Jump to a key chapter
Understanding the Thermodynamic System Meaning
Understanding the thermodynamic system can significantly enhance your understanding of engineering. At its most basic, a thermodynamic system is a section of the universe that has been set aside for study. It involves elements in physics and engineering, especially relating to the conversion and transfer of energy.A thermodynamic system is defined as a part of the universe set aside for studying properties and phenomena related to the transfer and conversion of thermal energy.
Origins of the Thermodynamic System
The concept of the thermodynamic system has its roots in the mid-19th century, when the laws of thermodynamics were first being established. It was during this time that scientists recognised the need for a well-defined space or section of the universe to examine thermal processes. The study of thermodynamics initially took off with the invention of the steam engine. Early scientists and engineers such as James Joule, Sadi Carnot, and Lord Kelvin, among others, rallied to understand and optimize the performance of these machines by studying the principles of heat and energy transfer within them.For an example, a steam engine is a thermodynamic system. When coal is burned, it heats up the water in the boiler, producing steam. The energy produced is then transferred to do useful work, such as moving a piston or turning a wheel.
Key Concepts in the Thermodynamic System Meaning
There are several key concepts when it comes to understanding the thermodynamic system meaning.- System Boundary: The system boundary is the imaginary surface that separates the system from its surroundings.
- Types of Systems: There are mainly three types of thermodynamic systems. The Open System where matter and energy can cross the system boundary, Closed System where only energy can cross the boundary, and the Isolated System where neither energy nor matter can cross the boundary.
- State of a System: The state of a system is defined by its properties. Properties can be either intensive (not dependent on the size of the system like temperature) or extensive (dependent on the size of the system like volume).
Interpreting the Thermodynamic System in Real-World Studies
The principles of thermodynamic systems mainly find applications in practical engineering problems and real-world scenarios. Whether it's designing a refrigerator, planning an efficient energy conversion process, or understanding the functioning of the human body, the concept of the thermodynamic system is quite relevant.On a larger scale, even the Earth can be considered as a thermodynamic system. Scientists often classify Earth as an open system, given it exchanges both energy (in the form of heat and light from the Sun) and matter (like meteorites entering the Earth's atmosphere) with its surroundings.
Breaking Down Thermodynamic System Examples
Let's delve deeper and break down some specific examples of thermodynamic systems. Through examining different types of systems and real-world case studies, you can gain a clearer understanding of how these principles apply in practice.Commonly Used Thermodynamic Systems
Various examples of thermodynamic systems are commonly used in different fields. In classical mechanics, for instance, a gas confined within a cylinder by a piston constitutes a widely-used thermodynamic system. This system's state is typically described by properties such as pressure, volume, and temperature.System | Description |
Gas inside a cylinder | This is a common example of a closed system as the gas does not leave but its temperature and pressure can change with the addition of heat. |
Car Engine | An example of an open system where both matter (air, fuel) and energy (heat, work) cross the boundary. |
Thermos Flask | This is an example of an almost isolated system where neither matter nor energy is exchanged with the surroundings. |
Real Life Example of Thermodynamic Systems
Thermodynamics isn’t limited to just theoretical applications; its principles are reflected in everyday scenarios. A refrigerator is a prime example. When you make use of a refrigerator, a sequence of thermodynamic processes occur - isothermal (constant temperature) and adiabatic (no heat transfer) transitions - that result in cooling or freezing your food.Refrigerator: Within the refrigerator, a refrigerant (usually a fluid) travels through a cycle of evaporation and condensation. This refrigerant absorbs heat from the refrigerator's interior during evaporation and releases it outside during condensation. Therefore, the refrigerator interior cools down, preserving the food items inside. This is a prime example of a thermodynamic system in real-life.
Case Studies: Thermodynamic System Examples in Action
Now, let's look at some case studies, where we have applied the principles of thermodynamics to understand and solve real-world problems. One such case relates to power plants. Power generation plants harness different types of thermodynamic cycles, with the most common being the Rankine cycle. This cycle is composed of four main processes: Isentropic compression, Isobaric heat addition, Isentropic expansion, and Isobaric heat rejection.During the Rankine cycle, water is pressurized and vaporized using heat from a source (like burning coal). The high-pressure steam then drives a turbine, which runs a generator to produce electricity. The steam is cooled and condensed back into water before restarting the cycle.
