Jump to a key chapter
Understanding Stress Corrosion Cracking
Stress Corrosion Cracking, often abbreviated as SCC, is a critical concern in the field of engineering. It represents a type of damage that can occur in various materials under stressful conditions in corrosive environments. Let's delve deeper into its definition, causes, and influencing factors in the following sections.
Definition of Stress Corrosion Cracking
Stress Corrosion Cracking (SCC) is defined as the process where certain materials, particularly metals, deteriorate by cracking due to the combined effects of tensile stress and a corrosive environment. This failure can lead to unexpected and sudden breakdowns in structures that show no external signs of distress.
The Role of Stress Corrosion Cracking in Materials Engineering
In the field of materials engineering, understanding SCC is vital. It plays a significant role in determining the lifespan and reliability of different manufactured structures. When SCC happens, it can compromise the integrity of the infrastructure, leading to failures that may have serious implications, especially when it comes to safety.
An example would be a cracking in oil pipelines. The combination of stress from the transported oil and corrosive substances within the oil can cause SCC, potentially resulting in significant environmental and economic damage.
Causes of Stress Corrosion Cracking
- Chemical Environment: The presence of certain corrosive substances can cause SCC. These can include chloride ions, sulphide ions, and hydrogen sulphide, among others.
- Material Susceptibility: Certain materials are more prone to SCC. These generally include high-strength alloys.
- Stress: Both residual and applied stress can lead to SCC. This could be tension, bending, or torsional stresses.
Factors Influencing Stress Corrosion Cracking
The tendency of a material to undergo SCC is influenced by several factors:
These factors can be broadly divided into three main categories: environmental factors, material factors, and mechanical factors.
Environmental factors | Includes temperature, humidity, and the presence of certain chemicals. |
Material factors | Relates to the inherent properties of the material, such as its chemical composition and microstructure. |
Mechanical factors | Includes the type of stress, its magnitude and the rate of strain. |
The Interplay of Factors
It is important to note that the interplay of these various factors determines the susceptibility of a material to Stress Corrosion Cracking. A material might be resistant to SCC in one environmental condition but may become prone to it when circumstances change.
For instance, increasing the operating temperature can sometimes decrease the risk of SCC but may also increase it in other situations.
A Closer Look at the Stress Corrosion Cracking Mechanism
Let's delve a little deeper to comprehend the mechanism behind Stress Corrosion Cracking. By looking at the procedure behind SCC, the conditions that lead to this phenomenon, and its potential consequences, one can better understand its complete process and develop strategies to prevent or manage it effectively.
The Process of Stress Corrosion Cracking
Stress Corrosion Cracking is a complex process, and it can be viewed as a harmonised occurrence of corrosion-reaction, element-transportation, and mechanical-deformation. It generally commences with the formation of miniature corrosion pits or cracks on the material's surface. Over time, these minuscule cracks propagate and extend under the effect of stress.
The overall process is often divided into the following stages:
- Initiation: In the presence of tensile stress and a corrosive environment, small, often microscopic, cracks start to form on the surface of the material.
- Propagation: These cracks, under continued stress and corrosion, begin to propagate through the material. Crack propagation can be modelled using the formula \[ \frac{{da}}{{dt}} = AK^{{n}}\], where \(a\) is the crack length, \(t\) is the duration, \(A\) and \(n\) are constants, and \(K\) is the stress intensity factor.
- Failure: Finally, when the cracks reach a critical size, the structure fails under the applied or residual stress.
The whole process is quite dynamic and can be affected by a number of factors including the material properties, environment, and mechanical load conditions.
Conditions Leading to Stress Corrosion Cracking Mechanism
Not all materials and environments lead to Stress Corrosion Cracking. Specific conditions must be met for SCC to occur. These conditions are;
- Tensile Stress: This can be an externally applied load or residual stress within the material.
- Susceptible Material: The material must be vulnerable to SCC in the given environment. High strength alloys are particularly prone to cracking.
- Corrosive Environment: Certain chemical species or conditions such as high temperature or pressure or the presence of specific corrosive elements can engender SCC. For example, stainless steels are susceptible to SCC in the presence of chlorides.
