Jump to a key chapter
An Overview of Diffusion Creep in Materials Engineering
Within the expansive field of materials engineering, you'll often come across a significant process known as 'Diffusion Creep'. This particular phenomenon plays a crucial role in determining how materials behave under various conditions. It is especially important when considering the performance of materials under high temperatures or stress conditions.
Understanding the basic concept of Diffusion Creep
Diffusion Creep can be defined as a deformation mechanism in materials where strain is produced because of the diffusion of atoms within the material.
To elaborate, when a material is subjected to high stress or temperatures, the atoms within the material move, or 'diffuse', from areas of high stress concentration to areas of lower stress. This results in deformation, or change in shape, of the material.
If you try to imagine atoms within a material as small balls packed tightly together, diffusion creep would be these balls slowly moving around and rearranging themselves when exposed to stress or heat.
The rate at which diffusion creep occurs within a material is directly related to the diffusion coefficient. The diffusion coefficient is a fundamental property that describes the rate at which atoms or molecules diffuse in a material.
Primary characteristics and kinds of Diffusion Creep
When considering Diffusion Creep, you'll notice two primary characteristics: it is time-dependent (the more time that passes, the more deformation occurs) and it is temperature-dependent (the higher the temperature, the faster the atoms move, and the more deformation occurs).
Moreover, there are three main types of Diffusion Creep:
- Nabarro–Herring creep
- Coble creep
- Solute drag creep
Each of these is slightly different, depending on the exact mechanism of atom diffusion.
The role of Diffusion Creep in Materials Engineering
In materials engineering, understanding and predicting material behaviour under extreme conditions is crucial. This is where the role of Diffusion Creep becomes important. The process of diffusion creep can affect the material's mechanical properties, like its strength, elasticity and toughness.
Name | Role of Diffusion Creep | ||||||
Strength | Long-term exposure to high stress can lead to deformation, reducing a material's strength. | ||||||
Elasticity | Diffusion Creep can alter a material's ability to return to its original shape after deformation. |
Creep Mechanism | Engineering Implications |
Diffusion Creep | Leads to uniform deformation. Considered during material selection and design for equipment operating at high temperatures, such as turbine blades or nuclear reactor components. |
Dislocation Creep | Causes non-uniform deformation. Factors in when selecting materials for applications experiencing higher stresses at intermediate temperatures, like microchips or structural parts in vehicles. |
Practical examples: Diffusion Creep vs Dislocation Creep
In engineering practice, the impact of Diffusion Creep and Dislocation Creep can be appreciated through notable examples.
Consider turbine blades in jet engines. Here, the high operating temperatures can cause Diffusion Creep, leading to gradual deformation over time. Conversely, in a computer microchip, Dislocation Creep may play a bigger role due to relatively lower temperatures and higher stresses, resulting in potential failure if not adequately mitigated.
Understanding the distinctive mechanics and influences of Diffusion Creep and Dislocation Creep is crucial in designing and operating anything from everyday devices to advanced machinery. By recognising the conditions that favour each creep mechanism, engineers can predict how materials will behave when exposed to stress and high temperatures, allowing them to optimise designs and materials for durability, safety, and performance.
The Effects of Grain Size on Diffusion Creep
Within the fascinating world of materials science, one crucial factor that can affect the rate of Diffusion Creep is grain size. The relationship between Diffusion Creep and grain size is such that the smaller the grain size, the higher the rate of creep. But let's delve deeper into this close-knit relationship.
How grain size influences Diffusion Creep
Understanding how different properties of a material influence Diffusion Creep is an enriching process. Grain size is one such influential factor that plays a crucial role. The 'grains' in materials are essentially small, randomly oriented, crystallised regions, and the lines that separate these regions are referred to as 'grain boundaries'.
The concept of 'grain boundaries' is important. These are spots where the atomic lattice discontinuities occur. This makes grain boundaries high energy regions where atoms can easily move from one grain to another.
In the case of Diffusion Creep, diffusion across grain boundaries (boundary diffusion) is generally faster than through the grain interiors (lattice diffusion). Hence, a material with smaller grains, meaning a larger number of grain boundaries, will exhibit a higher rate of Diffusion Creep.
