Jump to a key chapter
Bridge Design Overview
The art and science of bridge design involve creating structures that can withstand various forces and provide safe passage across obstacles like rivers, valleys, or roads. A fundamental understanding of bridge design is crucial for aspiring engineers looking to make an impact in this domain.
Bridge Design Explained
Bridge design is a multifaceted engineering discipline focusing on creating structures that efficiently handle loads and stresses. When you design a bridge, several important factors must be considered to ensure the bridge's durability and effectiveness. These include:
Load: The weight that the bridge must support, including both the bridge's weight and any additional weight from vehicles or pedestrians.
To effectively distribute these loads, you use a variety of materials, such as steel, concrete, and wood, each providing distinct benefits depending on the design. For example, steel is often used for its strength and flexibility, while concrete is valued for its durability and low maintenance requirements.
Material | Properties |
Steel | Strong and flexible |
Concrete | Durable and low maintenance |
Wood | Lightweight and easy to work with |
Consider a short-span beam bridge, where the force calculation for a uniformly distributed load uses the formula \[w = \frac{M}{L}\] where w is the load per unit length, M is the moment, and L is the length of the beam. This simple calculation is pivotal in preliminary design stages.
In-depth knowledge of mathematical principles like Fourier transformations and finite element analysis plays a crucial role in advanced bridge design. Fourier transformations allow engineers to understand the cycles of forces acting on a bridge, such as vibrations from traffic. Finite element analysis is used to simulate how a bridge behaves under various conditions, offering insights that are crucial when designing long-span and complex bridges like cable-stayed bridges. Understanding these mathematical tools enables finer precision in design adjustments.
Importance of Bridge Design Principles
The principles of bridge design are essential for ensuring the safety and longevity of the structure. Bridge design must adhere to essential principles and standards for several reasons:
A well-designed bridge not only addresses immediate needs but can also mitigate future risks such as natural disasters or increased traffic loads.
Key principles in bridge design include:
Safety: The bridge must safely handle expected and unexpected loads throughout its life cycle. Efficiency: Optimal use of materials and construction methods to create cost-effective yet robust structures.
To achieve these principles, engineers follow design codes and guidelines, ensuring that bridges meet both regional specifications and international standards. The importance of these principles is often underscored by historical failures, where inadequate designs led to catastrophic outcomes. Adopting robust design methodologies and incorporating safety margins can avert such failures.
- Innovative materials
- Advanced computational analysis
- Rigorous safety testing
Exploring the balance between aesthetics and functionality is another layer within bridge design. Iconic bridges like the Golden Gate Bridge or the Tower Bridge demonstrate how aesthetic considerations can enhance the functionality, becoming landmarks while serving crucial infrastructure roles. The integration of art and engineering is particularly evident in designs blending ecological friendliness, such as grass-covered pedestrian bridges aiming to reconnect wildlife habitats.
Engineering Bridge Design Techniques
The field of bridge design is a cornerstone of civil engineering, focusing on constructing reliable passageways over obstacles. It combines scientific principles and innovative techniques to ensure these structures are both functional and lasting.
Structural Bridge Design Fundamentals
Designing a bridge involves several fundamental concepts that ensure stability and safety for its users. These include an understanding of loads, material properties, and structural integrity.
Structural Load: The forces that a bridge must support, typically including static loads (the bridge's own weight) and dynamic loads (vehicles, wind, etc.).
An engineer must balance these loads using different materials such as steel, concrete, or combinations thereof. Each material has distinct qualities; for instance, steel is known for its tensile strength, making it ideal for suspension bridges. Conversely, concrete offers compressive strength, suitable for arch and beam bridges. Calculating the loads and stresses efficiently is crucial. For example, the moment of force for a beam bridge under uniform load distribution is calculated using: \(M = \frac{wl^2}{8}\) where w is the load per unit length and l is the span length. These calculations guide you in choosing the right materials and designs to withstand expected forces.
Consider a suspension bridge, where the vertical cables support the deck by transferring the load to the bridge towers. The calculation involves determining the tension in these cables using equations such as \(T = \frac{wL}{2}\) where T is the tension, w is the load per unit length, and L is the span of the bridge.
