Jump to a key chapter
Definition of Utility Systems in Engineering
Utility systems are an integral part of engineering that ensure the smooth operation and maintenance of primary systems and infrastructure. These systems encompass a variety of services essential for industrial, commercial, and residential areas. They play a crucial role in enabling the effective functioning of key operational requirements.
Utility Systems Explained
Utility systems, in the realm of engineering, refer to the complex network of systems designed to support various non-core activities of a building or facility. These systems are vital for maintaining the operational flow and ensuring the efficiency of primary business operations. Understanding utility systems involves examining each of the component systems that contribute to the larger infrastructure.
- Water Systems: These include water treatment, supply, and waste management systems. Water is essential for various industrial processes and human needs.
- Electricity Systems: These systems manage the distribution of electrical power required for various equipment and systems.
- Heat and Ventilation Systems: These maintain the ambient conditions necessary for equipment operation and human comfort.
- Compressed Air Systems: Devoted to delivering compressed air used in various tools and machinery.
- E is the energy used in kilowatt-hours (kWh)
- P is the power in kilowatts (kW)
- t is the time in hours (h)
A deeper understanding of utility systems can lead to significant advantages in cost reduction and efficiency improvement. For instance, take the energy efficiency of a heating system. By utilizing advanced technologies like variable speed drives, which adjust the speed of motors based on demand, you can enhance energy efficiency by as much as 30%.Moreover, the integration of smart grids has transformed utility management. A smart grid allows for real-time data analysis, enabling predictive maintenance and system optimizations that were not previously possible. It reduces energy waste, improves reliability, and allows for integration with renewable energy sources.On the mathematical side, optimizing utility systems often involves understanding various equations and formulas, such as those for thermal efficiency. The thermal efficiency of a heat engine is a crucial metric:\[ \eta = \frac{W_{\text{out}}}{Q_{\text{in}}}\times 100\%\]Where:
- \( \eta \) is the thermal efficiency as a percentage
- \( W_{\text{out}} \) is the work output from the engine
- \( Q_{\text{in}} \) is the heat input to the engine
Importance of Utility Systems in Engineering
Utility systems form the backbone of numerous engineering sectors. From supporting industrial operations to facilitating residential living, they ensure the integrated and efficient functioning of various systems. Let's delve into their significant roles and impacts.
Role in Modern Infrastructure
In modern infrastructure, utility systems are indispensable. Here’s a closer look at their roles:
- Electrical Systems: Power is indispensable for any modern infrastructure. Electrical systems deliver energy required for lighting, powering appliances, and running complex machinery. The calculation of electrical power consumed can be defined by\[P = VI\]where \(P\) is the power in watts, \(V\) is the voltage in volts, and \(I\) is the current in amperes.
- Water Supply Systems: These systems ensure water is supplied consistently for both industrial processes and domestic use, crucial to sustaining life and operations.
- Gas Systems: Gas is used in heating and powering certain machinery. Managing these systems is vital to improving efficiency and safety.
- Telecommunication Systems: They enable instant communication and data exchange, which are essential for businesses and personal use.
Consider a typical city power grid. As more sectors get powered, load management becomes crucial.
Sector | Power Demand (kW) |
Residential | 5,000 |
Industrial | 15,000 |
Commercial | 10,000 |
In utility systems, particularly electrical and thermal systems, the concept of redundancy is often applied. This ensures that if one system fails, others can take over, preventing significant disruption.
Impact on Sustainability
Utility systems are increasingly pivotal in the pursuit of sustainability. Efficient systems reduce resource wastage, and innovation in these utilities can significantly lower the carbon footprint.Here are several ways utility systems contribute to sustainability:
- Energy Efficiency: Systems like LED lighting and programmable thermostats radically improve energy efficiency.
- Water Conservation: Advanced plumbing systems and water treatment technologies reduce consumption and enable recycling for environmental conservation.
- Waste Management: Effective waste-to-energy systems convert waste products into usable energy, reducing landfill use.