It's important to note that the absence of any one of these conditions can drastically decrease the chances of SCC occurring.
Consequences of Stress Corrosion Cracking
Stress Corrosion Cracking, when unchecked, can cause disastrous consequences. It poses a significant risk because it is hard to detect in the early stages, and it minimises the tensile strength and load-bearing capacity of materials.
Structural Compromise | SCC can lead to the failure of structures, compromising the integrity of buildings, bridges, and other critical infrastructure. |
Economic Impact | The cost of repairing or replacing corroded structures can be significant. Preventative measures are necessary to reduce these expenses. |
Safety Risk | Unexpected structural failures due to SCC could lead to accidents and potential loss of human lives. |
A comprehensive understanding of Stress Corrosion Cracking and its consequences can aid engineers and researchers in designing safer and more reliable structures, reduce maintenance costs, and prevent catastrophic structural failures.
Stress Corrosion Cracking Examples in Engineering
Real-world examples can enhance the understanding of Stress Corrosion Cracking. From inherent flaws in industrial applications to case studies of accidents caused by SCC, these real-life instances highlight the importance of understanding and mitigating the effects of SCC.
Examples of Stress Corrosion Cracking in Industry
In industrial environments, there have been numerous recorded cases of Stress Corrosion Cracking. SCC isn't confined to a single type of infrastructure or specific type of material, which makes it a pervasive issue.
A significant content of SCC has been reported in the pipelines of the oil and gas industry. In these cases, the combination of the high-strength alloys used in pipe manufacturing, the extreme operating pressures, and the corrosive chloride ions present in the environment, lead to the ideal conditions for SCC to occur. The subsequent cracking can lead to severe pipeline failures, resulting in expensive repairs, significant downtime, and, in worst-case scenarios, harmful environmental impacts.
Another noteworthy instance of SCC is in the nuclear power industry. Many nuclear power plants use stainless steel in their reactor pressure vessels, due to its high resistance to heat and corrosion. However, under the high temperatures and pressures these vessels operate, and in the presence of an environment enriched with high concentrations of hydroxyl anions, these stainless-steel vessels can undergo Intergranular Stress Corrosion Cracking (IGSCC). This is a specific type of SCC where the crack propagation follows the grain boundaries of the material. Any form of cracking in nuclear power plants can have dangerous implications and hence, SCC is a significant concern in this industry.
Examining Real-World Stress Corrosion Cracking Scenarios
A deep dive into specific instances of Stress Corrosion Cracking can provide insights into the dangers associated with it and highlight the importance of proactive prevention and detection methods.
A striking case of SCC terrorised the public in 2003 when the Big Dig tunnel in Boston, United States, suffered a deadly ceiling collapse. The construction used epoxy anchors to secure heavy concrete ceiling panels. The combination of the installed stress in the anchors, the corrosive environment within the tunnel, and the susceptibility of the anchor material led to SCC. The corrosion cracks propagated unseen until they reached a critical size, causing a catastrophic ceiling panel collapse that resulted in a fatality. This real-world example underscores the insidious nature of SCC – it can occur without any external signs and lead to disastrous failures.
Another real-world scenario unfolded in the 1990s when Stress Corrosion Cracking severely impacted the fleet of F-14 Tomcat aircraft. This fighter jet used high-strength steel in its landing gear, which was therefore prone to SCC. This led to a series of incidents where the landing gear of these aircraft failed when under stress during landing, risking the lives of the pilots and damaging expensive aircraft.
These real-life scenarios underscore the importance of effective detection and prevention methods for Stress Corrosion Cracking. Understanding that the interplay of materials, environmental factors, and stress conditions can lead to SCC, allows for appropriate material selection, suitable design decisions, and preventive maintenance schedules to minimise the risk of catastrophic failures due to SCC.
Testing for Stress Corrosion Cracking
Diagnosing the presence of Stress Corrosion Cracking (SCC) and gauging its extent is an essential aspect of managing this phenomenon, and this entails conducting appropriate tests. Let's delve into the major methods that are employed and discover how to interpret the results from these tests.