Exploring Thermodynamic System Applications
To truly appreciate the scope and importance of thermodynamic systems, let's explore some of their practical applications. These applications range widely, covering a plethora of industries and even permeating our day-to-day lives. They underscore the importance of a strong foundational understanding of thermodynamics.Thermodynamic System Applications in Industry
Thermodynamic principles and systems permeate a plethora of industries, underpinning processes that are fundamental to their operation. They are indispensable in sectors like power generation, refrigeration, automotive, aerospace, and even the IT industry. Power generation plants, such as thermal power plants, operate on thermodynamics. They use heat energy, often from burning fossil fuels, to generate steam which drives turbines to produce electricity. This process involves a series of thermodynamic transformations and the entire power plant can be modelled as a thermodynamic system. In the automotive industry, the internal combustion engines found in most vehicles are prime examples of thermodynamic systems. Combustible mixtures of fuel and air are ignited causing an increase in temperature and pressure, which is utilised to perform work on the pistons, setting the vehicle in motion. In the realm of refrigeration and air conditioning, thermodynamic processes play a crucial role. Here, heat is intentionally transferred from colder to hotter regions, contrary to the natural flow of heat. This is achieved using refrigerants that undergo a thermodynamic cycle. Furthermore, the role of thermodynamics is not constrained to just traditional industries. In the rapidly evolving IT industry, thermodynamics plays a crucial role in cooling data centres. Timely and efficient heat removal from servers is of utmost importance to ensure their efficient operation and increase their lifespan. Table summarising applications:Industry | Thermodynamic System Application |
Power Generation | Conversion of thermal energy into mechanical work to generate electricity. |
Automotive | Internal combustion engines utilising fuel-air mixtures for vehicle propulsion. |
Refrigeration and Air Conditioning | Manipulation of heat transfer to maintain desired temperature conditions. |
IT Industry | Cooling mechanisms for servers in data centres. |
Thermodynamic System Applications in Everyday Life
The principles of thermodynamic systems are omnipresent in our daily lives, even if we don't explicitly realise it. From the refrigerators cooling our drinks to the boilers heating our water, these appliances are essentially thermodynamic systems at work. For example, in your refrigerator, a refrigerant absorbs heat from inside the fridge, cooling the contents. This heat is then transferred outside, leaving the interior cool. The process known as \( \text{refrigeration cycle} \), is a typical example of a thermodynamic system. Your modern, energy-efficient home is another example of an everyday thermodynamic system. It is a form of a closed system where heat energy is managed to make the dwelling more comfortable. In the summer, heat energy is released out of your home to make it cooler and the reverse happens in the winter, wherein heat is conserved inside your home to make it warmer. The automobile you drive to work every day? Its engine is an open thermodynamic system. Fuels are combusted to generate heat energy, which then gets converted into mechanical energy, driving the movement of the vehicle. Not to mention, the human body itself is a thermodynamic system. Energy intake (through food) and energy expenditure (through physical activities) are constantly balanced to maintain our body temperature, metabolic processes, and overall homeostasis.Potential Future Applications of Thermodynamic Systems
The applicability of thermodynamics is not just limited to contemporary mechanics or appliances, but also extends to the future advancements in technology and industry. They underpin innovations that could enable a more sustainable and energy-efficient future. In the future, we might see more widespread use of thermoelectric devices, which convert waste heat into electricity using thermodynamic principles. These devices have the potential to revolutionise power generation and create more sustainable energy systems. Another exciting potential application is in the field of quantum thermodynamics. As our understanding of quantum physics deepens, researchers are exploring the potential of quantum heat engines and refrigerators that could have high efficiencies and miniaturisation capabilities. This could have significant implications for future technologies, including quantum computing. In the race to create more efficient solar cells, researchers are leveraging thermodynamics to optimise their processes. Solar-thermodynamic systems that combine photovoltaic cells and thermodynamic cycles for electricity production are being developed. These systems have the potential to increase solar conversion efficiency and contribute towards greener energy future. All these potential applications underscore the limitless possibilities that a robust understanding of thermodynamics can unlock in the future. Despite being a centuries-old science, it remains as relevant as ever, underpinning the advances in today's technology while paving the way for tomorrow's breakthroughs.Decoding the Thermodynamic System Formula
Understanding the formulae associated with thermodynamic systems is crucial to unlocking their full potential and effectively applying them in practice. The formulae of thermodynamics encompass everything from heat transfer and energy conservation to entropy and enthalpy. In this section, we'll delve into the basics of the thermodynamic system formulas, their applications in calculations, and dive deeper into understanding the variety of formulas present in thermodynamics.Basics: An Overview of Thermodynamic System Formula
At the heart of thermodynamics lie fundamental laws that govern the interactions of heat, work, and energy. These laws give rise to a series of thermodynamic formulae that are integral to determining system behavior. The First Law of Thermodynamics, also known as the law of energy conservation, is represented as \(\Delta U = Q - W\). Here,- \(\Delta U\) denotes the change in internal energy of the system.