While standard calculations are integral, advanced computational methods like Finite Element Analysis (FEA) expand design possibilities. FEA allows for detailed simulation of stress points and deflections under various loads, providing a more comprehensive analysis that can prevent design oversights. It is increasingly used to explore innovative materials like fiber-reinforced polymers, which offer high strength-to-weight ratios.
Innovations in Truss Bridge Designs
Truss bridges are characterized by a framework of interconnected elements forming triangular units. This design effectively manages compression and tension, offering efficient load distribution and enabling the use of lighter materials.
Truss: A framework, typically consisting of triangles, designed to support structures by distributing forces through its elements.
Recent innovations in truss bridge design involve improvements in geometry and material analysis that enhance durability and load-bearing capacity. By applying concepts like modular design, you can decrease construction time and improve maintenance efficiency. The K-truss and Warren truss are popular choices due to their simplicity and strength. Utilizing mathematical modeling, engineers can optimize the truss design. The stability of a simple truss can be verified using the equation: \(m = 2j - 3\) where m is the number of members and j is the number of joints. This equation ensures that the truss is statically determinate, which is crucial for efficient force distribution.
Advancements in software tools allow engineers to test thousands of truss configurations quickly, enabling the exploration of novel designs tailored for specific environmental conditions.
Exploring the integration of smart materials, such as shape memory alloys, offers exciting possibilities in truss bridge designs. These materials respond to environmental changes, potentially increasing a bridge's resilience to factors like temperature and wind changes. Their ability to self-adjust and 'heal' could redefine maintenance protocols, significantly extending the lifespan of these vital infrastructures.
Bridge Structural Analysis Methods
Bridge structural analysis is crucial in bridge design, ensuring that structures can safely withstand the loads and stresses they encounter. By applying various methods, engineers evaluate the performance of bridge components and materials, addressing safety, durability, and efficiency.
Tools for Bridge Structural Analysis
To accurately assess and analyze bridge structures, engineers use specialized tools and software. These tools facilitate precise modeling, simulation, and calculation of forces, moments, and deflections. Some common tools include:
Finite Element Analysis (FEA): A computational method that simulates how a bridge behaves under varied loading conditions, providing insights into stress distribution and potential failure points.
Using software such as FEA, you can break down complex structures into simpler, manageable units or elements to perform detailed analysis. This method helps identify stress concentrations and optimize material use. Another frequently employed tool is the Structural Analysis Program (SAP2000), which allows for the detailed assessment of structural behavior using comprehensive modeling capabilities. This software supports various structural elements like trusses, frames, and plates.
- Simulation Tools: Allow exploration of different load scenarios.
- CAD Software: Offers 3D modeling for visualization and precise design adjustments.
Consider a simple truss bridge. Using SAP2000, you input the geometry and material properties to simulate loads. The analysis might show tension in top chords and compression in bottom chords, suggesting designs changes to enhance stability. A typical equation to verify a simple truss is \(m = 2j - 3\), ensuring it's statically determinate.
Engineers continually innovate bridge analysis tools. Recently, machine learning and AI have introduced predictive models that assess structural health and predict potential failures. Predictive maintenance technologies use data from sensors embedded in bridges to forecast deterioration and avert disasters. These developments are reshaping how engineers approach bridge lifecycle management, making future analyses more proactive and precise.
Case Studies in Bridge Design
Studying real-world bridge designs sheds light on the practical applications of structural analysis and provides valuable insights into tackling engineering challenges. Analyzing case studies fosters a deeper understanding of how different designs respond to environmental and societal demands.
Every bridge project offers unique insights into geography, materials, and technology, making case studies an invaluable resource for continuous learning.
One such case study is the Millau Viaduct in France, a cable-stayed bridge that spans the Tarn River Valley. Its design required overcoming geological and wind challenges through innovative solutions, including using high-performance concrete and steel cables for enhanced flexibility.The Tacoma Narrows Bridge disaster is a historical case that illustrates the critical importance of proper aerodynamic analysis. Early design oversights led to its collapse in 1940 merely months after its opening. Understanding the failure mechanisms prompted the development of more robust engineering standards in bridge design and construction.
