Jump to a key chapter
Understanding the Joule Kelvin Expansion
Joule Kelvin Expansion, also known as a throttling process or a Joule-Thomson process, is a fascinating phenomenon in thermodynamics that you'll undoubtedly encounter during your engineering studies. This process demonstrates that a real gas will behave differently when subjected to certain conditions, expanding in a way that can either increase or decrease its temperature.A Joule Kelvin expansion is considered an adiabatic process, occurring without any heat being added or removed from the system. In this process, a real gas is forced through a constriction, like a valve or a porous plug, without any external work being done.
Breaking Down the Joule Kelvin Expansion Meaning
At this point, you might be wondering, "What exactly happens during a Joule Kelvin Expansion?" No need to worry, as we're about to dive deep into the microcosmos of molecules and the impacts of pressure and temperature change on their behavior. The physics behind this expansion is relatively straightforward. When a gas experiences a decrease in pressure, the molecules within it begin to spread out. Depending on the type of gas and its initial temperature, this internal rearrangement comes with an associated change in its internal energy and therefore, its temperature. However, it's essential to note that Joule Kelvin Expansion does not result in a temperature change for all gases. Most gases, such as air and nitrogen, exhibit a cooling effect. Still, other gases, like hydrogen and helium under standard room temperature, will warm up during expansion.A everyday example of Joule Kelvin expansion is the feel of a gas canister after it has just been used. Gas canisters often feel colder after being used due to the temperature drop during the expansion of the gas while it is being released.
Interestingly, the cooling effect in Joule Kelvin Expansion is utilised extensively in industrial applications, including the liquefaction process of gases in air separation plants, and even in cooling systems for scientific equipment and research!
Core Components of Joule Kelvin Expansion
The interesting aspects of this phenomenon can be attributed to three critical components:Pressure Drop | This is an essential ingredient of the Joule Kelvin expansion. Without a decrease in pressure, none of the other effects can take place. |
Temperature Change | The possible change in temperature that occurs will be contingent on the gas and its initial conditions prior to expansion. |
The Joule-Thomson coefficient | The Joule-Thomson coefficient is a term that encapsulates the amount of temperature change that occurs per unit decrease in pressure. It's calculated using the formula: \(\mu = \left(\frac{\partial T}{\partial P}\right)_H\) |
Differentiating between Joule Kelvin Expansion and Other Thermodynamic Processes
The Joule Kelvin expansion is often confused with the Joule expansion. While both share the Joule name, they are starkly different!- The Joule expansion is an isolated (no heat or work exchange) and free (no force exerted) expansion.
- On the other hand, the Joule Kelvin process is a throttling (force exerted to cause expansion) procedure without an exchange of heat.
Think of a bottle of sparkling water. Without opening it, no gas escapes, and the temperature remains static. That's akin to Joule expansion. Once you twist the cap and hear the "hiss" of gas escaping, that's Joule Kelvin Expansion in action!
The Science Behind Joule Kelvin Expansion
In the world of thermodynamics, Joule Kelvin Expansion is a significant process that vividly showcases the characteristic behaviour of real gases under certain situations. This practice is termed after the physicists James Prescott Joule and William Thomson (Lord Kelvin), who extensively researched the properties of gases and their behaviour under different conditions.The Enthalpy Joule Kelvin Expansion Explained
The Joule Kelvin Expansion significantly depends on the enthalpy of the system. In thermodynamics, enthalpy is the total potential energy of a system plus the product of its volume and pressure. The formula is represented as: \[ H = U + PV \] where:- \(H\) signifies enthalpy,
- \(U\) stands for internal energy, and
- \(PV\) represents the product of pressure-volume work done.
