Jump to a key chapter
Understanding Composite Material in Materials Engineering
Composite materials, often simply referred to as composites, are an essential aspect to explore in Materials Engineering.
Composites Definition in Engineering Context
In engineering, a composite material is defined as a material system composed of a combination of two or more micro- or macro- constituents that are distinct in form and chemical composition, and which remain separate in the finished structure. Put simply, this means a composite is made up of at least two materials which remain uniquely identifiable in the end product. The two individual materials work together to give the composite unique properties, but within the composite you can still see the individual materials in some way.
In this context, a primary constituent, often referred to as the 'matrix', binds together one or more secondary constituents, called 'reinforcements'. The property of the resultant composite is superior to those of the constituent materials.
Historical Origin of Composite Materials
Composite materials are not a new concept. Their use dates back to ancient times. The Egyptians and Mesopotamians used mud and straw to create strong and durable building bricks. They also used primitive composites for tools, weapons, and even early aircraft. The first modern composite, Fibreglass, was developed in the 1940s for use in the aerospace industry. The idea of combining materials to make them stronger has continued into modern times, which has resulted in the creation of a wide range of composites used today.
Categorical Examples of Composites in Engineering
A few categorical examples of engineering composites include:- Fibre-Reinforced Polymer (FRP)
- Structural Sandwich Composites
- Metal Matrix Composites (MMC)
- Ceramic Matrix Composites (CMC)
Fibre-Reinforced Polymer (FRP) | Composite materials made of a polymer matrix reinforced with fibres |
Structural Sandwich Composites | Composite materials fabricated by attaching two thin skins to a lightweight but thick core |
Metal Matrix Composites (MMC) | Composites with at least two constituent parts, one being a metal |
Ceramic Matrix Composites (CMC) | Composites in which both the matrix and the reinforcement are ceramics |
Commonly Used Composites in Industrial Applications
For example, consider Carbon Fibre Reinforced Polymers (CFRP) often used in the aerospace industry. The properties of CFRP composite matrix can be represented with tensor formulation in LaTeX:
\[ \begin{{bmatrix}} E_{1} & -v_{21}E_{1} \\ -v_{12}E_{2} & E_{2} \end{{bmatrix}} \]Where \(E_1\) and \(E_2\) are the Young's Moduli in the 1 and 2 directions, respectively, and \(v_{12}\) and \(v_{21}\) are the Poisson's ratios.
Use the following Python code to create a multidimensional array representing this matrix:
import numpy as np E1 = E2 = 210e9 # Young's Moduli in Pa v12 = v21 = 0.3 # Poisson's ratios matrix = np.array([[E1, -v21*E1], [-v12*E2, E2]]) print(matrix)
Another common type of composite material is Silicon carbide composite (SiC), which is used in high-temperature applications due to its excellent thermal and mechanical properties. It's constructed from silicon and carbon and used in situations where other materials would fail due to high temperatures.
Engineering Applications of Composite Material
Composite materials have found wide application across various branches of engineering. Their unique and superior properties, such as high strength, toughness, light weight, and resilience, have made them popular material choices in areas such as civil engineering, aerospace and automotive industries.
Role of Composites in Civil Engineering
Composite materials are revolutionising the landscape of civil engineering in multiple ways. They offer solutions to persisting age-old problems as well as emerging challenges in the field.
For instance, Fibre Reinforced Polymers (FRP), a type of composite material, are widely used in civil structures due to their excellent resistance to corrosion, high strength-to-weight ratio, and ease of installation.
- Buildings and bridges: FRPs are used for reinforcement in concrete structures, offering longer life and reduced maintenance costs.
- Tunnels: The high strength and corrosion resistance properties of FRPs make them ideal for tunnel linings.
- Water tanks: The impermeability and corrosion resistance of FRPs keep the water safe and clean.
- Pipes: FRP pipes have longer lifespan than traditional material pipes due to their resistance to corrosive chemicals and natural elements.