The equation governing this relation can be expressed as follows:
\[ \dot{\varepsilon} = \frac{D}{d} \cdot \frac{\sigma}{kT} \]It can be clearly seen from the equation that the creep rate \( \dot{\varepsilon} \) is inversely proportional to the grain size \( d \). Thus, reducing the grain size \( d \) can accelerate the creep rate \( \dot{\varepsilon} \).
The relationship between grain size and Diffusion Creep
There exists a straightforward relationship between grain size and Diffusion Creep. As highlighted before, smaller grain size materials tend to have a higher Diffusion Creep rate. This is primarily because of the quicker diffusion along grain boundaries compared to within the grain. Grain boundaries offer a shortcut for atoms, enabling swift atomic transport and hence, quicker deformation of the material.
Moreover, a distinction lies in how the different modes of Diffusion Creep, namely Nabarro-Herring Creep and Coble Creep, are influenced by grain size. Nabarro-Herring Creep involves diffusion through the grains, while Coble Creep engages diffusion along grain boundaries.
- Nabarro-Herring Creep: In this case, diffusion takes place through the grain's volume and is hence less affected by grain size. However, significantly reducing the grain size can limit the relative contribution of Nabarro-Herring Creep.
- Coble Creep: Here, grain size plays a significant role as diffusion occurs along the grain boundaries. Reduction in grain size enhances the contribution of Coble Creep to the overall creep rate.
Case studies: Grain size effects on Diffusion Creep in action
Scientific studies and engineering practices provide some intriguing examples of grain size effects on Diffusion Creep. These case studies further elucidate the strong correlation between grain size and Diffusion Creep, enriching our understanding of this phenomenon and its implications on materials performance.
Research carried out on olivine, a mineral found in the Earth’s upper mantle, indicates the effect of grain size on creep rate. Tests were performed to understand Diffusion Creep of olivine under varying grain sizes. Results showed that the smaller the grains of olivine, the faster was the creep rate.
Another interesting observation of this relationship stems from the manufacturing of metal alloys. For example, in high-performance titanium alloys used in aeroplane engines, it is seen that a decrease in grain size leads to an increased rate of Creep, ultimately impacting the material’s performance and lifespan.
Controlling Diffusion Creep by manipulating grain size
Realising the effects that grain size has on Diffusion Creep, efforts can be made to control Diffusion Creep by manipulating the grain size in materials. Primarily, there are two techniques used to control grain size: grain growth control and grain size stabilisation.
- Grain Growth Control: This involves managing the conditions during the formation of the material to control the grain size. For instance, a slower cooling rate during solidification of a molten metal can lead to larger grains, whereas a faster cooling rate can result in smaller grains.
- Grain Size Stabilisation: Stabilising the size of grains in a material can prevent undesired changes in grain size over time due to processes such as annealing. It typically involves the addition of specific elements to the material that form fine particles, restraining grain growth.
Through such processes, it becomes possible to govern the Diffusion Creep rate and optimise a material’s performance based on its targeted application. This understanding lets engineers manipulate material properties to optimise performance, particularly in high-temperature applications. The grasp of this concept will be immensely useful for anyone who wishes to delve deeper into materials science or engineering.
Mechanisms and Stress Exponent in Diffusion Creep
Exploring the fascinating realm of Diffusion Creep, the interconnected mechanisms underlying this process and particularly, the significance of the stress exponent in shaping these mechanisms, holds critical insights. Let's unravel these high-level engineering concepts and decode their implications in materials engineering.
Decoding the Diffusion Creep mechanism
Understanding the underpinnings of Diffusion Creep involves appreciating the contributions of two fundamental processes: Volume diffusion and grain boundary diffusion. These two processes provide the core foundation of the two recognised mechanisms of Diffusion Creep: Nabarro-Herring Creep and Coble Creep.
The first mechanism, Nabarro-Herring Creep, is propelled by volume diffusion. This means that the atoms move — or diffuse— through the volume of the crystal lattice or 'grains' in a material. These movements generate vacancies which, under the influence of stress, aggregate towards the grain boundaries. This collective migration ultimately leads to the gradual deformation of the overall material, characterising the Diffusion Creep process.
Coble Creep, on the other hand, is facilitated by grain boundary diffusion. In this case, the atoms diffuse along the grain boundaries, circumventing the need to traverse the entire volume of the grains. This process offers a more efficient route for atom migration, hence, is normally more operative when the material is composed of smaller grains that present a larger grain boundary area for diffusion.
