Jump to a key chapter
Understanding Osmotic Pressure
As future engineers, it's crucial that you understand the concept of osmotic pressure, a fundamental principle in the world of Science and Engineering. Always wondering why a raisin left in a glass of water becomes plump or why our fingers become wrinkled after prolonged immersing in water? The answer is osmotic pressure. So, let's dive right in?
Osmotic Pressure Meaning
Osmotic pressure is a colligative property of solutions that is influenced by the number of solute particles, not the type of solute particles. It can be thought of as the molecular 'force' exerted by water molecules as they attempt to move, or 'osmose', from an area of lower solute concentration to an area of higher solute concentration through a semi-permeable membrane.
Simple Explanation of Osmotic Pressure
Imagine you have two containers of water separated by a semi-permeable membrane. In the first container, you have pure water and in the second, you've mixed in some salt. Water molecules would naturally want to move from the area of lower solute concentration (the pure water side) to the area of higher concentration (the salt water side) until the concentrations on both sides are equal. The pressure that the water molecules exert to move across the membrane, that's what we call osmotic pressure.
Osmotic Pressure and Engineering Thermodynamics
In the field of engineering thermodynamics, osmotic pressure plays a big role. It falls under the category of non-mechanical work interactions, similarly to diffusion and capillary action. Here’s an interesting fact:
The osmotic pressure increases as the difference in solute concentration increases. This principle can be applied in operations like reverse osmosis for water purification where a high pressure is applied to the higher solute concentration side, forcing water to move back to the lower concentration side, hence purifying it.
The Principle Behind Osmotic Pressure
Osmotic pressure is underlined by two fundamental principles: the tendency of molecules to move from an area of higher concentration to lower concentration and the property of semi-permeability of cell membranes. Here is an enumerated discussion on these principles:
- The tendency of molecules, especially in a liquid or gas state, to spread out or disperse until uniform concentration is achieved. We call this process Diffusion.
- A semi-permeable membrane allows certain particles to pass through while blocking others. In the case of osmotic pressure, the semi-permeable membrane allows water to pass through but blocks the solute particles.
As an example, consider a raisin immersed in a glass of water. The skin of the raisin acts as a semi-permeable membrane. Inside the raisin, the concentration of solute (composed of various sugars and other organic compounds) is higher than the pure water outside. So, naturally, the water from outside will move into the raisin causing it to become plump.
In representing the osmotic pressure mathematically, we use the following equation:
\[ \Pi = iCRT \]In this formula:
- \(\Pi\) represents the osmotic pressure
- \(i\) refers to the van't Hoff factor, which is the number of particles into which the solute separates in solution
- \(C\) denotes the molar concentration of the solute
- \(R\) is the ideal gas constant
- \(T\) is the absolute temperature
Understanding the osmotic pressure and the principles behind it aids in the design of numerous engineering applications, such as water purification systems, pharmaceutical drug delivery, and a variety of biological-based engineering processes.
Osmotic Pressure in Everyday Life
From biology to geology, environmental science to your own kitchen, osmotic pressure can be found at work in many diverse contexts. By understanding osmotic pressure, you can appreciate the mechanisms that power numerous natural phenomena and man-made systems and leverage this knowledge to improve engineering outcomes.
Osmotic Pressure Examples
Osmotic pressure is a natural process with a multitude of examples, impacting diverse areas of life and science. These include cellular functions, the preservation of food, and even the operation of certain technologies. Below are a couple of instances where osmotic pressure plays an essential role.
Osmotic Pressure in Natural Phenomena
In nature, osmotic pressure is a crucial factor in many biological processes. For example, plant cells utilise osmotic pressure to maintain their rigidity. The cell wall of a plant cell is semi-permeable, permitting water to move in and out. When water moves into the cell due to the higher concentration of solute particles inside, it causes the cell to swell, creating a pressure against the cell wall referred to as turgor pressure.
In human biology, osmotic pressure is crucial in maintaining bodily homeostasis. For instance, our kidneys regulate the levels of water and salts in our bloodstream through an osmotic process. Too many salts in the blood can increase osmotic pressure, causing water to move from the body cells (where salt concentration is lower) into the bloodstream.
Osmotic Pressure in Man-Made Systems
Man-made systems also frequently employ osmotic pressure. For example, in food preservation, a high concentration of sugar or salt is used to create a high osmotic pressure environment. This process, known as osmotic dehydration, causes water to move out of microorganisms that might spoil the food, effectively preserving it.