Use of Composite Materials in Construction
The use of composite materials in construction has seen a significant uptick as they offer flexibility, robustness, and cost-effectiveness.
Take Carbon Fibre Reinforced Polymer (CFRP) for instance. CFRP exhibits superior strength and stiffness properties. This makes it a popular choice in modern construction, particularly where high-performance materials are necessary. Here's how it's making its presence known:
- Construction beams: CFRP is used for reinforcement of concrete beams, enhancing their load-bearing capacity.
- Floor slabs: CFRP is used in the construction of floor slabs, providing great stiffness and strength.
- Roofing: CFRP is increasingly being used in roofing applications, providing strength and excellent weather resistance.
- Roadways: CFRP is used in the construction of roadways, for reinforcement and for dealing with shifting ground.
Aerospace and Automotive Utilisation of Composite Material
The aerospace and automotive industries have heavily adopted the utilisation of composite materials for their lightweight, high strength, and resistance to varying temperatures and corrosive environments.
The most commonly used composites in these industries are carbon, glass, and aramid fibre reinforced composites. High-temperature resistant composites like Ceramic Matrix Composites (CMCs) are particularly used in aircraft engines and braking systems in vehicles.
- In the aerospace industry, composite materials help in improving fuel efficiency by reducing the weight of the aircraft. They also require less maintenance, boosting operational efficiency.
- In the automotive industry, increasing the use of composite materials can lead to lighter vehicles, potentially reducing fuel use and greenhouse gas emissions.
Advancements in Transport Applications of Composites
Transportation applications of composite materials involve various forms of transport such as cars, trucks, ships, and trains. Among these, cars and airplanes have particularly benefitted from advancements in composite materials.
Aramid Fibre Reinforced Polymers (AFRP), for instance, are increasingly used in the construction of body parts for cars. Aramid fibres, such as Kevlar, possess high strength, stiffness, toughness, and resistance to chemical and thermal degradation. The low weight and high performance of AFRP have contributed to enhanced vehicle performance and fuel efficiency.
- Composite materials are used in the making of cabin interiors, seats, windows, and even propellers.
- With advancements like Thermoplastic Composites, more and more parts of an aircraft are being made with composites, contributing to weight reduction and improved fuel efficiency.
- Given their resistance to corrosion, the maintenance of aircrafts has significantly reduced.
Comprehensive Analysis of Composite Material Properties
Composite materials are often chosen for specific engineering applications due to their unique properties that offer favourable physical and chemical characteristics. To fully grasp their potential, it's important to explore these properties in depth.
Physical Properties of Composites
Composite materials display a vast array of physical properties that stand them apart from other material categories. These properties largely depend on the type of matrix and reinforcement materials used, their proportions, and the ways they are combined.
The main physical properties of composite materials include:- Density
- Strength
- Stiffness
- Thermal Expansion
- Thermal Conductivity
- Electrical Conductivity
Density | The mass of the material per unit volume, related to the densities of the matrix and reinforcement materials and their proportions |
Strength | The ability to withstand an applied load without breaking or deforming |
Stiffness | The measure of the rigidity of the object. The higher the stiffness value, the less flexible the material is |
Thermal Expansion | The rate at which a material expands for each degree rise in temperature |
Thermal Conductivity | The ability of a material to conduct heat |
Electrical Conductivity | The measure of a material's ability to conduct electricity |
Understanding the Strength and Durability of Composites
One of the key attributes of composite materials is their high strength and durability. Strength in composite materials is a complex characteristic largely due to its dependence on a variety of factors.