How Energy is Conserved in Joule Kelvin Expansion
As this process is an isenthalpic one, an intriguing interplay of energy conservation can be observed. When gas expands, it does work on its surroundings, implying a decrease in internal energy. However, during the Joule Kelvin Expansion, while internal energy can drop, the energy spent in doing work is compensated for by the reduction in the product of pressure and volume, thereby securing the overall balance. In other words, the energy used to increase the system's volume tends to cause a drop in the gas's temperature. On the flip side, if a gas's initial internal energy is more due to intermolecular attractions, the internal energy can increase (as a result of the reduced proximity of gas particles post-expansion). In return, the temperature of the gas may witness an elevation. Therefore, the Joule-Thomson Coefficient is critical as it establishes the relationship between the change in temperature and pressure.Why Enthalpy Matters in Joule Kelvin Expansion
Enthalpy plays a key role in this process because it fundamentally captures the energy changes during the Joule Kelvin expansion. The reason is simple. Given that the process doesn't allow heat exchange with the surroundings and no external work is being done, the only source of energy that can be modified is the system's internal energy. The critical aspect to bear in mind is that in the Joule Kelvin expansion, while there is an alteration in enthalpy components, the total enthalpy remains intact. This point is often missed when first learning about Joule Kelvin expansion, which is why you should always remember: the process may initiate changes in pressure, volume, and temperature- but the total enthalpy of your system will always stay the same. This conclusion, appropriately named the 'First Law of Thermodynamics,' is an important benchmark for every study related to energy processes.Practical Illustrations of Joule Kelvin Expansion
Through thorough observations and understanding of Joule Kelvin Expansion, you might realise that this thermodynamic process is not confined within academic books, but is well-established and active within your daily experiences and the functioning of numerous technological appliances.Joule Kelvin Expansion Examples in Everyday Life
To deepen your understanding of this process, it is essential to link theoretical knowledge with examples from your everyday life. This enables you to observe how the principles of thermodynamics come into play and solidify your understanding of the effect. Aerosol Cans: When you use a deodorant, note how the can feels cold after a few seconds of spray. This is due to Joule Kelvin Expansion at play. The propellant gas inside the can is at high pressure; when you spray, the gas undergoes a rapid pressure drop as it exits into the atmosphere. Using the Joule-Thomson effect, the released gas cools due to the expansion, resulting in a cold sensation on the aerosol can. Refrigeration Systems: The refrigerators at your home also use the Joule Kelvin expansion for cooling purposes. The refrigerants used in these appliances experience a sudden drop in pressure as they pass through a tiny orifice, throttling the incoming high-pressure gas. This sudden drop in pressure leads to cooling of the gas, thus reducing the temperature inside the refrigerator. Car Tyres: Have you ever noticed that a car tyre becomes warm after driving for a while? This is also an example of Joule Kelvin Expansion. As you drive, the air inside the tyre expands due to heating from friction. When the tyre interfaces with the road, slight pressure changes within the tyres can cause the air inside to expand, increasing the tyre's temperature in the process.Engineering Scenarios Featuring Joule Kelvin Expansion
Besides everyday situations, Joule Kelvin expansion plays a considerable role in various engineering applications, particularly in areas such as cryogenics and gas liquefaction plants. Gas Liquefaction: One of the significant applications of Joule Kelvin expansion is in the field of gas liquefaction. Industries that require liquid gases (like liquid nitrogen and oxygen used in various medical and chemical processes) utilise the Joule Kelvin expansion process. When the gas is allowed to expand through a throttle valve from a region of high pressure to an area of lower pressure, the subsequent cooling, if extreme enough, can cause the gas to liquify. Cryogenics: In the realm of ultra-low temperature study, cryogenics, Joule Kelvin expansion plays a pivotal role. When a gas mixture is subjected to adiabatic expansion, it results in significant cooling. Cryogenic systems such as those used in research institutes, MRI scanning machines, and superconducting magnet cooling applications rely heavily on the cooling effect observed in Joule Kelvin Expansion. The Linde Cycle: Also known as the Hampson-Linde cycle, this approach employs Joule Kelvin expansion to realise gas liquefaction. Here, the working gas is compressed, following which heat is extracted via a heat exchanger. The gas is then expanded isenthalpically using a throttle valve. The resultant cold gas from expansion is then routed back to the heat exchanger, thus forming a cyclic process that results in continuous cooling and gas liquefaction. From aerosols to gas liquefaction plants, the Joule Kelvin Expansion and its remarkable impacts form the foundation of several everyday occurrences and industrial operations, offering invaluable insight into the practical application of thermodynamics in real-world engineering scenarios.Discovering the Versatility of Joule Kelvin Expansion
