutting-edge technology and tools we provide help students create their own learning materials. StudySmarter’s content is not only expert-verified but also regularly updated to ensure accuracy and relevance.
Learn more