Additionally, osmotic pressure is a principle used in reverse osmosis water purification systems. These systems utilise a high pressure to overcome osmotic pressure and force water from a highly concentrated solution to a less concentrated one, effectively purifying the water from contaminants.
Osmotic Pressure Applications in Engineering
Osmotic pressure plays a crucial role in many engineering sectors, from chemical engineering to environmental engineering. The technology of desalination plants, drug delivery systems, and nutrient absorption in the human digestive system are a few examples of its fascinating applications.
Effectiveness and Limitations
The effectiveness of using osmotic pressure in engineering applications relies heavily on the system's design factors. These factors include the type of semi-permeable membrane used, the difference in solute concentration on either side of the membrane, and the pressure applied to the system.
Design Factor | How it impacts effectiveness |
Type of semi-permeable membrane | The material characteristics, such as permeability and strength under pressure, can determine the efficiency and longevity of the system. |
Difference in solute concentration | A larger difference increases the osmotic pressure and consequently the potential for work or movement. |
Applied pressure | In systems like reverse osmosis, the higher the applied pressure, the greater the balance against the osmotic pressure, permitting more efficient purification. |
However, operating systems based on osmotic pressure also have certain limitations. For example, semi-permeable membranes can become clogged, reducing system efficiency. Additionally, if the applied pressure exceeds the membrane's pressure tolerance, it can lead to system failures. Understanding these effectiveness factors and limitations can provide a robust foundation for designing and perfecting osmotic pressure-based engineering applications.
Mathematical Representation of Osmotic Pressure
As engineers, the fundamental concepts of osmotic pressure are essential not just in theory but also through their mathematical representations. These formulas enable you to calculate the osmotic pressure given certain variables and are thus useful for practical applications.
Understanding the Osmotic Pressure Formula
The mathematical representation of osmotic pressure is often expressed in terms of the van't Hoff equation. The formula for osmotic pressure (\( \Pi \)) is:
\[ \Pi = iCRT \]This formula directly showcases hoe the osmotic pressure is influenced by various factors. Here:
- \( \Pi \) denotes the osmotic pressure.
- \( i \) is the van't Hoff factor, which indicates the number of particles the solute splits into in the solution.
- \( C \) is the molar concentration of the solute.
- \( R \) is the ideal gas constant, which is 0.0821 L atm / (mol K) when pressure is measured in atmospheres.
- \( T \) is the absolute temperature measured in Kelvin.
The formula demonstrates that osmotic pressure is directly proportional to the molar concentration of the solute, the temperature, and the van't Hoff factor. Understanding this relationship becomes pivotal while working with systems where osmotic pressure plays a crucial role.
Derivation of the Osmotic Pressure Formula
Under ideal conditions, the gas pressure is related to its concentration and temperature, as given by the Ideal Gas Law:
\[ PV = nRT \]where, \( P \) is the pressure, \( V \) is the volume, \( n \) is the number of moles, \( R \) is the gas constant, and \( T \) is the absolute temperature. This equation can be rearranged to express pressure as:
\[ P = \frac{nRT}{V} \]For a solution where solute particles can move freely, the pressure they would exert if enclosed in a similar volume is the osmotic pressure. This gives us the osmotic pressure equation when we replace \( P \) with \( \Pi \), \( n \) with the moles of solute, and \( V \) with the volume of the solution:
\[ \Pi = \frac{nRT}{V} \]Further by replacing \( n/V \) (moles of solute per volume of solution) with molar concentration \( C \), we arrive at the final form of the osmotic pressure equation:
\[ \Pi = CRT \]Practical Usage of the Osmotic Pressure Formula
The osmotic pressure formula is instrumental in assessing and designing systems where solute concentration variance needs to be managed. Here are a few examples:
- In medical treatments such as dialysis, where the toxin concentration needs to be managed, medical professionals use the osmotic pressure formula to design the dialysis process, ensuring efficient toxin removal while maintaining the necessary nutrients.
- In food processing, osmotic pressure can be manipulated to control food properties, like moisture content. Food scientists use the formula to control the dehydration process, contributing to product shelf life, safety, and texture.
- In the domain of water treatment, the formula is used to engineer optimal conditions for reverse osmosis systems, allowing for pure water extraction from seawater or wastewater.
By understanding and applying the osmotic pressure formula, you can manage and improve engineering solutions where the osmotic pressure can be leveraged. Therefore, the mathematical knowledge of osmotic pressure can equip you with the needed tools to optimise numerous real-world processes. However, one must always be mindful of the assumptions and limitations of the van't Hoff equation, especially where ideal-dilute conditions do not strictly apply.