- \(Q\) stands for the heat added to the system.
- \(W\) represents the work done by the system.
Application of Thermodynamic System Formulas in Calculations
Thermodynamic formulas find extensive applications in diverse fields, ranging from large-scale industrial calculations to specialised academic research. For instance, the First Law of Thermodynamics calculations are used to determine the energy requirements for different processes, contributing to increased efficiency and reduced costs in industries like power generation and automobile manufacturing. By utilising the Second Law of Thermodynamics, engineers can calculate the maximum possible efficiency of heat engines, or ascertain the energy potential of a process before it’s carried out, ensuring that resources aren’t wasted on unfeasible projects. In chemical industry, enthalpy calculations are often undertaken to predict reaction outcomes. The calculation of enthalpy changes allow scientists to know beforehand whether a reaction will release or absorb heat, an important factor in ensuring safety and cost-effectiveness. Finally, the Third Law of Thermodynamics, whilst having less direct applications, sets the foundation for calculating absolute entropies of substances, an essential aspect in thermodynamic data compilations and thermodynamic software.Understanding the Different Thermodynamic System Formulae
In addition to the basic formulas derived from the laws of thermodynamics, there are numerous other thermodynamic equations essential for studying different systems and processes. Take for example, the Carnot cycle. It is an ideal thermodynamic cycle that gives the maximum possible efficiency of a heat engine. Its efficiency is given by \[\eta = 1 – \frac{T_C}{T_H}\], where \(T_C\) is the temperature of the cold reservoir and \(T_H\) is the temperature of the hot reservoir. Equations detailing property relations, such as the Maxwell Relations are also a pivotal part of thermodynamics. They are derived from the second derivatives of thermodynamic potentials and provide useful means to relate various thermodynamic properties together. Yet another important set of formulas in thermodynamics stems from the concept of adiabatic processes. For instance, for an adiabatic process involving an ideal gas, the equation \(PV^{\gamma} = \text{constant}\) holds, where \(\gamma\) is the ratio of the specific heats of the gas. By gaining a comprehensive understanding of these formulas, it becomes possible to understand, predict, and modify the behaviour of thermodynamic systems across an array of applications. This understanding reveals why thermodynamics, though an old science, continues to play a pivotal role in developments of new technologies and solutions to complex engineering problems.Unravelling Thermodynamic System Types
Thermodynamics, the study of energy and its transformations, relies on the concept of a thermodynamic system - a certain amount of matter or a region in space upon which attention is focused during the study of a problem. Understanding the different types of thermodynamic systems is fundamental in engineering, as they each possess unique characteristics and consequently display different behaviours under various conditions.Classifying Different Thermodynamic System Types
In thermodynamics, systems are broadly classified into three categories: isolated, closed, and open systems. These definitions are based on the type of exchanges a system can have with its surroundings.An isolated system neither exchanges matter nor energy with its surroundings. In isolation, the total quantities of matter and energy remain constant. To visualise this, think of a thermos flask with a hot beverage inside - ideally, it neither gains nor loses heat or mass.