Methods of Stress Corrosion Cracking Test
Various approaches can be adopted to detect SCC, each with its unique strengths and applicable contexts. These tests typically come under one of two categories:
- Non-destructive Testing (NDT): These methods inspect the material without causing any harm or altering its properties.
- Laboratory testing: These tests are conducted in a controlled environment and can provide more detailed and quantitative data.
Determining which testing method to use depends largely on the specifics of the situation, including the type and size of the component, the severity of the potential failure, and the practicality and cost of the testing process.
Non-destructive Testing (NDT) methods for SCC include:
- Visual inspection: Although simple, visual inspection can often reveal signs of SCC, such as surface cracks or localised corrosion.
- Ultrasonic Testing (UT): This method uses high-frequency sound waves to detect internal and surface defects. UT can provide information about the depth, location, and size of the cracks.
- Eddy Current Testing (ECT): Effective for surface and near-surface defects, ECT exploits the concept of electromagnetic induction to detect disruptions that may be caused by SCC.
In the realm of Laboratory Testing, several techniques can be employed:
- Sustained Load Testing: In this method, the test sample is subjected to a constant tensile load in a corrosive environment over a long period. The time to failure is then used to determine the sample's SCC resistance.
- Slow Strain Rate Testing (SSRT): SSRT involves exposing the test sample to a corrosive environment while applying a slow, constant strain rate. Decreases in ductility compared to a sample tested in a benign environment indicate SCC.
Interpreting Stress Corrosion Cracking Test Results
Having conducted tests to detect SCC, the next step is interpreting the data. How does one interpret Stress Corrosion Cracking Test Results? There are broad guidelines to follow.
For Non-destructive tests, the results often come in the form of graphical images or scans. For instance, in Ultrasonic Testing, a 'B-scan' provides a cross-sectional view, highlighting potential defects. Qualified personnel then interpret these images, identifying anomalies that signify possible SCC. It's important to note that these methods often require prior knowledge of the structure to distinguish between relevant signals and noise.
In the case of Laboratory Tests, the interpretation of results can be more quantitative. For instance, in a Sustained Load Test, the time to failure under a specific load can be compared to the material's known performance in non-corrosive environments. A significant decrease in time suggests a susceptibility to SCC. In Slow Strain Rate Tests, a reduction in ductility or an increase in the plastic elongation rate may denote SCC vulnerability.
It is crucial to remember that although testing and interpreting the results can suggest the presence and severity of SCC, it is only through a detailed investigation that a definitive judgement can be made. Often, destructive tests like fractographic analysis are necessary for a thorough evaluation.
Test Type | SCC Indication |
Visual Inspection | Surface cracks or localised corrosion |
Ultrasonic Testing | Anomalies in B-scan |
Eddy Current Testing | Disruptions in electromagnetic field |
Sustained Load Test | Significant decrease in time to failure |
Slow Strain Rate Test | Reduction in ductility or increase in strain rate |
Accurately interpreting Stress Corrosion Cracking Test Results is vital in performing timely and appropriate interventions and preventing catastrophic failures. It is crucial to develop a nuanced understanding of the process, from picking the most suitable techniques for specific situations to accurately interpreting the results.
Exploring Specific Types of Stress Corrosion Cracking
Stress Corrosion Cracking (SCC) is not a uniform phenomenon. It stems from a variety of complex physical and chemical interactions and thus, manifests in different types. Let's explore two of these specific types - Intergranular Stress Corrosion Cracking and Chloride Induced Stress Corrosion Cracking, to deepen the understanding of this destructive mechanism.
Understanding Intergranular Stress Corrosion Cracking
Intergranular Stress Corrosion Cracking (IGSCC) is a specific form of SCC where cracks propagate along the grain boundaries of a material. These grain boundaries are regions where crystal structures of a metal meet and can exhibit different physical and chemical properties compared to the grains or crystals themselves. Thus, they are often more susceptible to chemical attack. When a corrosive environment, tensile stress, and susceptible material intersect, IGSCC can occur.
Intergranular Stress Corrosion Cracking (IGSCC): A phenomenon where cracks propagate along the grain boundaries of the materials, causing weakening and potential failure.