Specific Types of Osmotic Pressure
Osmotic pressure is ubiquitous, with several distinct types based on the nature of particles that contribute to it. Two important forms you should know about are colloid osmotic pressure and hydrostatic pressure, which play significant roles in biological and physical systems.
What is Colloid Osmotic Pressure?
An essential type of osmotic pressure is colloid osmotic pressure (COP), also known as oncotic pressure. This pressure is exerted by proteins, also termed colloids, that are too large to cross semipermeable membranes. In biological contexts, COP is vital in sustaining fluid balance within and outside cells.
Human blood plasma, for example, contains large proteins such as albumin, which cannot pass through the capillary walls due to their size. These undiffusible solute particles cause a difference in solute concentration between the inside and outside of the blood vessel, resulting in an osmotic gradient. This gradient drives the movement of water from areas of lower protein concentration to areas of higher protein concentration, creating colloid osmotic pressure.
COP plays a critical role in the fluid balance between the bloodstream and tissue spaces. If COP within blood vessels is too high compared to the tissue spaces, or interstitial fluid, edema, or water retention in tissues, may occur. Similarly, low COP within blood vessels can lead to dehydration. Therefore, understanding and controlling COP can be crucial in managing certain health conditions.
Colloid Osmotic Pressure (COP): The osmotic pressure contributed by proteins (colloids) that cannot cross a semipermeable membrane. COP helps maintain fluid balance in biological systems.
Differences between Standard and Colloid Osmotic Pressure
Standard osmotic pressure and colloid osmotic pressure, while both being forms of osmotic pressure, are caused by different types of solute particles. Here are the main differences:
- Standard osmotic pressure is caused by solute particles that can be any solute (such as sugar or salt), whereas colloid osmotic pressure is specifically due to colloids, like proteins.
- The solutes contributing to standard osmotic pressure can typically pass through semi-permeable membranes, while the colloids causing COP are usually too large for this.
- Standard osmotic pressure governs much of the water movement between different solute concentration areas, while COP plays a more specific role in managing fluid balance, especially in biological systems.
Hydrostatic Pressure vs Osmotic Pressure
Hydrostatic pressure and osmotic pressure, though often considered together when examining fluid dynamics, are distinct and result from different mechanisms. Hydrostatic pressure is the pressure exerted by a fluid at equilibrium at a given point within the fluid, due to the force of gravity. It increases with depth and varies in fluid systems based on factors like fluid density and gravitational pull.
Osmotic pressure, as you now know, is due to the movement of water across a semipermable membrane when there is a difference in solute concentration. Both hydrostatic and osmotic pressure play complementary roles in nature, particularly within biological systems. For example, within capillaries, the difference between them determines fluid exit and entry, thereby affecting the fluid balance within tissues.
Hydrostatic Pressure: Refers to the pressure exerted by a fluid at equilibrium due to the gravitational pull. It affects fluid dynamics and is involved in fluid balance in natural and engineered systems.
Comparative Analysis of Hydrostatic and Osmotic Pressure
Osmotic pressure and hydrostatic pressure share similarities but have core differences that differentiate their roles in fluid dynamics. Here are some comparative aspects:
- Cause: Osmotic pressure arises from the difference in solute concentration across a semi-permeable membrane, while hydrostatic pressure is due to gravity acting on a fluid.
- Action: Osmotic pressure drives the movement of water from an area of lesser solute concentration to a greater one, while hydrostatic pressure moves fluid from an area of greater pressure to lesser pressure.
- Effects in biological systems: Both pressures influence the fluid balance in capillaries, with hydrostatic pressure tending to drive fluid out of capillaries and osmotic pressure tending to pull fluid in. The net effect of these pressures regulates fluid exchange between body compartments.
Understanding the functions and effects of these distinct yet interactive pressures is crucial, as they are integral to many natural and engineered systems, including managing water flow in plants, regulating blood volume in humans, and designing complex hydraulic systems in engineering. By manipulating these pressures, biological systems maintain homeostasis, and engineers can create efficient drainage and irrigation systems.
The Science Behind Osmotic Pressure
Osmotic pressure is a crucial concept that bridges the realms of biology, chemistry, physics, and engineering. It is a phenomenal force governing the flow of solvent particles across semipermeable membranes, due to differences in solute concentrations. This principle underpins numerous natural activities, and it is also harnessed extensively in engineering designs for water treatment systems, among other applications.