Looking further into sustainability, the design of utility systems themselves has evolved. For example, smart grids use AI to anticipate demand and optimize distribution, which can reduce energy waste by up to 20%. Smart grids adjust supply in real-time, responding to fluctuations in demand instantly.Moreover, the development of renewable energy sources such as solar, wind, and geothermal energy is heavily dependent on efficient utility systems for storage and distribution. For solar panels, you may calculate energy input with:\[E = A \times r \times H \times PR\]where \(E\) is the energy output in kWh, \(A\) is the area of the solar panel in square meters, \(r\) is the solar panel yield, \(H\) is the average sunlight hours per day, and \(PR\) is the performance ratio.Such technological advancements transform utility systems from traditional models to ones more aligned with ecological preservation and sustainability.
Utility Systems Engineering Techniques
Understanding utility systems in engineering requires a focus on the techniques used for their design and optimization. It is also crucial to explore how these systems integrate with others to ensure efficiency and sustainability. We'll cover essential strategies and methods that highlight the complexity and innovation involved in utility systems engineering.
Design and Optimization Methods
Designing an efficient utility system involves meticulous planning and a focus on optimizing resources. Here are some key strategies:
- Energy Auditing: Regular auditing helps identify areas of energy waste and allows engineers to come up with solutions to minimize these inefficiencies.
- Computational Fluid Dynamics (CFD): This computer-aided engineering tool is used to solve fluid flow equations, helping predict how gases and liquids behave in systems such as HVAC and water distribution.
- Thermal Modeling: Mathematical models illustrate heat transfer within utility systems. The basic heat transfer equation is:\[Q = mc\Delta T\]where \(Q\) represents the heat transferred, \(m\) is mass, \(c\) is specific heat capacity, and \(\Delta T\) is temperature change.
- Advanced Control Systems: These systems use sensors and algorithms to automatically adjust operations, enhancing efficiency.
Consider an HVAC system, which uses CFD for optimization. Engineers model airflow patterns and temperature distribution to refine ductwork design and reduce energy consumption. This ensures optimal thermal comfort using minimal resources.
To delve deeper into optimization, consider the role of machine learning in utility systems. Machine learning algorithms can predict energy demand and adjust supply dynamically, creating a responsive and efficient system. For example, demand forecasting can be achieved through neural networks employing these formulas:\[ \text{Input: } X = [x_1, x_2, ..., x_n]\]\[ y = f(WX + b) \]where \(X\) is the input vector, \(W\) represents the weights, \(b\) is the bias, and \(f\) the activation function.This sophisticated approach allows utility systems to react intelligently to varying demands and maintain operational integrity. Some utility companies report efficiency gains by up to 15% after implementing AI-driven control systems.
Integration with Other Systems
Integrating utility systems with other infrastructure systems provides enhanced performance and reduced operational costs. The integration process involves synchronization with various platforms and technologies:
- Building Management Systems (BMS): These systems centralize control and monitoring of a facility’s mechanical and electrical services, increasing efficiency.
- Smart Grids: These leverage connectivity between distributed energy resources, providing real-time analytics and enabling dynamic energy distribution.
- IoT Devices: The Internet of Things allows for the collection of large data sets used to monitor system health and performance, adapting to changing conditions seamlessly.
IoT and smart grids can work synergistically for effective demand-side management, further optimizing energy distribution and reducing peak demand pressures.
The integration of utility systems with emerging technologies represents a leap forward in engineering capabilities. Blockchain technology is an exciting advancement, ensuring transparency and robustness in energy transactions. With this decentralized technology, utility systems can securely and efficiently manage energy trades and data verification. For instance, some municipal power utilities use blockchain to track energy provenance, ensuring that energy supplied from renewable sources is accurately accounted for.Furthermore, blockchain utilizes cryptographic algorithms for secure data handling:
'hash = sha256(previous_block_hash + transaction_data + timestamp)'This ensures a secure and tamper-evident record of transactions, making it highly reliable for various trades within integrated utility systems.Such integrations are crucial as they enable more decentralized, reliable, and sustainable utility systems that can power smart cities of the future.
Utility Systems Analysis
Analyzing utility systems involves examining their efficiency, effectiveness, and integration with core processes. Such analysis can lead to system enhancements and better resource utilization. The focus is on employing techniques that evaluate system performance and sustainability.