Joule Kelvin Expansion is not just a cornerstone of thermodynamics; it also offers immense flexibility due to its unique nature. This process is at the heart of several phenomena and technologies, from the cooling system in your refrigerator to the cryogenics in sophisticated medical equipment. This versatility stems from its fundamental properties and intricate interplay with the laws of thermodynamics, making it a fascinating subject to explore further.Is Joule Kelvin Expansion Reversible? Uncovering the Truth
The concept of reversibility in thermodynamics is a significant one. A thermodynamic process is termed 'reversible' if it can return to its original state without leaving any net change in either the system or its surroundings. Understanding whether a process is reversible or irreversible is vital to assess its efficiency and its feasibility in various applications. Joule Kelvin Expansion, is in fact, an irreversible process. It might appear confusing because the definition of Joule Kelvin Expansion outlines it as an isenthalpic (or constant enthalpy) process, suggesting a return to the initial state is feasible. However, the crux lies in the way this expansion occurs. When gas expands in a Joule-Kelvin Expansion, it does so without any external work being done. This phenomenon is often visualised using a throttle valve or a tiny porous plug that the gas passes through. There is no mechanism to produce external work as gas expands - the energy changes that occur are entirely internal. This expansion scenario is markedly different from an ideal, reversible expansion. The latter would often involve a piston-like system where the gas expansion drives the piston and performs external work. As the system could now interact with its surroundings, it would be possible- theoretically- to revert this process. However, in the case of Joule Kelvin Expansion, the lack of interaction with the outside environment during expansion means the process cannot be reversed without externally manipulating the system. An external compressor would be specifically required to recompress the gas to its original state, an element absent during the initial expansion.Impact of Reversibility on an Engineering System
The inherent irreversibility of Joule Kelvin Expansion significantly impacts its efficiency within any engineering system. Compared to hypothetical, fully reversible processes, irreversible processes always involve some loss of efficiency due to factors like friction, dissipative effects, and energy lost as waste heat. The impact is not limited to thermodynamics alone. In terms of engineering systems, irreversible processes like Joule Kelvin Expansion tend to have lower Carnot efficiency. Named after the French scientist Sadi Carnot, this parameter represents the maximum possible efficiency that any heat engine involving a heat transfer process can achieve. Specifically, the Carnot efficiency is given by the expression: \[ \eta_C = 1 - \frac{T_{cold}}{T_{hot}} \] where:- \(T_{cold}\) is the temperature of the cold reservoir, and
- \(T_{hot}\) is the temperature of the hot reservoir.
Applying Joule Kelvin Expansion in Different Fields
Joule Kelvin Expansion is applied across various fields, with its principles helping to drive innovations and industrial processes. Based on the principle of a sudden decrease in the pressure of a real gas resulting in cooling, this fundamental physics and engineering principle is utilised in fields as diverse as climate control, industrial manufacturing, medical imaging, and space technology.Important Joule Kelvin Expansion Applications
Joule Kelvin Expansion applications are significant and varied. Many phenomena around you are a manifestation of this expansion and the corresponding cooling — including some that you encounter in your daily life. Domestic and Industrial Refrigeration: The refrigeration systems you use at home or in industrial settings operate based on Joule Kelvin Expansion. As high-pressure refrigerant gas passes through an expansion valve to the lower pressure area, rapid cooling occurs, reducing the temperature inside the refrigerator. Medical Imaging: Modern medical systems, such as MRI and NMR machines, employ superconducting magnets that require intense cooling. The requisite cooling is facilitated by the Joule Kelvin Expansion principle. Cryogenics: Joule Kelvin expansion is central to cryogenics, the science of producing and managing very low temperatures. It is used to achieve and maintain these ultra-low temperatures required in scientific experiments and high-tech manufacturing. Space Applications: Joule Kelvin Expansion is also invaluable in space applications. For instance, it is used in the cooling systems of spacesuits to regulate the astronaut's body temperature in the harsh extremes of space.The Role of Joule Kelvin Expansion in Energy Systems
Joule Kelvin Expansion plays a crucial role when it comes to energy systems. One of the pivotal areas where Joule Kelvin Expansion is essential is in heat pump and refrigeration systems where it is used to achieve and maintain the desired cooling effect. The basic principle behind a heat pump system involves three key steps:- Compression: The refrigerant gas is compressed, causing it to heat up due to the work done in compressing it.
- Condensation: The hot, higher-pressure gas is then cooled, usually by an air or water heat exchanger, causing it to condense back into a liquid state.
- Throttling: This liquid refrigerant then goes through a throttling process, leading to Joule Kelvin Expansion. It expands rapidly, reducing its temperature and turning it back into a low-pressure gas.
