Jump to a key chapter
Understanding the Concept of Phase Transformations
Phase Transformations constitute a very important part in the field of materials engineering. This phenomenon involves a change in the physical phase of a substance due to a change in temperature, pressure, or the addition of a substance. Understanding these transformations will offer fundamental knowledge about the behaviour of materials under a variety of conditions and directly influence their performance in engineering applications.
Phase Transformations Definition
Phase transformations refer to the transition of matter between solid, liquid, and gaseous states. They can also occur between different crystal structures or molecular structures within those states. The transformations between solid, liquid and gaseous states are called first-order phase transitions while transformations within the same state are known as second-order phase transformations.
The classic example of this phenomenon can be represented by the heating and cooling of pure iron:
Temperature (°C) | Phase |
below 912 | α-Ferrite (bcc) |
912 to 1394 | γ-Ferrite (fcc) |
above 1394 | δ-Ferrite (bcc) |
Mathematically, these transformations can be determined using the Gibbs Phase Rule, which states that the number of independent variables \(F\) in a system at equilibrium is given by, \[ F = C - P + 2 \] where \(C\) is the number of components and \(P\) is the number of phases.
The phase transformations are not instant but rather proceed over a range of temperatures or pressures. This range is often referred to as a phase field, within which multiple phases may co-exist.
Real Life Examples of Phase Transformations
Phase transformations are ubiquitous in our daily life. A few common examples include:
- Water changing from a liquid to a gas when boiled or from a liquid to a solid when frozen.
- The conversion of graphite to diamond under high pressure and temperature conditions.
- When jewellery is made- Gold alloy, with a mixture of gold, copper, and silver initially in a solid phase, is transformed into a liquid phase to be reshaped or resized.
- Concrete setting- After being mixed and cast, concrete undergoes a chemical phase transformation to become a solid material.
- Butter melting on a hot frying pan.
- Rusting of Iron.
By understanding the impact of these transformations on the physical properties of materials, you can better predict and control their behaviour in a range of applications.
An In-depth Look at Phase Transformations in Metals
Metals, intrinsically composed of microstructures, undergo phase transformations due to several influencing factors such as temperature, pressure and time. These transformations have profound implications for the properties, functionality and utilisation of metals. Understanding these transformations, therefore, is critical to the refinement and optimisation of metallic materials in the fields of engineering and metallurgy.
The Role of Temperature in Phase Transformations in Metals
Temperature plays an integral role in inducing phase transformations in metals. These transformations are typically classified as equilibrium or non-equilibrium based on how systematically they occur in response to variations in temperature. In an equilibrium phase transformation, a metal is allowed to adjust its microstructure at each temperature during the heating or cooling process. This allows the atoms enough time to diffuse and rearrange into the most thermodynamically stable formation.
However, real-world processing conditions usually don't afford such luxuries of time. Most industrial treatments of metals involve rapid temperature changes that result in non-equilibrium phase transformations. Here, the transformations are dictated by kinetic factors, giving rise to a plethora of metastable microstructures.
A classic example to illustrate this is the transformation of Austenite to Ferrite and Cementite in the cooling of carbon steel. If the cooling is abrupt (quenching), a metastable phase called Martensite is formed, which is extremely hard and brittle. Contrarily, slow cooling results in the formation of the equilibrium microstructure: Pearlite, a composite of Ferrite and Cementite.
The transformation temperature is the temperature at which the original phase starts to transform into the new phase. For each type of phase transformation, each metal has a unique phase transformation diagram or phase diagram that depicts what phases are present at a given temperature. Mathematically, various models such as the Johnson-Mehl-Avrami-Kolmogorov (JMAK) model are employed to monitor these transformations. The JMAK equation is, \[ X(t) = 1 - \exp \left( -k t^n \right) \] where \(X(t)\) represents the fraction of material that has transformed at time \(t\), \(k\) is a temperature-dependent rate constant, and \(n\) is the Avrami exponent, which varies based on the transformation mechanism.
How Phase Transformations Influence the Properties of Metals
Phase transformations in metals lead to dramatic alterations in their properties. As a metal undergoes a phase transformation, the characteristics of its new phase – such as crystalline structure, mechanical strength, density, and electrical properties – could vary significantly from those of its initial phase.
The term 'properties' refers to various physical characteristics a metal exhibits. This includes but is not limited to, mechanical properties (like ductility, hardness, strength), electrical properties (conductivity, resistivity), and magnetic properties (permeability, coercivity).
The substantial influence of phase transformations on the properties of metals is leveraged in a variety of industrial applications. For instance, in steel production, adjustments to the cooling rate can induce different phase transformations, yielding distinct microstructures such as Martensite, Bainite, or Pearlite, each with unique properties. Martensite's hardness makes it suitable for tool steels, while the ductility and toughness of Pearlite find applications in structural steels.