Bridge | Key Challenge | Solution |
Millau Viaduct | High winds | Aerodynamically stable deck design |
Tacoma Narrows | Aerodynamic forces | Improved analysis techniques |
Exploring the recent seismic retrofit project of the Golden Gate Bridge provides insights into how engineers enhance existing structures. This project integrated state-of-the-art seismic technologies to improve resilience against earthquakes, crucially updating the design without affecting its iconic appearance. Such adaptations highlight engineering ingenuity in balancing contemporary safety standards with historical preservation.
Applying Bridge Design Principles
Understanding and applying the fundamental principles of bridge design ensure that bridges are safe, functional, and durable. Each design incorporates mathematical, scientific, and material considerations to effectively meet the challenges posed by diverse environments.
Real-world Bridge Design Applications
Bridge design in the real world involves synthesizing practical insights with theoretical knowledge to create effective structures. Engineers tackle complex geographical and environmental challenges with innovative solutions that prioritize safety, cost-efficiency, and longevity.
Cable-Stayed Bridge: A type of bridge where cables directly connect the towers and the deck, offering both aesthetic appeal and efficient load distribution.
Consider the Akashi Kaikyō Bridge in Japan, the world's longest suspension bridge. The design incorporates aerodynamic enhancements to withstand typhoons, using equations to calculate wind pressure such as: \[P = 0.6 \times C_d \times V^2\] where P is the pressure, C_d is the drag coefficient, and V is the wind speed.
In practice, modern bridge designs often incorporate sustainable materials and construction techniques to reduce environmental impact while extending the bridge's service life.
The application of bridge design also extends to retrofitting older bridges. The retrofitting of the Forth Road Bridge in Scotland exemplifies this, where engineers assessed needs for increased traffic loads and implemented carbon fiber reinforcement to strengthen the bridge without adding excessive weight.
Challenges in Structural Bridge Design
When designing bridges, engineers face numerous challenges that require careful consideration and innovative solutions. These include environmental factors, material limitations, and unexpected stressors.
Load-Bearing Capacity: The maximum load a bridge can handle safely without experiencing failure or structural compromise.
To address these challenges, you must calculate various stress factors and apply appropriate design principles. For example, thermal expansion is a critical factor, especially in regions with vast temperature fluctuations. Formula-based calculations, like those determining material expansion \( \text{ΔL} = \text{αLΔT} \) where ΔL is the change in length, α is the coefficient of thermal expansion, and ΔT is the temperature change, guide engineers in choosing materials that accommodate these changes. Environmental impacts such as erosion and seismic activity present further complications, necessitating additional design considerations and practices like installing seismic isolators or riverbank stabilizers.
- Seismic forces require detailed dynamic analysis.
- Erosion control involves sediment management lifecycle planning.
Further examination of bridge design challenges reveals the impact of climate change, with rising sea levels and increased storm intensity posing significant threats. Adaptive design features, such as elevated structures and floating components, are being explored to ensure that future bridges can endure these conditions. The integration of climate models into the design process is becoming essential in anticipating long-term environmental changes and securing bridge viability for future generations.
bridge design - Key takeaways
- Bridge Design Principles: Focused on creating durable structures that withstand loads and stresses. Key principles include safety, efficiency, and the optimal use of materials.
- Engineering Bridge Design: Combines scientific principles and innovative techniques to ensure functional and lasting structures spanning obstacles like rivers and valleys.
- Structural Bridge Design: Involves understanding loads, material properties, and structural integrity to ensure stability and safety.
- Truss Bridge Designs: Feature frameworks of interconnected elements forming triangles, optimizing load distribution and permitting the use of lighter materials.
- Bridge Structural Analysis: Ensures structures safely withstand loads through methods like Finite Element Analysis (FEA) for detailed simulations of stress points.
- Bridge Design Explained: Incorporates aspects like load calculations, material selection (steel, concrete, wood), and structure forms (arch, beam, suspension) for effective bridge design.
Learn faster with the 12 flashcards about bridge design
Sign up for free to gain access to all our flashcards.