In both processes, the movements of atoms from a region of lower stress to a region of higher stress is the driving force, leading to the characteristic slow, plastic deformation of the material over time, otherwise known as 'creep'.
Coble Creep and Nabarro-Herring Creep are two mechanisms of Diffusion Creep where atoms migrate due to differential stress, leading to plastic deformation of the material.
The significance of the Diffusion Creep Stress Exponent
Amidst the dynamic interplay of forces in Diffusion Creep, the 'stress exponent' emerges as a pivotal factor, influencing the overall rate of creep. Termed as 'n', this stress exponent in the power-law creep equation, presides over how significant a role stress plays in influencing the creep rate.
\[ \dot{\varepsilon} = A \sigma^{n} \exp\left({-\frac{Q}{RT}}\right) \]In the equation above, \( \sigma \) is the applied stress, \( n \) is the stress exponent, while other elements \( A, Q, R, \) and \( T \) represent constant factors and temperature.
Curiously, for Diffusion Creep, the stress exponent 'n' equals 1. This means that the creep rate is linearly dependent on stress, hence, doubling the stress would simply double the creep rate, and so forth. This feature fundamentally differentiates Diffusion Creep from other types of creep mechanisms that inherently possess higher stress exponents and thus, more convoluted stress-creep rate dependencies.
Understanding the dynamics of the Diffusion Creep Stress Exponent
With such a commanding role, it's worthwhile to delve deeper into the stress exponent's interplay in Diffusion Creep. As mentioned earlier, in the arena of Diffusion Creep, the stress exponent equals one. Interestingly, this was a profound revelation, uncovered after numerous tests that manifest a linear relationship between stress and the creep rate, irrespective of most other factors.
Such a feature implies that, for materials dominated by Diffusion Creep, controlling the stress helps control the creep rate.
However, it's crucial to mention that while we often assume the stress exponent as a constant value (in this case, one), in reality, its value could somewhat fluctuate owing to various factors. Influential parameters can include temperature, time, grain size, and material properties, necessitating a complex understanding of the stress exponent and its variability under different conditions.
Case Studies: Diffusion Creep Stress Exponent applications in Materials Engineering
Turning theory into practice, various case studies underscore the critical role and practical implications of the Diffusion Creep Stress Exponent in materials engineering. Specifically, in high-temperature applications, where creep becomes a dominating deformation mechanism, understanding and leveraging knowledge of the stress exponent becomes intensely relevant.
For instance, in the manufacturing of turbine blades for jet engines, it is imperative to minimise creep at high temperatures to maintain blade integrity and prolong service life. The stress exponent's knowledge in Diffusion Creap informs engineers to judiciously manage stress levels to effectively control creep rates. Another case study could be in strategies for geological waste storage. Here, Diffusion Creep, influenced by the scenario-appropriate stress exponent, might determine the longevity and stability of the storage structures, especially under intense pressure conditions at great depths.
Thus, the role of the stress exponent, its dynamics, and profound impact on Diffusion Creep processes illuminate our understanding, tailor our approach, and optimise strategies to harness the best of materials science in practical applications.
Diffusion Creep - Key takeaways
- Diffusion Creep is a high-temperature deformation mechanism where atoms move from areas of high stress to low stress through the process of atomic diffusion, resulting in the change of shape of a material.
- The factors that affect the rate of Diffusion Creep include the diffusion coefficient, grain size, applied stress and absolute temperature. For instance, smaller grain size and higher stress or temperature can enhance the rate of Diffusion Creep.
- Diffusion Creep and Dislocation Creep are two key deformation mechanisms under stress. The main difference lies in the mode of atomic transport: in Diffusion Creep, deformation is due to the movement of atoms through the material, while in Dislocation Creep, deformation is due to the movement of dislocations.
- Grain size significantly influences Diffusion Creep. The 'grains' in materials are small, crystallised regions and the lines that separate these regions are 'grain boundaries'. Diffusion is generally faster across grain boundaries, and materials with smaller grain sizes, which have a larger number of grain boundaries, have a higher rate of Diffusion Creep.
- Stress exponent in Diffusion Creep determines the sensitivity of the creep rate to the applied stress. In general, Diffusion Creep exhibits a lower stress exponent compared to Dislocation Creep, meaning that Diffusion Creep is less sensitive to changes in applied stress.