One of the main factors affecting the onset and progression of IGSCC is the chemical composition of the material. Certain alloying elements, when present in higher concentrations at the grain boundaries, can promote IGSCC. In particular, carbon and certain common alloying elements like chromium can form precipitates at the grain boundaries, depleting the surrounding area of crucial corrosion resisting elements and creating a path for IGSCC to take root.
You can envisage the grain boundaries as being 'highways' for crack propagation - once initiated, cracks find it easier to progress along these paths of least resistance.
Chloride Induced Stress Corrosion Cracking Explained
Chloride Induced Stress Corrosion Cracking (CISCC), as the name implies, refers to SCC predominantly caused by the presence of chloride ions. These ions, often found in sea water or de-icing salts, can severely affect particular types of materials, like stainless steels and nickel-based alloys. Understanding CISCC and its impact is critical in industries such as maritime, aerospace, and infrastructure, where elements are frequently exposed to chloride-rich environments.
Chloride Induced Stress Corrosion Cracking (CISCC): This is a type of SCC that occurs in chloride-rich environments. Certain materials, such as stainless steels and nickel-based alloys, are most susceptible.
To understand why chloride ions are so potent, it is important to delve into the mechanism of corrosion. Corrosion, in metals, usually involves the transfer of electrons from the metal atoms (acting as anodes) to the oxygen in water (acting as a cathode). This process is sluggish in the absence of an electrolyte, a role that chloride ions readily fulfill. The chloride ions not only accelerate this reaction but also specifically attack the protective oxide layer on some metals, exposing bare metal to corrosive attacks.
Furthermore, chloride ions can induce pitting, a localized form of corrosion, that generates small pits or cavities on the material surface. These pits can act as initiation sites for SCC, leading to rapid crack propagation under stress.
The Impact of Chloride on Stress Corrosion Cracking
The presence of chloride ions can dramatically impact SCC, accelerating its rate and potentially leading to premature and catastrophic failures. This is especially true for metals like stainless steel, which rely on the formation of a corrosion-resistant oxide layer for protection.
Chloride ions disrupt this protective oxide layer, leading to a scenario termed breakdown of passivity. When a high concentration of chloride ions is present, they compete with the oxygen for the metal's surface. They can displace the oxygen, breaking down the oxide film and exposing the metal underneath to corrosive attack.
This exposure results in accelerated corrosion and potentially the formation of pits - small, localised areas of corrosion that can act as nucleation sites for cracks. Even when the external load is below the yield strength of the material, these pits can concentrate the stress, raising the local intensity and creating favourable conditions for the inception and propagation of SCC.
Therefore, in environments with high chloride ion concentration, a vigilant approach to material selection, design, and maintenance is essential. Regular inspections for signs of pitting, and performing tests to detect the early stages of SCC can mitigate the risks associated with Chloride Induced Stress Corrosion Cracking. Remember, prevention is not only better but often cheaper than a cure.
Stress Corrosion Cracking - Key takeaways
- Stress Corrosion Cracking (SCC): A complex process that includes corrosion-reaction, element-transportation, and mechanical-deformation. Initiation, propagation, and failure are the three major stages of this process.
- Conditions for SCC: The occurrence of SCC requires tensile stress, a material susceptible to SCC and a corrosive environment. The absence of any of these conditions can drastically decrease the chances of SCC.
- Consequences of SCC: SCC can cause structural failure compromising the integrity of buildings, bridges, etc., resulting in significant economic impacts and potential safety risks.
- Stress Corrosion Cracking Test: Testing for the presence and extent of SCC is crucial. Non-destructive and laboratory tests are the major methods used to detect SCC.
- Intergranular Stress Corrosion Cracking (IGSCC) and Chloride Induced Stress Corrosion Cracking: These are specific types of SCC. In IGSCC, cracks propagate along the grain boundaries of a material, whereas in Chloride Induced SCC, cracking occurs due to the presence of chlorides.
Learn faster with the 15 flashcards about Stress Corrosion Cracking
Sign up for free to gain access to all our flashcards.