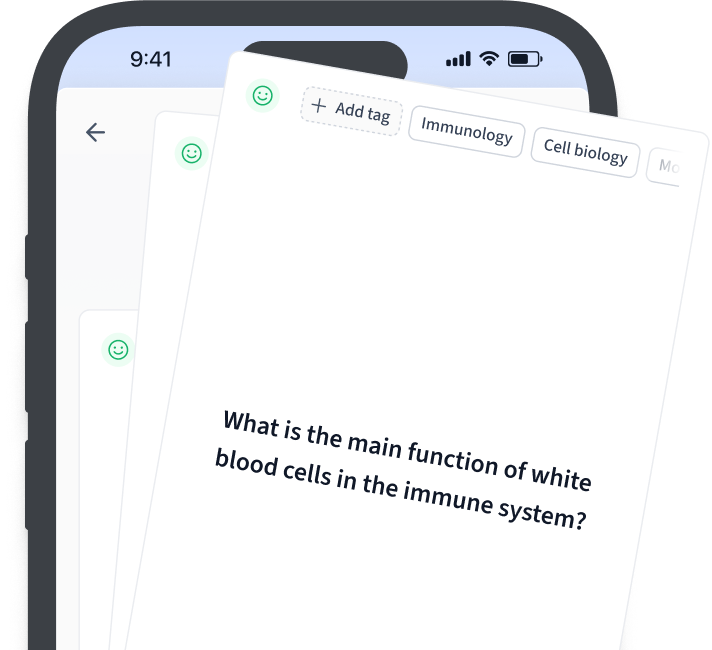
Frequently Asked Questions about bridge design
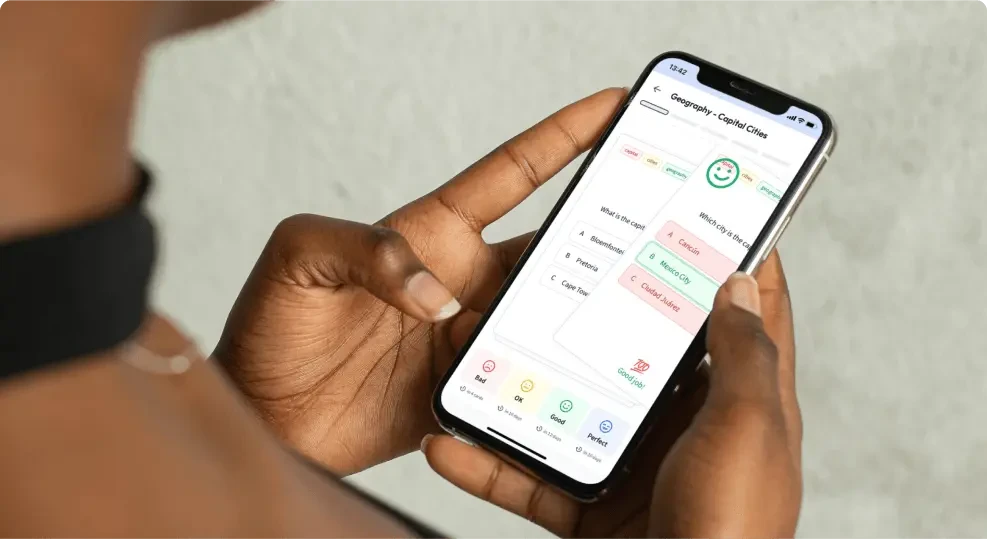
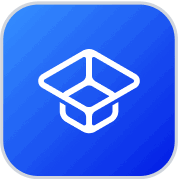
About StudySmarter
StudySmarter is a globally recognized educational technology company, offering a holistic learning platform designed for students of all ages and educational levels. Our platform provides learning support for a wide range of subjects, including STEM, Social Sciences, and Languages and also helps students to successfully master various tests and exams worldwide, such as GCSE, A Level, SAT, ACT, Abitur, and more. We offer an extensive library of learning materials, including interactive flashcards, comprehensive textbook solutions, and detailed explanations. The cutting-edge technology and tools we provide help students create their own learning materials. StudySmarter’s content is not only expert-verified but also regularly updated to ensure accuracy and relevance.
Learn more