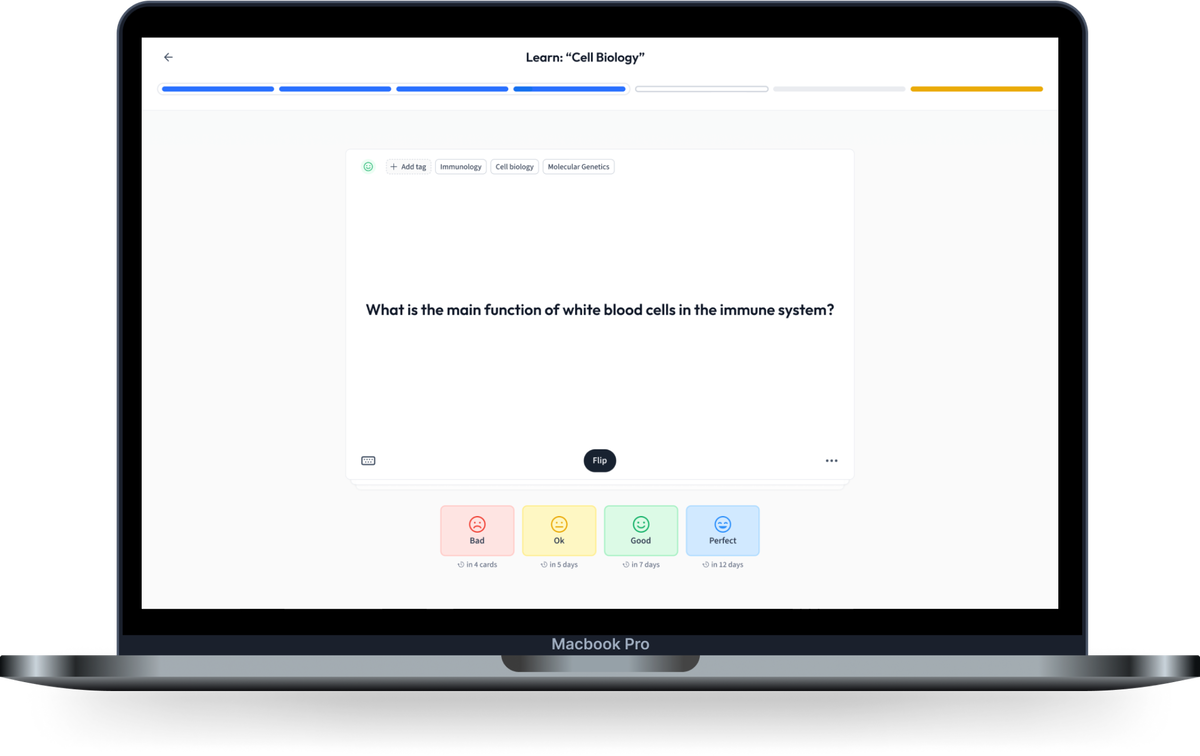
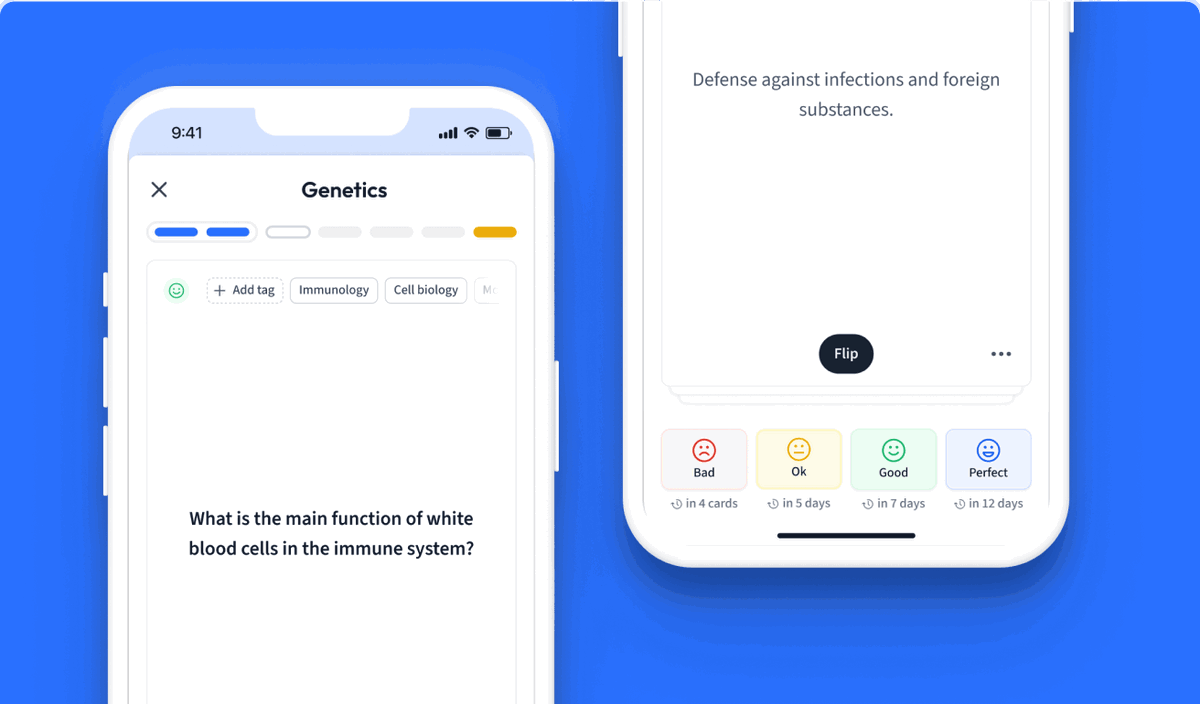
Learn with 15 Diffusion Creep flashcards in the free StudySmarter app
We have 14,000 flashcards about Dynamic Landscapes.
Already have an account? Log in
Frequently Asked Questions about Diffusion Creep
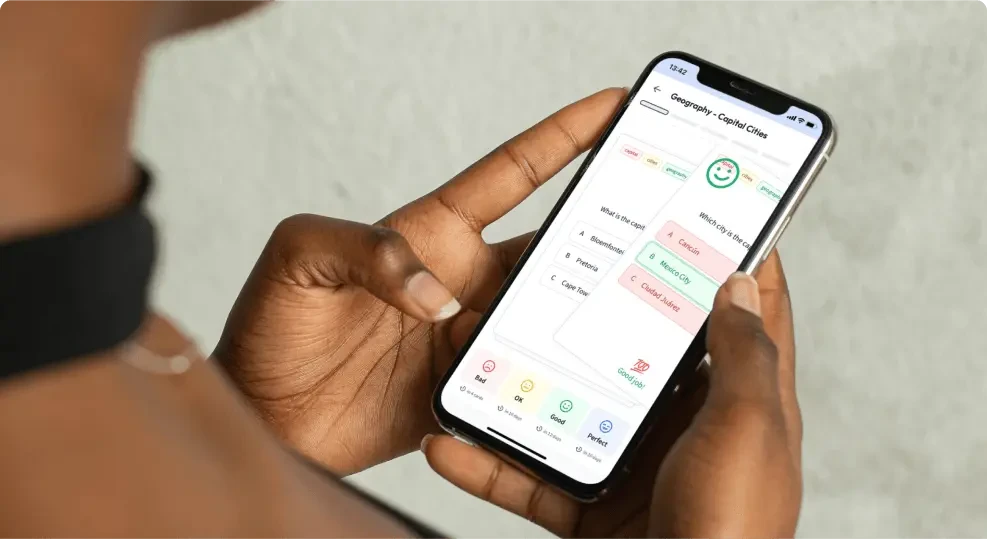
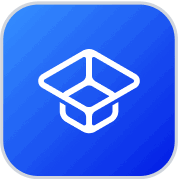
About StudySmarter
StudySmarter is a globally recognized educational technology company, offering a holistic learning platform designed for students of all ages and educational levels. Our platform provides learning support for a wide range of subjects, including STEM, Social Sciences, and Languages and also helps students to successfully master various tests and exams worldwide, such as GCSE, A Level, SAT, ACT, Abitur, and more. We offer an extensive library of learning materials, including interactive flashcards, comprehensive textbook solutions, and detailed explanations. The cutting-edge technology and tools we provide help students create their own learning materials. StudySmarter’s content is not only expert-verified but also regularly updated to ensure accuracy and relevance.
Learn more