Furthermore, phase transformations are also crucial to processes such as age hardening of aluminium alloys, where heat treatments facilitate a sequence of transformations leading to the precipitation of second-phase particles – strengthening the alloys.
In dental amalgams, for example, the mercury (liquid phase) is mixed with silver, tin, and copper alloy (solid phase) under high pressure until the alloy dissolves into the mercury to form a malleable paste. Once setting begins, a series of phase transformations occur that results in a hard, durable filling material.
Armed with this knowledge, you can comprehend how controlling phase transformations provides opportunities to tailor metals to cater to specific functional requirements, paving the way for advancements in technology, architecture, healthcare, and many more sectors.
Characteristics of Phase Transformations
Phase transformations, vital in both natural processes and industrial applications, are characterised by a number of specific features that make them unique forms of physical change. They occur under certain set conditions, and involve a switch to an energetically more favourable state.
Key Characteristics of Phase Transformations
Pertinent characteristics defining phase transformations include:
- Transformative States: The process involves the transformation from one phase to another — solid to liquid (melting), liquid to gas (evaporation), solid to gas (sublimation) and their reverse processes are all examples of phase transformations.
- Energy Changes: Every phase transformation is typically accompanied by an exchange of thermal energy — energy is absorbed during melting, evaporation and sublimation, while it is released during freezing, condensation and deposition.
- Pressure and Temperature Conditions: Phase transformations are fundamentally reliant on specific conditions of temperature and pressure — changes in these variables can determine the direction and extent of a phase transformation. For example, an increase in pressure causes gases to convert into liquids and further into solids, while an increase in temperature causes solids to become liquids and further vaporise into gases.
- Thermodynamic System: The transformations are defined in terms of a thermodynamic system transitioning from one state to another — usually from a less stable state to a more stable and lower energy state.
- Irreversibility: Once the phase transformation is complete, the process often can’t be reversed, or if it can, it is under specific conditions — especially in the case of second-order transformations.
Let's delve a little deeper into these characteristics:
Transformative States: A phase represents a uniform portion of a material, having the same physical and chemical properties. Two identical phases separated by a phase boundary makes a two-phase system. Phase transformations see materials transition between these states, serving as the differentiating factor from other physical phenomena.
Energy Changes: Phase transformations imply a shift in the structure of matter, hence cause energy changes. Events such as melting or evaporating demand energy (endothermic) to overcome intermolecular forces, while transitions like freezing or condensation release energy (exothermic).
Pressure and Temperature Conditions: A factor crucial to initiating a phase change is either temperature or pressure. For example, water subjected to a temperature below 0°C (at normal pressure) will freeze into ice, exhibiting a solid-liquid phase transformation. Similarly, pressure-induced phase transformations are a typical occurrence in the Earth’s mantle due to the extreme pressures that exist.
Thermodynamic System: Phase transformations come under a crucial thermodynamics theme, focusing on the system's energy changes. The phases always tend towards the energetically more favourable state (lower Gibbs free energy). The Gibbs free energy, G, is given by: \[ G = H - TS \] where H is enthalpy, T is absolute temperature, and S is entropy. For a phase transformation to occur, \(\Delta G\) should be negative.
Irreversibility: The paths taken by the elements and compounds during phase transformation are usually irreversible, in the sense, once formed, the new phase does not degrade or is degradable only under specific conditions. This is especially true for kinetically driven, non-equilibrium transformations where the new phase is 'trapped' into a metastable state.
Remember, while these properties do make phase transformations a fascinating study, complexities arise in real world scenarios. Asynchronous transformations and transformations under non-ideal conditions often yield unexpected results, making the field of phase transformations ever-evolving and intriguing.
Phase Transformation Diagrams Simplified
Phase transformation diagrams are invaluable tools, providing a graphical map of when and how phase transformations occur under equilibrium conditions. They outline the stability of different phases relative to temperature and composition of the system and assist in understanding the impact of controlled heating and cooling on phase transformation and material properties.
Reading and Interpreting Phase Transformation Diagrams
Phase diagrams are effectively a roadmap to illustrate how a particular material will behave under different conditions of temperature and pressure. Two of the most commonly referenced diagrams are the unary, or single-component phase diagrams, and the binary, or two-component phase diagrams.
The unary phase diagram represents the phase behaviour of a pure substance, while the binary phase diagram represents the phase behaviour of a mixture of two different substances. In a unary diagram, the vertical dimension typically represents pressure, while the horizontal dimension represents temperature.
In contrast, for a binary phase diagram, the vertical axis still represents temperature, but the horizontal axis now corresponds to composition, expressed as the mass percentage or mole percentage of one component. The diagram is divided into regions, each region representing a single phase or multiple phases, stipulating the material's state under particular conditions of temperature and composition.