Strength in composite materials can be calculated using the 'Rule of Mixtures' and 'Inverse Rule of Mixtures'. The formula for calculating the longitudinal strength (\( \sigma_{Lc} \)) is given by: \[ \sigma_{Lc} = V_{f} . \sigma_{Lf} + V_{m} . \sigma_{Lm} \] where \(V_{f}\) and \(V_{m}\) represent the volume fraction of the fibre and matrix respectively, and \( \sigma_{Lf} \) and \( \sigma_{Lm} \) represent the longitudinal strength of the fibre and matrix respectively. Similarly, the transverse strength (\( \sigma_{Tc} \)) is given by: \[ \sigma_{Tc} = \frac{1}{\frac{V_{f}}{\sigma_{Tf}} + \frac{V_{m}}{\sigma_{Tm}}} \] The durability of composites is determined by their resistance to impacts and their ability to withstand changes in environment conditions (such as temperature and humidity) over extended periods. Factors such as the corrosion resistance of the matrix material, fibril orientation, and environmental stress-cracking resistance directly influence the durability of composites.Chemical Properties of Composite Materials
Composite materials also possess interesting chemical properties which contribute to their wide range of applications. Respectively, their chemical resistance to degradation, corrosion resistance, and dimensional stability are chief among such properties.
Reactivity and Resistance: Insight into Composites
Composite materials show varying degrees of resistance to chemical reactions. One common element that affects the reactivity of composites is the type of resin used in their production. Polyester resins offer resistance to a broad range of acids, alkalis, and solvents, whereas epoxy resins typically display superior resistance to most chemicals.
A material's susceptibility to chemical reaction can be quantified by its 'chemical potential', represented by the symbol \( \mu \). The chemical potential \( \mu \) for a component i in a solution is given by the formula: \[ \mu_{i} = \mu_{i}^{\circ} + RT \ln x_{i} \] where \( \mu_{i}^{\circ} \) in the standard-state chemical potential, R is the universal gas constant, x_{i} is the mole fraction and T is the temperature in Kelvin.The choice of fibre also has a profound effect on the composite's chemical resistance. For example, glass fibre composites resist a wide range of chemicals, but are vulnerable to attack by hydrofluoric acid, hot phosphoric acid, and strong alkaline solutions. Aramid fibre composites, on the other hand, can be sensitive to certain solvents and strong acids.
In summary, by selecting appropriate combinations of matrix and reinforcement materials, composites can be designed to withstand various harsh environments, making them an invaluable asset in multiple engineering applications.
Evaluating the Advantages of Composites in Engineering
Composite materials bring a multitude of advantages in engineering practices. Their notable attributes are cost-effectiveness, ability to provide enhanced performance and durability, energy efficiency, and environmentally friendly characteristics. Each of these factors contributes to the growing prevalence and application of composite materials in various engineering sectors.
Cost-Effective Nature of Composite Materials
Composite materials offer excellent long-term economic benefits. While the upfront costs of composite materials can be higher than traditional materials, the overall investment pays off significantly due to their longer lifespan and lower maintenance costs. Gone are the days when engineers had to regularly repair and replace corroded traditional materials - composites provide a more durable and cost-effective solution.
Cost-effectiveness of composites stems from two main sources: material efficient design possibilities and lifetime savings.
- Lower maintenance costs: Composites resist corrosion, reducing the need for costly repairs and frequent replacements.
- Increased life expectancy: Composites offer longer service life compared to traditional materials such as steel or concrete.
- Reduced installation costs: The lightweight nature of composites facilitates transportation and installation, thereby reducing associated costs.
Energy Efficiency and Its Direct Impact on Costs
Energy efficiency is another avenue where composite materials shine. The energy-efficient nature of composite materials directly correlates with cost savings, especially in industries like aerospace and automotive.
For instance, the fuel efficiency of vehicles and aircrafts depends heavily on their weight. By reducing the weight, the energy required to move these machines also decreases, leading to a significant decrease in fuel consumption and, therefore, operational costs. Composite materials, being lightweight yet strong, effectively address this issue. Replacing dense, heavy metals with light composites has greatly reduced energy consumption in these sectors, making them more environmentally friendly and cost-effective. Moreover, the superior thermal insulation properties of composites also contribute to their energy efficiency. This characteristic is especially beneficial in the construction industry where energy efficient buildings are increasingly sought after. With their excellent thermal insulation capabilities, composite materials help in maintaining the internal temperature of a building, reducing the need for energy-intensive heating and cooling systems, and thereby lowering energy costs.Enhanced Performance and Durability of Composites
In engineering applications, performance and durability carry crucial weight. Composite materials, with their unique combination of properties, provide an edge over traditional materials in these respects.