Benefits of Understanding Joule Kelvin Expansion in Engineering Careers
Understanding Joule Kelvin Expansion can offer major value for your engineering career, regardless of your specific field. In Mechanical Engineering, it underpins systems such as heat pumps and refrigeration, while in Chemical Engineering, it facilitates gas liquefaction processes. For Electrical and Electronics Engineers, grasping Joule Kelvin Expansion can provide significant insights into thermal management, important for electronic system design. In areas like Biomedical Engineering, understanding these principles can prove vital in designing low-temperature technologies like MRI machines. Gradually, with the growing demand for energy-efficient technologies and the quest for minimising global warming potential, thermal engineering - and, by extension, the understanding of principles like Joule Kelvin Expansion - is becoming increasingly important in shaping future innovation across diverse fields. Thus, an in-depth comprehension of this area is not only intellectually rewarding but can also open doors to a vast landscape of diverse professional opportunities.Mastering the Joule Kelvin Expansion Formula
Understanding Joule Kelvin Expansion rests heavily on getting to grips with a specific formula. This mathematical representation of Joule Kelvin Expansion allows us to calculate the change in temperature of an ideal gas as it expands under constant enthalpy conditions.An Overview of the Joule Kelvin Expansion Formula
The Joule Kelvin Expansion is defined by the formula: \( \mu_{J.T} = \left(\frac{\partial T}{\partial P}\right)_H \)
- \( T \) is the temperature,
- \( P \) is the pressure, and
- \( \mu_{J.T} \) is the Joule Thompson coefficient, which characterises the cooling or Joule Kelvin effect.
For instance, for helium gas at room temperature, the Joule-Thomson coefficient is negative, meaning that upon expansion, the gas will warm up instead of cooling.
Step by Step Guide to Apply the Joule Kelvin Expansion Formula
Mastering the Joule Kelvin Expansion formula requires understanding steps to calculate this key coefficient. Step 1: Identify the properties of the gas, including its specific heat at constant volume and pressure \( C_v \) and \( C_p \), and the gas constant \( R \). Step 2: Compute the gas's coefficient of volume expansion \( \beta \) and its isothermal compressibility \( \kappa \). Step 3: Input these values into the formula for the Joule Murdock-Kelvin Coefficient \( µ_{JT} \): \[ \mu_{J.T} = \frac{1}{C_p} \left( T \left(\frac{\partial \beta}{\partial T}\right)_p - \beta + T \beta \kappa \right) \] Step 4: Use the \( µ_{JT} \) value in the Joule Kelvin Expansion formula to calculate the temperature change with a pressure change under isenthalpic conditions. Always remember to check the units of the variables you're working with. Ensure all are consistent throughout the calculations to avoid errors.Common Mistakes to Avoid when Using Joule Kelvin Expansion Formula
Working with the Joule Kelvin Expansion Formula often involves some common pitfalls that can result in incorrect calculations: Ignoring Ideal Gas Assumptions: For low pressures and high temperatures, real gases closely resemble ideal gases. However, for high pressures or low temperatures, deviations are significant and should be accounted for with the help of a compressibility factor. Misinterpretation of \( \mu_{J.T} \): The Joule-Thomson coefficient is not always positive. A negative \( \mu_{J.T} \) implies heating upon expansion. Such a situation occurs for monatomic gases like helium and neon at room temperature. Incorrect Temperature Calculations: When a gas expands, it doesn't mean it will always cool down. For some gases, at particular initial temperatures, there might be no cooling (inversion temperature) or even heating. It's essential to use the Joule-Thomson coefficient correctly to predict temperature changes. Overlooking Units: Thermodynamic calculations should always be conducted with great attention to units. Pay close attention to the units of each term to avoid incorrect results. Understanding, applying, and navigating the Joule Kelvin Expansion Formula and the math behind it is integral to fully understand this important physical principle. With this understanding, you can leverage this principle in various applications and deepen your comprehension of thermodynamics.Joule Kelvin Expansion - Key takeaways
- Joule Kelvin Expansion is an isenthalpic process, meaning it happens under constant enthalpy - the energy spent in doing work is offset by a reduction in the product of pressure and volume.
- Enthalpy is a critical component in Joule Kelvin Expansion as it captures the energy changes during the process. Even though there are alterations in enthalpy components, the total enthalpy remains intact.
- Joule Kelvin Expansion examples include aerosol cans (the cooling sensation after spraying), refrigeration systems (sudden drop in pressure leads to cooling), and car tyres (warming up due to pressure changes from driving).
- Engineering applications of Joule Kelvin Expansion are found in gas liquefaction, cryogenics, and the Linde Cycle, illuminating the practical application of thermodynamics in real-life engineering scenarios.