An important feature in binary phase diagrams is the solubility limit. This line defines the maximum concentration of the solute which the solvent can hold at that particular temperature. To the left of this line, the material is completely solid, while to the right, it’s a mixture of liquid and solid
'Solubility limit' refers to the maximum amount of a solute that can be dissolved in a solvent at a given temperature.
Another critical line you'll come across is the liquidus line. Above this line, materials are in a liquid state. There's also the solidus line; below this line, the material is fully solid. The region between the liquidus and solidus lines is where you'd find the mixture of liquid and solid.
Consider the phase diagram for water. Every time you freeze water into ice or boil it into steam, you are moving along the lines in its unary phase diagram. When you heat ice, it changes to water at 0°C under 1 atmosphere of pressure and turns into steam at 100°C under the same pressure. These transition temperatures get lower at higher altitudes, where the pressure is less - information that you can quickly reference from the phase diagram.
Unary Phase Diagram | A phase diagram that involves only one component. It usually demonstrates the effects of temperature and pressure on a substance in a closed system. |
Binary Phase Diagram | A phase diagram that involves two components. It usually illustrates the limits of phase stability in terms of temperature and composition in a closed system. |
Solubility Limit | The maximum concentration of solute that can dissolve in a solvent at a given temperature. |
Liquidus Line | Represents the temperatures above which the material is completely in the liquid phase. |
Solidus Line | Represents the temperatures below which the material is completely in the solid phase. |
Learning how to read phase diagrams can be immensely helpful when dealing with materials. It allows you to predict the phase changes that will take place in a material at various temperatures, and consequently, modify the material's properties by altering their phase compositions. This can lead to the development of new materials that are custom-tailored for particular applications.
Various Types of Phase Transformations
Phase transformations are classified into various types based on a range of factors such as the nature of phase change, the driving force, and the mechanism of transformation. Gaining an understanding of these different types provides critical insights into the behaviour of materials under various conditions.
Common Phase Transformation Types
There are several types of phase transformations that are encountered in the study of materials science. Some of the most commonly observed are:
- Eutectoid Transformations
- Eutectic Transformations
- Peritectic Transformations
- Congruent Transformations
- Incongruent Transformations
- Eutectoid Transformation: A Eutectoid Transformation takes place when a solid transforms into two different solids. This transformation occurs at a fixed temperature and composition. A classic example is the transformation from austenite to pearlite in iron-carbon alloys.
- Eutectic Transformation: This transformation mirrors Eutectoid Transformation, but it involves the transition from a liquid to two different solid phases.
- Peritectic Transformation: A Peritectic Transformation involves the reaction between a solid and a liquid to yield a different solid phase. Much like the eutectoid and eutectic transformations, it happens under predetermined temperature and composition conditions.
- Congruent Transformation: In a Congruent Transformation, the phase transformation happens in such a way that there's no change in the composition of the system. For example, when graphite turns into diamond under high-pressure conditions, the composition (pure carbon) remains the same, and hence this transformation is congruent.
- Incongruent Transformation: Contrastingly, an Incongruent Transformation involves a change in the composition of the system. For instance, when limestone (calcium carbonate) is heated, it decomposes to form calcium oxide and carbon dioxide. This change in composition characterises the transformation as incongruent.
Understanding the Differences Between Phase Transformation Types
It is important to understand the differences between these phase transformations as each type results in unique final microstructures and hence, different macroscopic properties:
- Eutectoid vs Eutectic Transformations: Although these two transformations are similar in that they both involve one phase transforming into two, there's a distinct difference. In a Eutectoid Transformation, a single solid phase transforms into two other solid phases. In contrast, a Eutectic Transformation involves a liquid phase transforming into two different solid phases. Because of these differences, they result in two different microstructures.
- Eutectic vs Peritectic Transformations: These two transformation types differ based on their initial phases. While a Eutectic Transformation begins with a single liquid phase, a Peritectic Transformation involves a solid and a liquid reacting to form a new solid phase.
- Congruent vs Incongruent Transformations: The key difference between these two transformations is compositional change. In Congruent Transformations, the system's composition remains unchanged; for instance, when diamond (pure carbon) transforms to graphite (also pure carbon). With Incongruent Transformations, the system's composition changes. For example, when limestone (calcium carbonate) turns into calcium oxide and carbon dioxide upon heating.
In essence, the nature of the initial and final phases, their quantities, and the compositional changes define these various types of transformations. They are significant for developing and optimizing processes in material manufacturing by manipulating these transformation pathways to foster desirable material properties.
Phase Transformations - Key takeaways
- Phase transformations in metals are influenced by factors such as temperature, pressure, and time.
- Temperature-induced transformations in metals could be of two types: equilibrium (systematically occurring) and non-equilibrium (kinetically driven) transformations.