The enhanced performance of composites is mainly due to:- High strength-to-weight ratio: Composites have a strength-to-weight ratio greater than that of steel or aluminium. This makes them ideal for applications where strength without added weight is vital.
- Tailored properties: The properties of a composite can be customised to perform optimally under specific conditions. By adjusting the type, quantity, and orientation of the reinforcement, engineers can develop a composite that fits a specific purpose perfectly.
- Resistance to corrosion: Unlike metals, composites resist corrosion in all types of weather and harsh environments, making them ideal for outdoor and marine applications.
- Resistance to fatigue: Composites have greater fatigue strength than metals, which makes them highly effective in applications subject to cyclical loading.
The Role of Composites in Environmentally Friendly Engineering
As the focus on environmentally friendly practices grows, the role of composite materials in green engineering becomes noteworthy. They are not only suitable for creating energy-efficient systems, but they also contribute to reducing environmental impact in multiple ways.
Firstly, by reducing the energy consumed during the operational phase, composites contribute significantly to lowering greenhouse gas emissions. Their lightweight nature leads to decreased fuel consumption in vehicles and aircraft, whereas their thermal insulation properties result in energy savings in buildings. Secondly, some composites are made from renewable natural fibres such as flax, hemp, and jute, or recycled fibres from waste or scrap materials. Using these 'green' composites helps in reducing the reliance on non-renewable resources, and promotes material recycling and waste reduction. Moreover, some types of composites have a smaller carbon footprint than traditional materials. For instance, manufacturing glass fibre composites can emit less carbon dioxide than producing equivalent steel or aluminium parts. In summary, composite materials not only offer enhanced performance and cost-effectiveness but also contribute to reducing the impact on the environment, positioning them as an excellent choice for future engineering applications.Areas of Improvement and Challenges in the use of Composite Material
Despite the significant strides in the use of composite materials in engineering, there remains room for improvement, and pressing challenges persist. The journey towards optimising these materials is filled with compelling research, innovative approaches, and sustainable practices, overshadowed by some limitations. The areas for improvement focus primarily on manufacturing more lightweight and stronger composites, while the challenges centre on maintaining their cost-friendly and environmentally sustainable characteristics.
Scope for Improvement in Composite Materials Engineering
Engineering practices are continuously evolving. While composite materials offer numerous advantages over traditional materials in terms of their strength-to-weight ratio, maintenance costs, and durability, efforts are directed towards further improving their performance and cost-effectiveness. The core areas of exploration in this regard are aimed at creating lighter, stronger composites and refining the manufacturing processes to reduce production costs.
Lighter and stronger composites: This refers to the development of composite materials with a higher strength-to-weight ratio. The lighter a material, the less energy is needed to move it - an attribute highly desirable in industries like aerospace and auto engineering.
Refining manufacturing processes: This pertains to the various techniques and strategies used in the production of composites, aiming to make them more efficient, speedy, and cost-effective.
Ongoing Research on Lighter and Stronger Composites
Researchers and engineers are continually working on ways to make composites lighter without compromising their strength. Advancements such as nanocomposites - materials that are reinforced with nanoparticles - have shown promise in this regard. By dispersing nanoparticles into a composite matrix, the mechanical properties of the resulting nanocomposite, especially its strength and stiffness, can be significantly improved, providing an exceptional strength-to-weight ratio.
For example, carbon nanotubes, which are incredibly light yet strong, are mixed with other composite materials to engineer composites that could be used in making lighter and more fuel-efficient aircraft and vehicles.