How Osmotic Pressure Works at the Molecular Level
To grasp how osmotic pressure operates, let's delve into its mechanisms at the molecular level. The process starts when a semipermeable membrane separates a solution from a pure solvent. In this scenario, the membrane will allow solvent but not solute particles to pass through.
A principle known as diffusion asserts that particles move spontaneously from areas of higher concentration to lower concentration until equilibrium is reached. This is an attempt to equalise the concentrations on either side of the membrane. However, for the pure solvent, the concentration of solute particles is lower than that received from the solution. Therefore, the solvent molecules naturally migrate towards the solution side, seeking to equalise the concentration.
For osmosis, the flow of solvent particles across the membrane due to differences in concentration leads to a volume change on both sides of the membrane. An increase in volume on the solution side and a decrease on the solvent side occur, causing a difference in liquid levels across the membrane. This volume change exerts a force, termed 'osmotic pressure', which resists the further movement of solvent particles.
However, the process does not go on indefinitely—the solvent migration ceases when the pressure induced by the increasing volume on the solution side equals the initial driving force for solvent movement. At this point, the osmotic pressure equals the force driving the solvent transfer.
For example, imagine a U-shaped tube blocked in the middle by a semipermeable membrane. If you filled one side with pure water and the other with saltwater, the water molecules would begin migrating towards the saltwater side. The water levels could be measured on either side of the membrane. The osmotic pressure would be exerted when the water level on the saltwater side goes up. At equilibrium, the pressure resulting from the increased water level would balance the initial driving force for the water transfer.
Osmotic Pressure and its Relationship with Temperature and Pressure
Two crucial factors influencing osmotic pressure are temperature and pressure. The osmotic pressure is directly proportional to both these parameters, as expressed in the mathematical formula:
\[ \Pi = iCRT \] Where:- \( \Pi \) = Osmotic Pressure
- \( i \) = van't Hoff factor indicating the number of particles the solute splits into
- \( C \) = Molar concentration of the solute
- \( R \) = Ideal gas constant
- \( T \) = Absolute temperature in Kelvin
This implies that if the temperature or pressure increases, the osmotic pressure will also increase, provided all other variables remain constant. Conversely, a decrease in temperature or pressure will diminish osmotic pressure. This phenomenon can be observed in real-world applications; for instance, if a reverse osmosis system operates at higher pressures, it will generate higher osmotic pressure, leading to more efficient purification.
The Thermodynamics of Osmotic Pressure and Its Significance in Engineering
The principles of thermodynamics are essential in explaining how osmotic pressure arises from molecular interactions. The thermodynamic theory states that a system will move towards a state of minimum potential energy. In terms of osmosis, this is achieved when the concentration of solute molecules becomes equal on both sides of the semipermeable membrane, thereby reaching equilibrium.
Engineers often have to consider the effects of osmotic pressure, especially when designing systems like water treatment plants, desalination units, or even biological implants like artificial kidneys. Here, having a comprehensive understanding of the thermodynamics of osmotic pressure is vital. Designing such systems requires an adept manipulation of pressures, temperatures, and material properties to optimise energy efficiency and performance.
Moreover, thermodynamics principles are also leveraged to develop mathematical models dictating the operational performance of such systems. For instance, the van't Hoff equation mentioned earlier is a thermodynamic equation used to calculate the osmotic pressure. Therefore, a firm grip on the underlying thermodynamic principles can significantly enhance the proficiency in designing and optimising such osmotic pressure-dependent systems.
One noteworthy application where such thermodynamic principles are exploited is in food preservation. Osmotic pressure is utilised to increase the shelf-life of foods in a process known as osmotic dehydration. In this process, food is soaked in a hypertonic solution of sugar or salt. The high solute concentration outside the food causes water inside the food to diffuse out, thereby reducing its water activity. This action helps inhibit microbial growth, effectively increasing the food's shelf-life. Such an example beautifully illustrates the interplay of osmotic pressure and thermodynamics in solving real-world challenges.
Osmotic Pressure - Key takeaways
- Osmotic pressure is a key factor in many biological processes, such as maintaining rigidity in plant cells and maintaining bodily homeostasis in humans.
- Man-made systems also often use osmotic pressure, such as food preservation via osmotic dehydration and reverse osmosis water purification systems.
- The mathematical representation of osmotic pressure is often expressed through the van't Hoff equation, which shows that osmotic pressure is directly proportional to the molar concentration of the solute, the temperature, and the van't Hoff factor.