Techniques for Effective Evaluation
Evaluating utility systems requires a structured approach using various techniques to ensure their optimal functioning. Below are some important techniques:
- Benchmarking: This involves comparing system performance against industry standards to identify areas for improvement.
- Energy Modeling: Utilizes mathematical models to assess the energy flow and consumption within systems. An example formula is:\[E_{\text{total}} = \sum (E_{\text{input}} - E_{\text{loss}})\]where \(E_{\text{total}}\) is the total energy used, \(E_{\text{input}}\) is the energy input, and \(E_{\text{loss}}\) is the energy lost.
- Simulation Techniques: Allow the visualization of utility system operations under different scenarios, predicting possible outcomes and adjustments.
- Performance Metrics: Metrics are established to quantify efficiency and productivity, such as energy use intensity (EUI).
Consider a scenario where you are evaluating the energy efficiency of a building's HVAC system. Using energy modeling, you can determine how the system's adjustments during various seasons affect energy consumption. A simple model could apply:\[E_{\text{HVAC}} = c_{1}T_{\text{out}} + c_{2}T_{\text{in}}\]where \(E_{\text{HVAC}}\) is the energy consumed, \(c_{1}\) and \(c_{2}\) are coefficients, and \(T_{\text{out}}\) and \(T_{\text{in}}\) are the outside and inside temperatures respectively. Such evaluations lead to strategic adjustments within the system for optimal performance.
Digging deeper into evaluation techniques highlights the importance of predictive analytics in utility systems. Predictive analytics employ vast datasets and machine learning algorithms to forecast potential issues and maintenance needs. By utilizing time-series analysis and regression models, you can preemptively address potential failures or inefficiencies before they escalate.Predictive models might use algorithms like:
'model = SARIMA(order=(p,d,q), seasonal_order=(P,D,Q,s))'Here, SARIMA (Seasonal AutoRegressive Integrated Moving Average) is commonly used for time-series analysis, where \(p, d, q\) and \(P, D, Q, s\) are model parameters that tailor the forecasting to the system's specific patterns.Implementing predictive analytics in utility systems not only enhances reliability but also reduces operational costs by allowing for timely intervention instead of reactive fixes.
Case Studies in Analysis
Case studies provide real-world insights into the practical application of utility systems analysis techniques. By examining successful projects, you can understand best practices and innovative solutions.Consider a utility system optimization project at a manufacturing plant. The plant faced high energy costs due to inefficient systems. A thorough analysis revealed several insights:
- Power Monitoring: Installation of smart meters enabled accurate tracking of energy usage across different sections.
- Load Shifting: By re-scheduling energy-intensive processes to off-peak times, the plant reduced energy demand charges.
- Improved Insulation: Enhanced thermal insulation led to reduced heating and cooling needs.
In case studies, always pay close attention to the context in which a particular utility system operates. This ensures findings are applicable to similar scenarios and adjustments align with specific operational needs.
One fascinating case study involves a smart building initiative in a major city. The project employed a holistic approach by integrating all utility systems with a centralized control system. This enabled seamless communication between electrical, HVAC, and water systems.The project utilized IoT sensors and advanced analytics to achieve:
- Real-time monitoring and adjustments.
- Predictive maintenance alerts, preemptively addressing failures.
- Significant water savings through automated leak detection systems.
utility systems - Key takeaways
- Definition of utility systems in engineering: Integral part of engineering ensuring smooth operation and maintenance of primary systems and infrastructure.
- Utility systems explained: Complex network supporting non-core activities of a building, essential for maintaining operational flow and efficiency.
- Importance of utility systems in engineering: Backbone supporting industrial operations, residential areas, and modern infrastructure functionality.
- Utility systems engineering techniques: Design and optimization methods like energy auditing, computational fluid dynamics, and advanced control systems.
- Utility systems analysis: Techniques such as benchmarking, energy modeling, and simulation to evaluate efficiency and integrate with core processes.
- Impact on sustainability: Efficient systems reduce resource wastage, improve energy efficiency, and lower carbon footprints.
Learn faster with the 12 flashcards about utility systems
Sign up for free to gain access to all our flashcards.