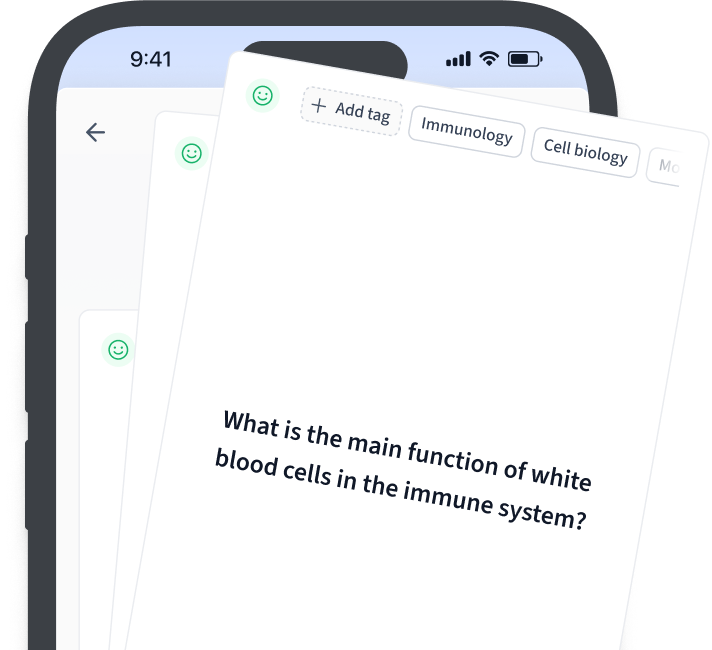
Frequently Asked Questions about Stress Corrosion Cracking
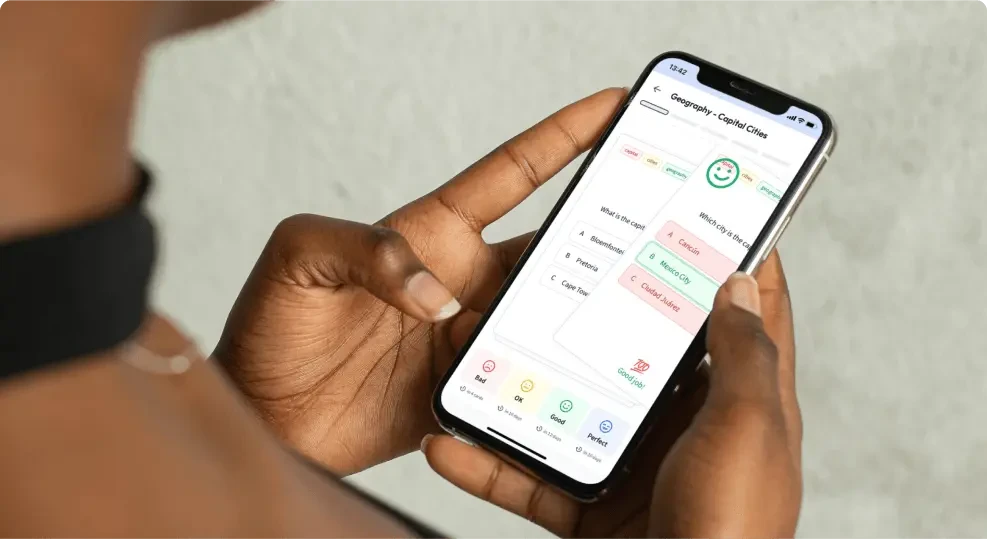
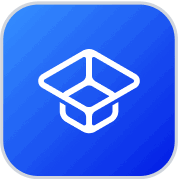
About StudySmarter
StudySmarter is a globally recognized educational technology company, offering a holistic learning platform designed for students of all ages and educational levels. Our platform provides learning support for a wide range of subjects, including STEM, Social Sciences, and Languages and also helps students to successfully master various tests and exams worldwide, such as GCSE, A Level, SAT, ACT, Abitur, and more. We offer an extensive library of learning materials, including interactive flashcards, comprehensive textbook solutions, and detailed explanations. The cutting-edge technology and tools we provide help students create their own learning materials. StudySmarter’s content is not only expert-verified but also regularly updated to ensure accuracy and relevance.
Learn more