- Colloid osmotic pressure, or oncotic pressure, is exerted by proteins (colloids) that cannot cross a semipermeable membrane and is crucial in sustaining fluid balance within and outside cells.
- Hydrostatic pressure, distinct from osmotic pressure, is the pressure exerted by a fluid at equilibrium due to gravity. These two types of pressure play complementary roles, particularly within biological systems.
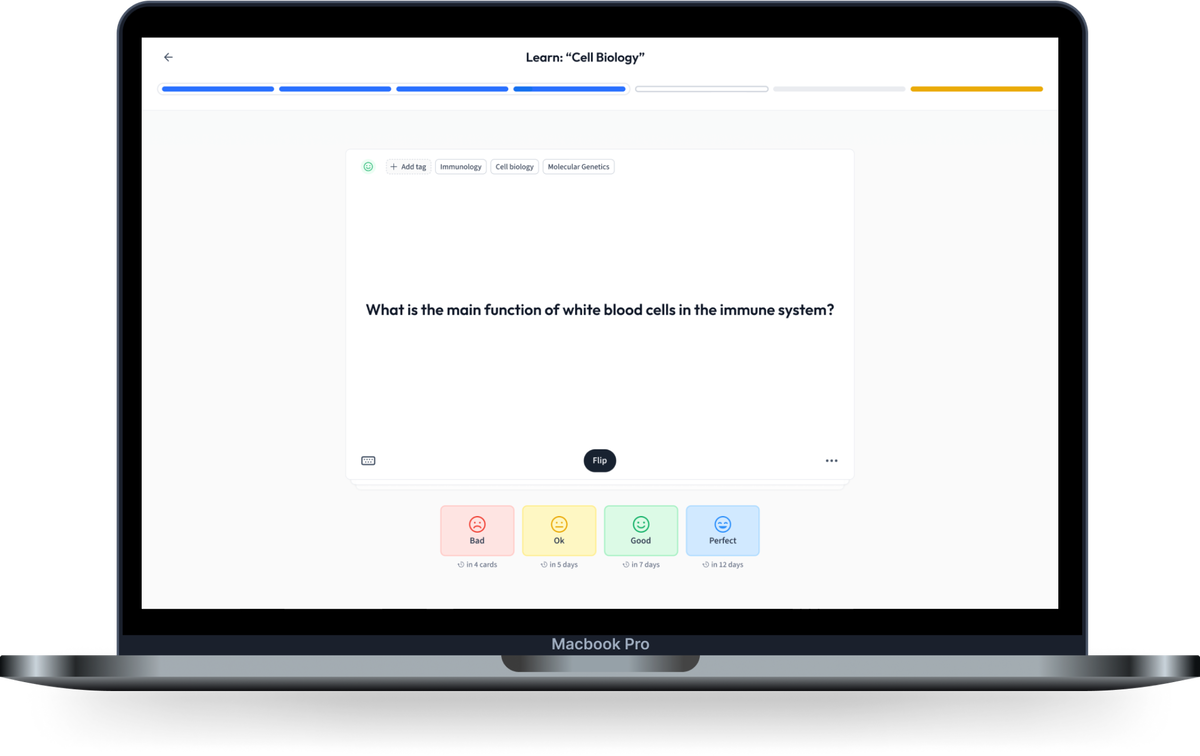
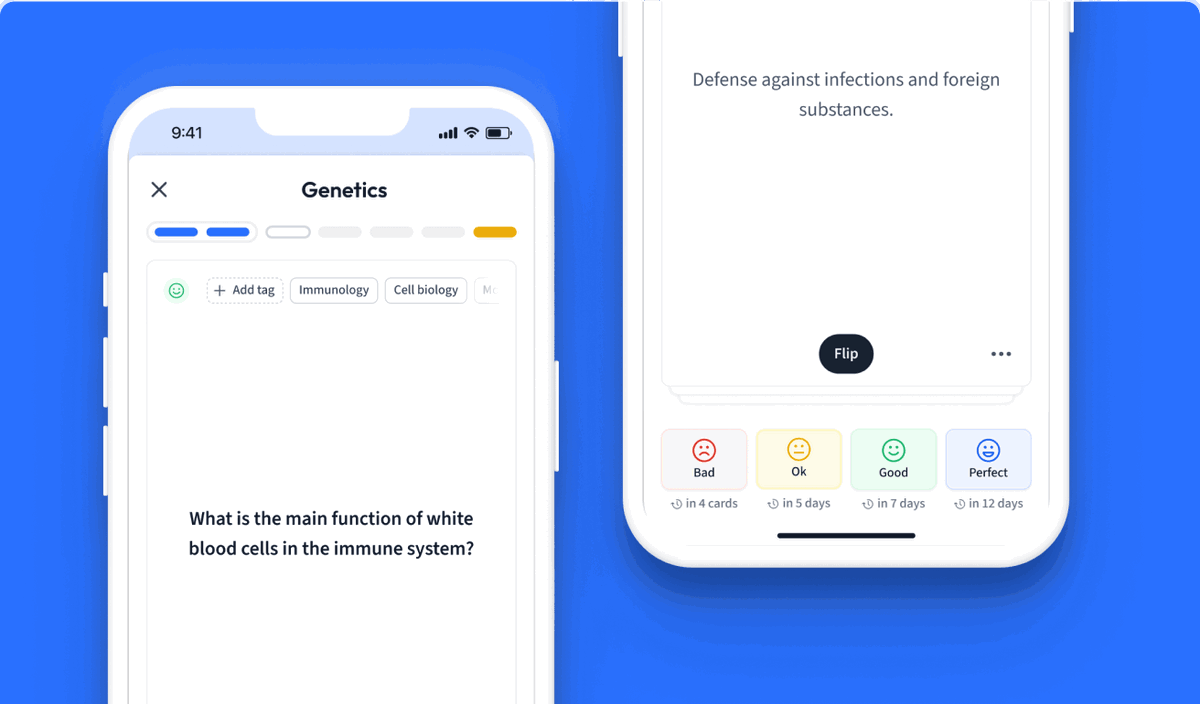
Learn with 15 Osmotic Pressure flashcards in the free StudySmarter app
We have 14,000 flashcards about Dynamic Landscapes.
Already have an account? Log in
Frequently Asked Questions about Osmotic Pressure
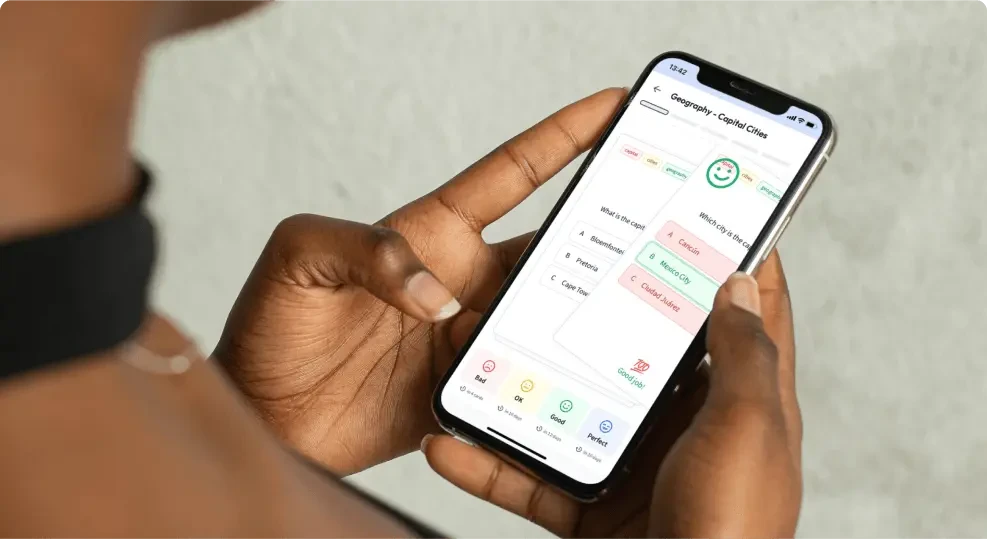
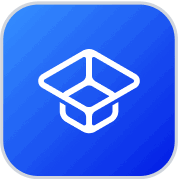
About StudySmarter
StudySmarter is a globally recognized educational technology company, offering a holistic learning platform designed for students of all ages and educational levels. Our platform provides learning support for a wide range of subjects, including STEM, Social Sciences, and Languages and also helps students to successfully master various tests and exams worldwide, such as GCSE, A Level, SAT, ACT, Abitur, and more. We offer an extensive library of learning materials, including interactive flashcards, comprehensive textbook solutions, and detailed explanations. The cutting-edge technology and tools we provide help students create their own learning materials. StudySmarter’s content is not only expert-verified but also regularly updated to ensure accuracy and relevance.
Learn more