- The transformation temperature is the temperature at which the original phase starts to transform into a new phase, and is monitored using models such as the Johnson-Mehl-Avrami-Kolmogorov (JMAK) model.
- Phase transformations can drastically alter the properties of a metal, including its crystalline structure, mechanical strength, density, and electrical properties.
- Phase transformations in metals can be graphically illustrated using phase transformation diagrams, which show the stability of different phases relative to the temperature and composition of the system.
Learn faster with the 15 flashcards about Phase Transformations
Sign up for free to gain access to all our flashcards.
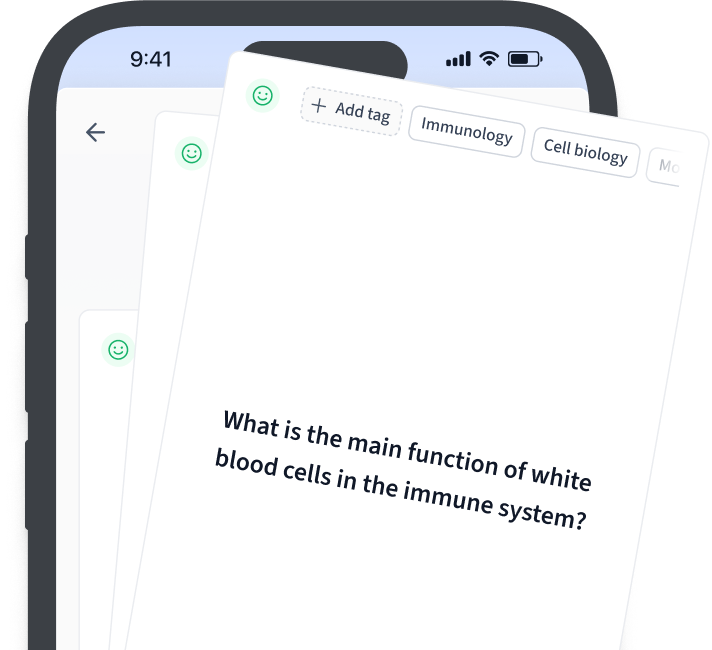
Frequently Asked Questions about Phase Transformations
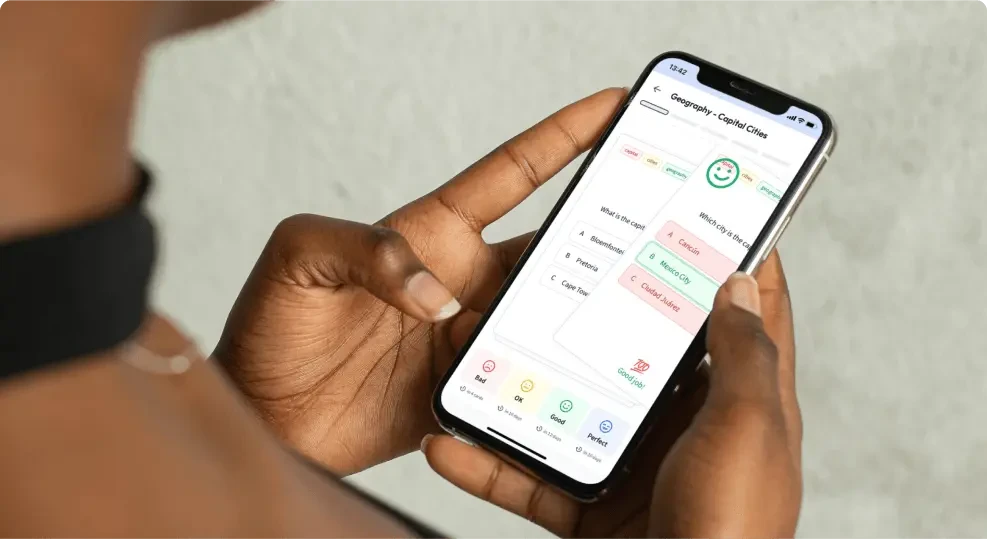
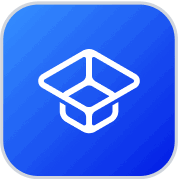
About StudySmarter
StudySmarter is a globally recognized educational technology company, offering a holistic learning platform designed for students of all ages and educational levels. Our platform provides learning support for a wide range of subjects, including STEM, Social Sciences, and Languages and also helps students to successfully master various tests and exams worldwide, such as GCSE, A Level, SAT, ACT, Abitur, and more. We offer an extensive library of learning materials, including interactive flashcards, comprehensive textbook solutions, and detailed explanations. The cutting-edge technology and tools we provide help students create their own learning materials. StudySmarter’s content is not only expert-verified but also regularly updated to ensure accuracy and relevance.
Learn more