A closed system can exchange energy (in the form of heat, work, or both) but not matter with its surroundings. A pressure cooker provides an apt example - energy in the form of heat can escape, but its contents cannot.
An open system can exchange both matter and energy with its surroundings. Many real world systems operate as open systems like a refrigerator or a water boiler, which both exchange matter (water or refrigerant) and energy (heat) with their surroundings.
Comparison of Thermodynamic System Types
Each thermodynamic system comes with its unique attributes and are more suited for some forms of analysis over others. Here is a comparative look at their traits:System Type | Mass Transfer | Heat Transfer | Work Exchange |
Isolated | No | No | No |
Closed | No | Yes | Yes |
Open | Yes | Yes | Yes |
Unique Features of Various Thermodynamic System Types
The different types of thermodynamic systems provide fascinating opportunities for scientific exploration, each possessing unique features. An isolated system, for example, provides an almost perfect environment for examining the conservation of energy. For instance, considering the internal energy of an isolated system given by the formula \(\Delta U = Q - W\), since \(Q = W = 0\), \(\Delta U = 0\), which means the total internal energy stays constant. It’s important to note, however, that while energy is conserved in an isolated system, it's not necessarily evenly distributed or balanced. Closed systems, on the other hand, can be found all around us, from car engines to refrigerators. The concept of a closed system is particularly important when studying processes that involve the transfer of energy. Within a closed system, heat transfer can occur, and work can be done on or by the system, leading to unique energy exchange dynamics. Open systems are the most complex to study among the three types, considering they allow both mass and energy to flow across their boundaries. They're also the most common in real-world engineering scenarios. However, despite their complexity, open systems follow the principles set by the laws of thermodynamics. A familiar example is the 'Steady Flow Energy Equation' often used in the study of fluid dynamics in open systems. It is given by \(H_1 + C_1^2/2 + gZ_1 = H_2 + C_2^2/2 + gZ_2 + Q - W \) wherein H is the enthalpy, C the velocity, Z the height, Q the heat transfer and W the work done. In conclusion, understanding the different types of thermodynamic systems and their respective characteristics is crucial to effectively apply the principles of thermodynamics and navigate complicated engineering challenges. By scrutinizing their unique features, one can better comprehend the fundamental laws of energy and matter transformations and their diverse applications.Thermodynamic System - Key takeaways
- Thermodynamic System Meaning: Thermodynamic systems are typically described by properties such as pressure, volume, and temperature. Thermodynamics isn't just limited to theoretical applications; its principles are reflected in everyday scenarios.
- Thermodynamic System Examples: Gas inside a cylinder, car engine, and thermos flask are examples of closed, open, and almost isolated thermodynamic systems, respectively. Other examples are the human body, the refrigeration cycle in a refrigerator, and the Rankine cycle in power plants.
- Thermodynamic System Applications: Thermodynamic systems are indispensable in sectors like power generation, refrigeration, automotive, aerospace, and even the IT industry. They are also present in daily appliances like refrigerators and boilers, and even in the human body itself.
- Thermodynamic System Formula: The process of heat transfer can be represented by the formula \( Q = mc\Delta T \). The First Law of Thermodynamics can be represented as \(\Delta U = Q - W\), and The Second Law of Thermodynamics introduces a new property called Entropy (S), and it is given by \(dS = \frac{dQ_{rev}}{T}\).
- Thermodynamic System types: These include open, closed, and isolated systems. Power generation plants represent a series of thermodynamic transformations, making the entire power plant a thermodynamic system. The internal combustion engines in vehicles are examples of open thermodynamic systems.