Challenges in the Usage and Recycling of Composite Materials
While the advantages of composite materials are many, some challenges cannot be ignored. These primarily involve factors such as high initial production costs, limitations in recycling and environmental impact.
Production cost: The upfront cost of producing composites, particularly those reinforced with high-performance fibres, can be considerably high. This sometimes limits their usage to only high-value applications.
Recyclability and environmental impact: The recycling of composite materials, particularly thermoset composites, is quite challenging. Furthermore, the manufacturing processes for some composites can have a significant environmental impact, factors that contradict the drive towards sustainable engineering practices.
Overcoming the Limitations for a Sustainable Future with Composites
The high initial production costs are being addressed by focusing on the development of efficient and cost-effective manufacturing procedures. For instance, the use of automated fibre placement (AFP) and automated tape laying (ATL) technologies in the production of aerospace composites is being explored to lower production costs and increase efficiency.
The recyclability issue of composite materials warrants serious consideration for sustainable engineering practices. Various recycling techniques such as mechanical recycling, energy recovery, and feedstock recovery are being investigated for the recycling of composites, particularly thermoset composites.
In addition to this, research is being conducted into the development of bio-based composites and thermoplastic composites, which have a lower environmental impact and are easier to recycle. Addressing these challenges efficiently is crucial in ensuring that composite materials remain not only a viable but also a sustainable choice for future engineering applications. The combination of continual research, technological advancements, and a focus on sustainable practices should help overcome these hurdles and unlock the full potential of composite materials.Composite Material - Key takeaways
- Composite Material: These materials are commonly used in construction due its qualities of flexibility, robustness, and cost-effectiveness. Notably, Carbon Fibre Reinforced Polymer (CFRP) offers superior strength and stiffness, making it a popular choice in modern construction for tasks like enhancing load-bearing capacity of concrete beams, constructing floor slabs, roofing, and building roadways.
- Aerospace and Automotive Uses: Composite materials are used extensively in the aerospace and automotive industries for being lightweight, high in strength, and resistant to varying temperatures and corrosive environments. Notable examples are carbon, glass, aramid fibre reinforced composites, and high-temperature resistant composites like Ceramic Matrix Composites (CMCs).
- Physical and Chemical Properties of Composites: Composite materials exhibit various unique properties depending on the type of matrix and reinforcement materials used. Physical properties include density, strength, stiffness, thermal expansion, thermal conductivity, and electrical conductivity. Their chemical properties include resistance to degradation, corrosion resistance, and dimensional stability. The chemical potential of a component can determine its susceptibility to chemical reaction.
- Advantages of Composites in Engineering: Besides offering enhanced performance and durability, composite materials are cost-effective, energy efficient, and environmentally friendly. The mouldability of composites allows for material efficient designs, and their durable nature results to longer lifespan and lower maintenance costs. Their lightweight characteristic and superior thermal insulation properties contribute to energy efficiency in various applications.
- Environmentally Friendly Applications: Composite materials, especially green composites made from renewable or recycled fibres, significantly contribute to reducing environmental impact. Their energy-efficient nature leads to reduced greenhouse gas emissions, and their use in constructing energy efficient buildings result in lower energy costs.