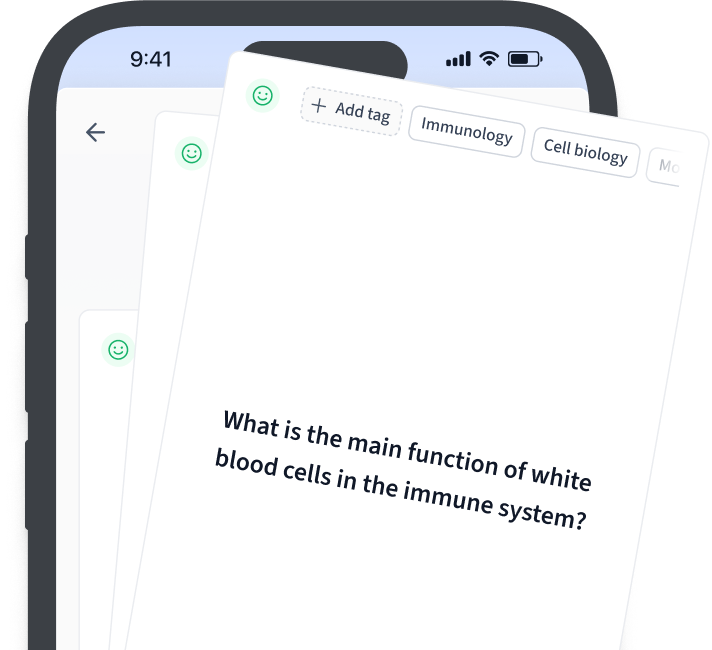
Frequently Asked Questions about utility systems
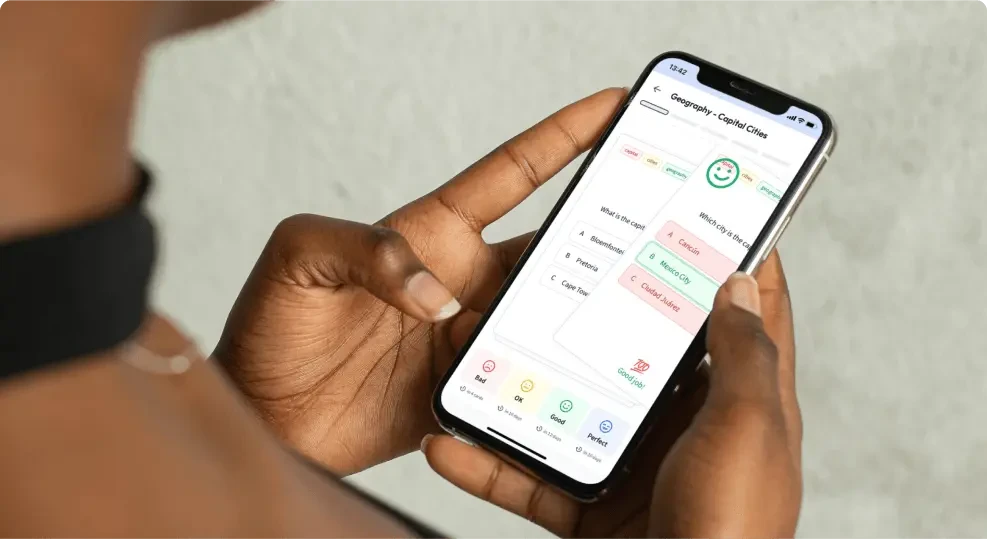
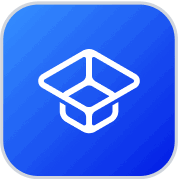
About StudySmarter
StudySmarter is a globally recognized educational technology company, offering a holistic learning platform designed for students of all ages and educational levels. Our platform provides learning support for a wide range of subjects, including STEM, Social Sciences, and Languages and also helps students to successfully master various tests and exams worldwide, such as GCSE, A Level, SAT, ACT, Abitur, and more. We offer an extensive library of learning materials, including interactive flashcards, comprehensive textbook solutions, and detailed explanations. The cutting-edge technology and tools we provide help students create their own learning materials. StudySmarter’s content is not only expert-verified but also regularly updated to ensure accuracy and relevance.
Learn more