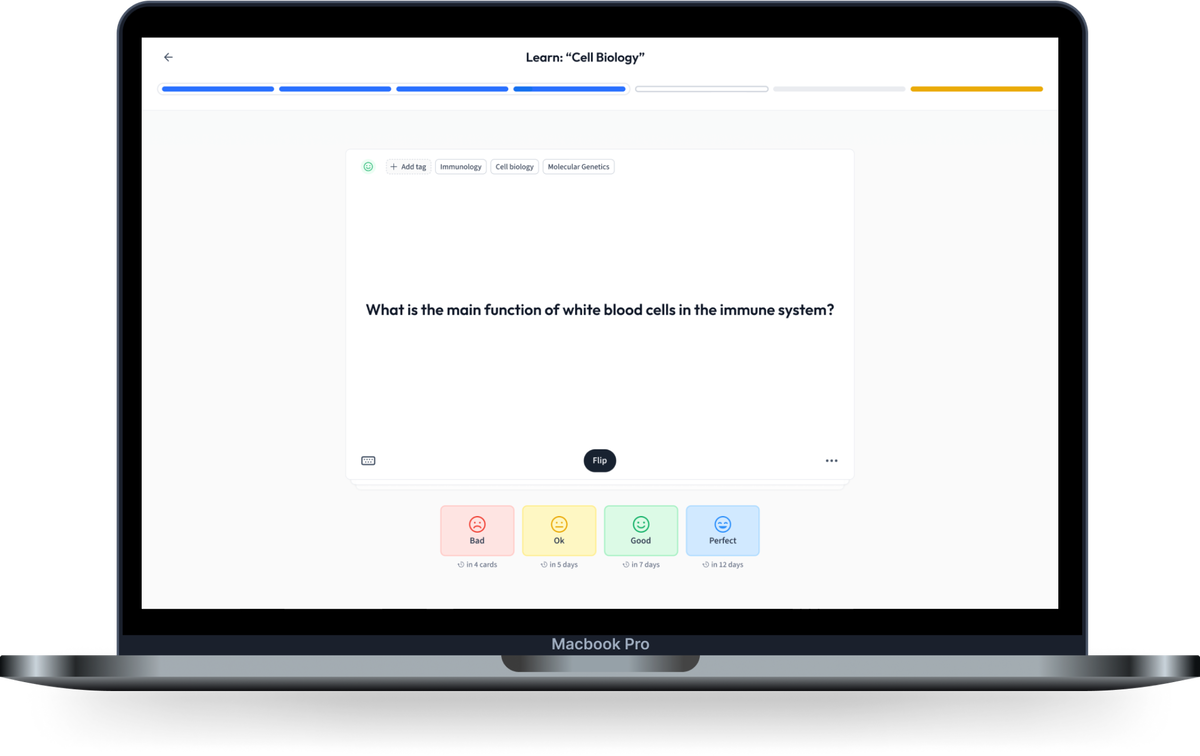
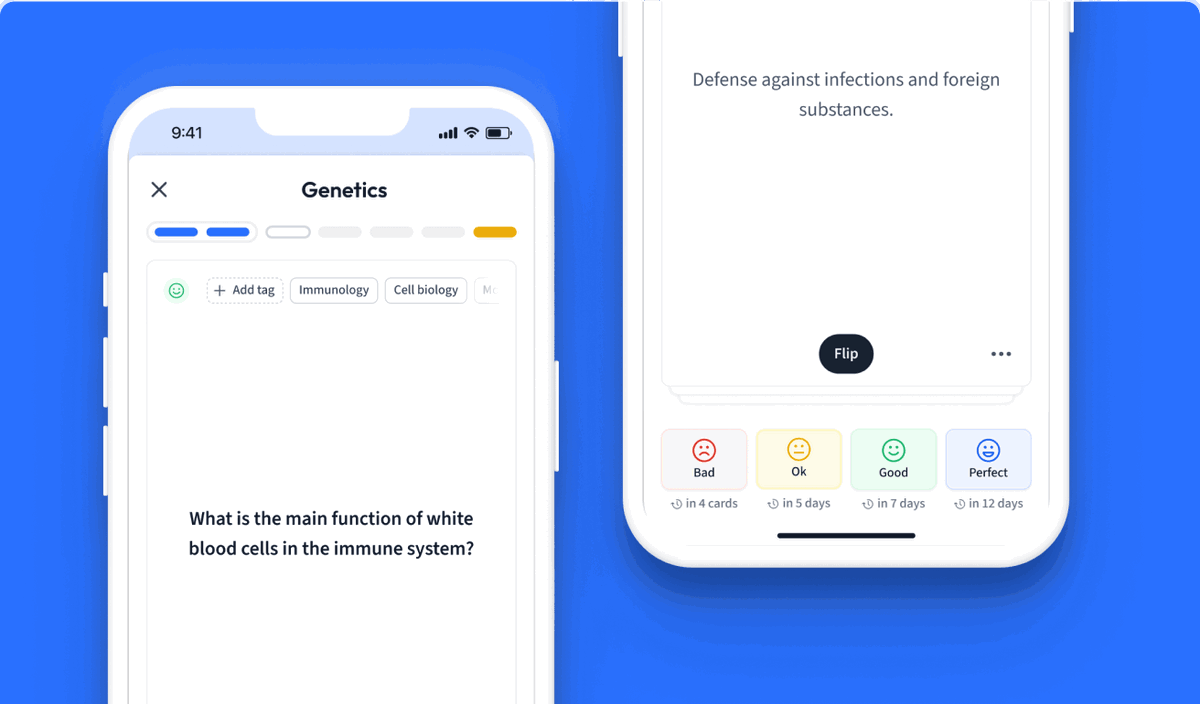
Learn with 15 Thermodynamic System flashcards in the free StudySmarter app
Already have an account? Log in
Frequently Asked Questions about Thermodynamic System
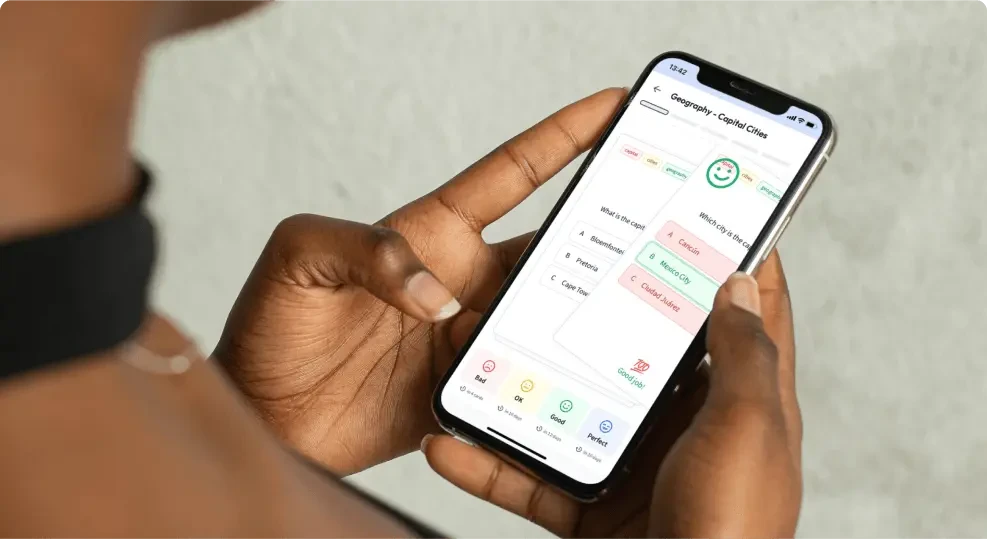
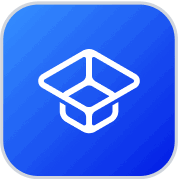
About StudySmarter
StudySmarter is a globally recognized educational technology company, offering a holistic learning platform designed for students of all ages and educational levels. Our platform provides learning support for a wide range of subjects, including STEM, Social Sciences, and Languages and also helps students to successfully master various tests and exams worldwide, such as GCSE, A Level, SAT, ACT, Abitur, and more. We offer an extensive library of learning materials, including interactive flashcards, comprehensive textbook solutions, and detailed explanations. The cutting-edge technology and tools we provide help students create their own learning materials. StudySmarter’s content is not only expert-verified but also regularly updated to ensure accuracy and relevance.
Learn more