- Despite the constant enthalpy, Joule Kelvin Expansion is an irreversible process. The lack of external interaction during expansion makes it impossible for the process to be reversed without externally influencing the system. This contributes to its lower Carnot efficiency compared to a hypothetically fully reversible process.
Learn faster with the 12 flashcards about Joule Kelvin Expansion
Sign up for free to gain access to all our flashcards.
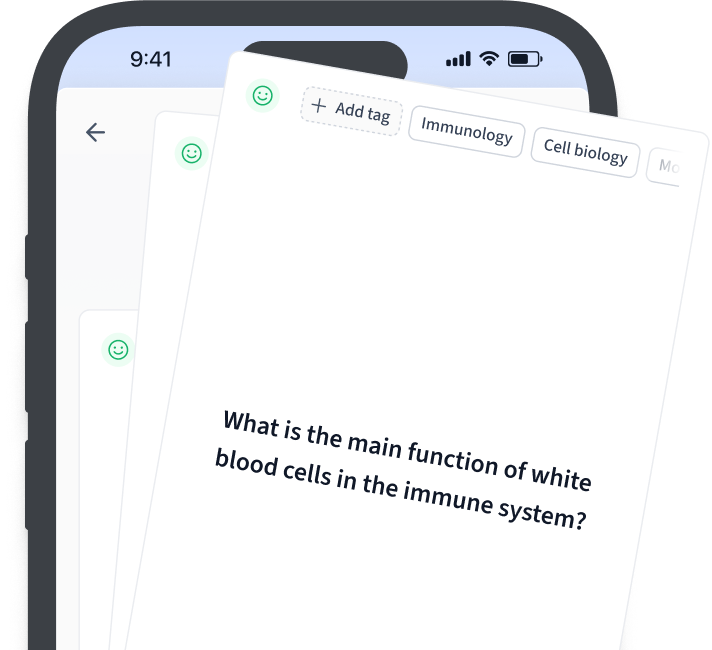
Frequently Asked Questions about Joule Kelvin Expansion
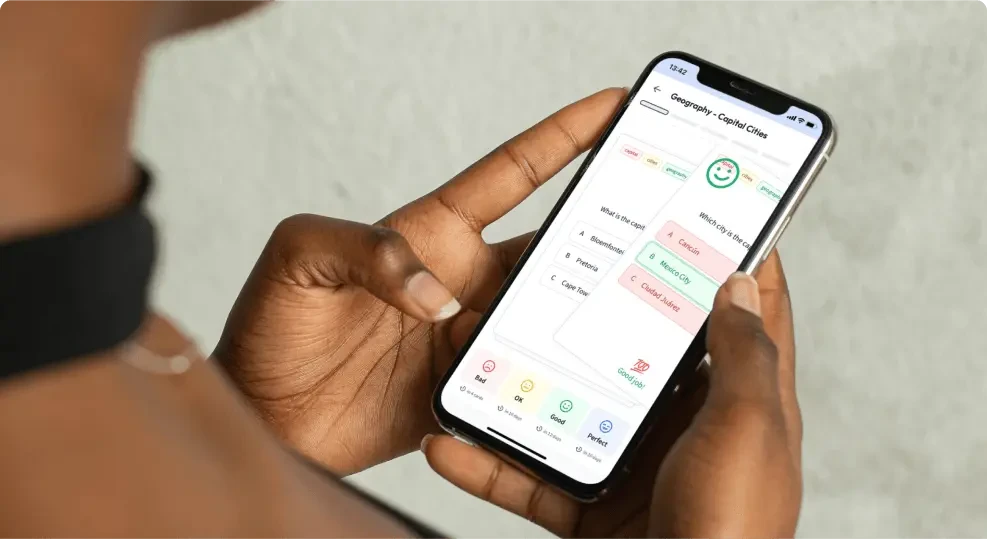
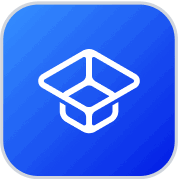
About StudySmarter
StudySmarter is a globally recognized educational technology company, offering a holistic learning platform designed for students of all ages and educational levels. Our platform provides learning support for a wide range of subjects, including STEM, Social Sciences, and Languages and also helps students to successfully master various tests and exams worldwide, such as GCSE, A Level, SAT, ACT, Abitur, and more. We offer an extensive library of learning materials, including interactive flashcards, comprehensive textbook solutions, and detailed explanations. The cutting-edge technology and tools we provide help students create their own learning materials. StudySmarter’s content is not only expert-verified but also regularly updated to ensure accuracy and relevance.
Learn more