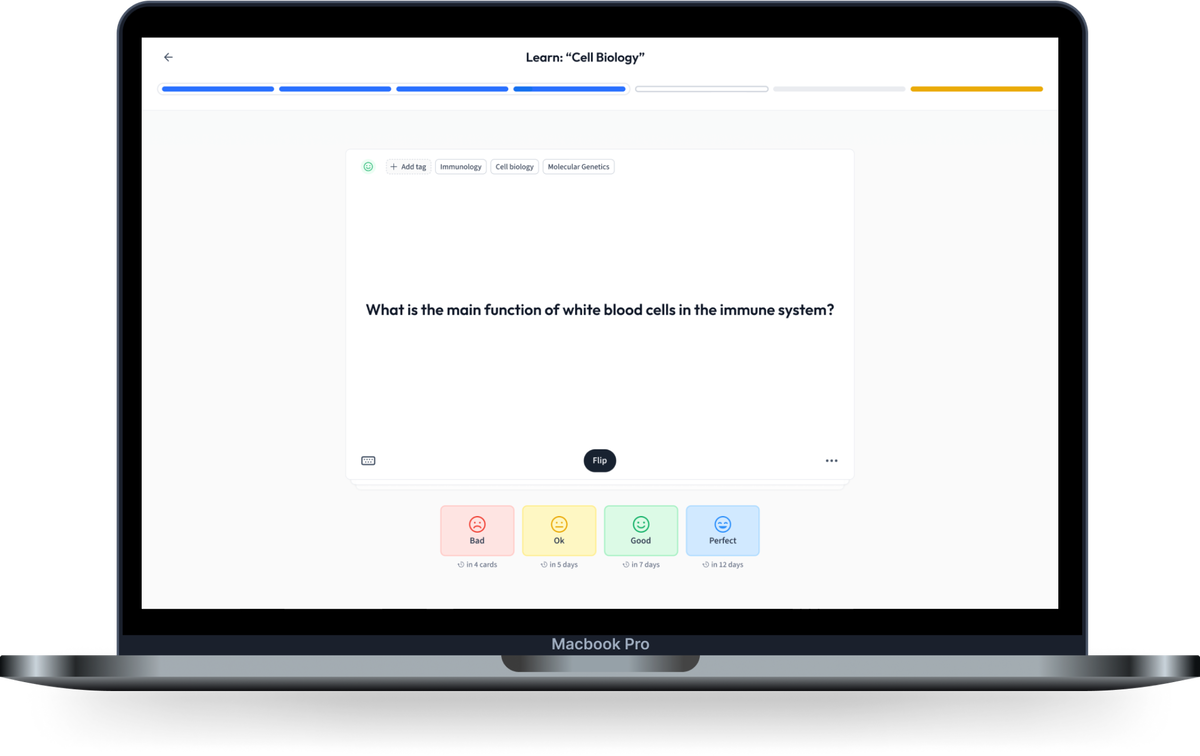
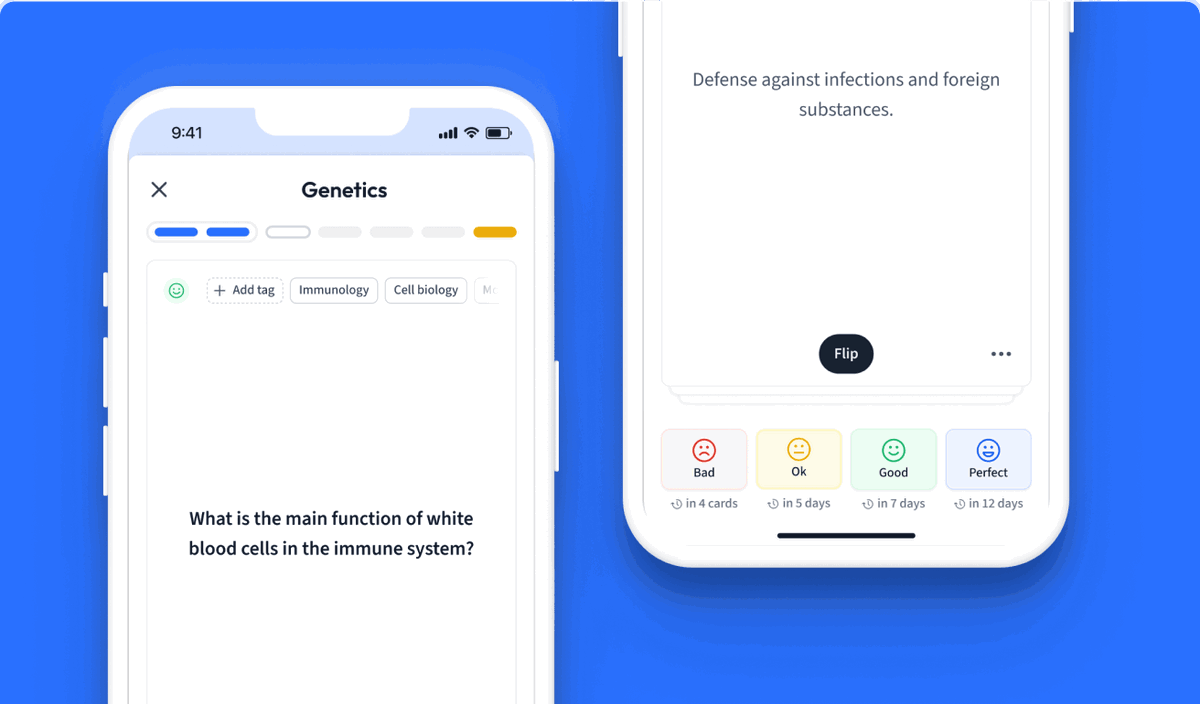
Learn with 15 Composite Material flashcards in the free StudySmarter app
We have 14,000 flashcards about Dynamic Landscapes.
Already have an account? Log in
Frequently Asked Questions about Composite Material
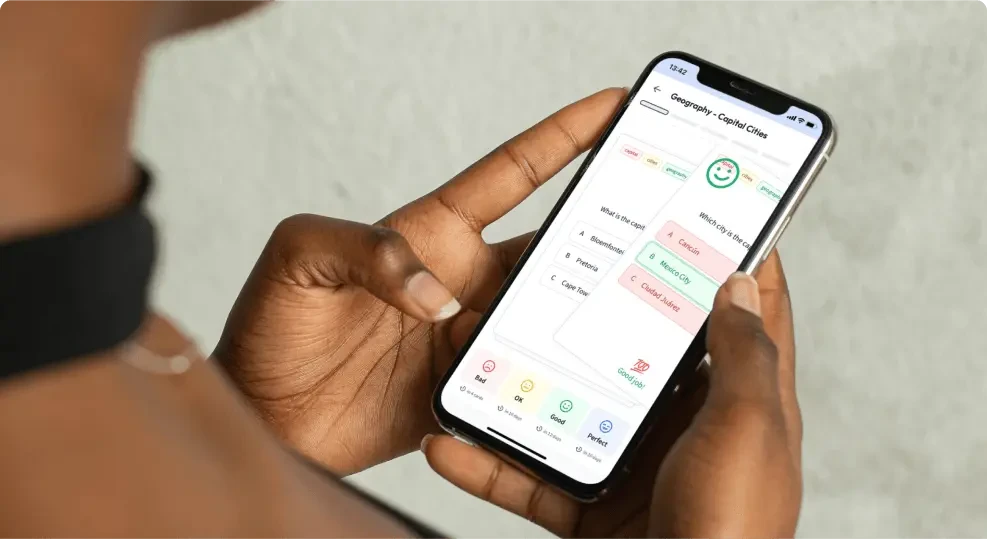
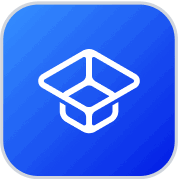
About StudySmarter
StudySmarter is a globally recognized educational technology company, offering a holistic learning platform designed for students of all ages and educational levels. Our platform provides learning support for a wide range of subjects, including STEM, Social Sciences, and Languages and also helps students to successfully master various tests and exams worldwide, such as GCSE, A Level, SAT, ACT, Abitur, and more. We offer an extensive library of learning materials, including interactive flashcards, comprehensive textbook solutions, and detailed explanations. The cutting-edge technology and tools we provide help students create their own learning materials. StudySmarter’s content is not only expert-verified but also regularly updated to ensure accuracy and relevance.
Learn more