Jump to a key chapter
Understanding Work Hardening
When you delve into the fascinating world of engineering, you often come across an important yet seemingly complex phenomenon known as Work Hardening. Fear not! By the end of this section, you'll understand this essential principle and its pivotal role in materials engineering. So, let's embark on this captivating journey together.
Definition of Work Hardening: Breaking it Down
Work Hardening, also known as strain hardening, is an intriguing process that occurs in ductile materials when they are mechanically deformed. This deformation essentially increases the dislocation density within the material, thereby significantly boosting its hardness and strength.
Imagine bending a paperclip back and forth. You'll notice it's easy to bend at first, but as you continue, it becomes noticeably harder to deform. This increased resistance is an uncomplicated demonstration of Work Hardening in action!
Interestingly, Work Hardening can be reversed through another process known as annealing, which involves heating the material to a certain temperature. This process reduces dislocation density and restores ductility, essentially reverting the material back to its pre-deformed state.
Fundamental Principles of Work Hardening
In the realm of materials science, understanding the fundamental principles behind work hardening is crucial. Put simply, work hardening occurs because deformation causes an increase in dislocation density within a material.
Here are the chief principles you need to grasp:- Dislocations occur in a crystal lattice and represent areas of misalignment or misfit.
- When a material is deformed, these dislocations move, usually causing an increase in dislocation density.
- The increased dislocation density impedes further dislocation motion, thus making the material harder and stronger.
Where \sigma represents the applied stress, \sigma_0 denotes the yield stress of the material, d stands for dislocation density, \(n\) is the strain hardening exponent, and \(k\) is the strain hardening constant.
1) Strain Rate | The rate at which strain is applied to the material. |
2) Temperature | The temperature at which the deformation process is carried out can significantly alter its implications. |
Diving into the Work Hardening Process
For you to truly grasp the concept of work hardening, it's essential to break down the process into simpler terms. Let's explore what happens within a material during each stage of work hardening and shed light on how this intricate process enhances properties such as hardness and mechanical strength.
Stages of the Work Hardening Process
Work hardening starts the moment a strain is applied to a ductile material, setting off a chain of reactions within the material's crystal lattice structure. To understand the phenomena better, let's discuss the stages in chronological order:
- Strain-stage: In this preliminary stage, the strain applied causes the dislocations within the material to move, resulting in a significant increase in dislocation density.
- Hardening-phase: As dislocation density increases, it becomes increasingly difficult for the dislocations to move. This rise in resistance to dislocation movement is essentially the hardening phase, where the material's hardness and strength level up.
- Saturation-stage: After a point of strain, an equilibrium state is reached where the rate of new dislocation generation equals the rate at which dislocations are annihilated, leading to a saturation in hardness and strength.
Where \(\sigma\) signifies the stress at a particular deformation level, \(K\) is the strength coefficient, \(\epsilon\) symbolises the true plastic strain, and \(n\) denotes the strain-hardening exponent.
Role of Work Hardening in Materials Engineering
Work hardening has immense significance in the field of materials engineering. Be it in manipulating the properties of metals to make them more resistant to wear and tear or in the creation of hard but ductile materials, work hardening's role cannot be discounted.
Work hardening's influence spans multiple areas:- Metal Forming: Through work hardening, malleable metals gain the required strength and hardness. This is a common technique employed in sheet metal industries.
- Imparting Strength: The intrinsic strength of lower strength metals can be magnified with work hardening.
- Increasing Wear Resistance: Metals subjected to work hardening demonstrate an increased resistance to wear and deformation, making them more durable.
Work Hardening Examples to Consider
To truly appreciate the role of work hardening in our everyday life, let's consider practical examples. Among the most recognisable instances would be the manufacturing process of coins and metal wires.
- Coins: Production of coins involves deforming a metal blank through pressing it between two dies. This metal flow causes strain hardening, making the coins more robust and resistant to everyday wear.
- Metal Wires: Industrial production of metal wires involves deforming a metal rod through a series of dies. Each pass through a die induces more plastic strain, causing work hardening and resulting in a wire that's strong yet flexible.
Exploring the Work Hardening Equation
Work Hardening is an essential process in the field of materials engineering, but to grasp its real essence, a mathematical perspective is needed. Let's delve deeper into the work hardening equation, its significance, and how it helps to understand the science behind the process.
Interpreting the Work Hardening Equation
The mathematical representation of work hardening, also known as the power-law hardening equation, provides a clear view of the relationship between stress and plastic strain in a particular material.
The equation in its common form is given as: \[ \sigma = K \cdot \epsilon^n \]Here, \(\sigma\) represents the stress at a particular deformation level, \(K\) is the strength coefficient, \(\epsilon\) symbolises the true plastic strain, and \(n\) signifies the strain-hardening exponent.
Both the strength coefficient, \(K\), and the strain-hardening exponent, \(n\), are material-dependent parameters and can be determined through experimental stress-strain tests.
The parameters in the equation can be defined as follows:Stress \(\sigma\) | This stress is the total load acting on the specimen divided by the original cross-sectional area of the specimen. |
Strength coefficient \(K\) | It is a measure of material's resistance to deformation, and typically increases with increase in dislocation density or material impurities. |
True plastic strain \(\epsilon\) | It is the natural logarithm of the instantaneous gauge length divided by the original gauge length. |
Strain-hardening exponent \(n\) | It describes the increase in stress required to continue deformation as the material is strain-hardened. |
Tools for Work Hardening
Suppose you've sought to understand the process of Work Hardening, deciphered the essential equations behind it and grasped its role in engineering. Now it's time to move from conceptual understanding to practical implementation. To that end, let’s focus on the tools and equipment used to achieve Work Hardening, taking you one step further in demystifying this fascinating aspect of materials engineering.
An Overview of Work Hardening Equipment
Equipment used for work hardening are primarily tools that apply mechanical stress to a material. The choice of a specific tool, however, primarily depends on the desired application, type of material, and the output sought.
The major types of equipment essential to the process of work hardening include:- Rolling Mills: Fundamentally used in the metal forming industry, rolling mills compress the metal between rotating rolls to cause deformation. There are several types of rolling mills - two-high, three-high, four-high, cluster mill, and tandem rolling mill, each with different arrangements of rolls and used for specific kinds of applications.
- Presses: Mechanical presses are used to apply various force levels to a workpiece. They are commonly used in sheet metal forming and deep drawing operations. The work piece is placed between two dies, and the mechanical press applies force, causing the desired deformation.
- Hammers: Manual or mechanised hammers are used to cause deformation in the workpiece through a series of blows. Although a more traditional method, this is still used in some industries where small scale or specific shape deformations are required.
- Swaging Machines: These are used for a specific kind of forming named swaging, which involves radial deformation of the work piece. The mechanism includes a set of dies that hammer radially on the workpiece, causing it to deform and take the shape of the die.
As well as these key pieces of equipment, there is also a myriad of accessory tools used in conjunction with the above for specific purposes. These can include dies, mandrels, punches, and much more. It's crucial to note that the choice of tools and their configuration will hinge on the nature of the material and the desired hardening effect.
Using Work Hardening Equipment: A Practical Approach
Managing work hardening equipment necessitates an understanding of not just theoretical principles but also handling techniques. Whether you're in a complex industrial setup or a smaller workshop, having a sound knowledge of how to use the equipment to achieve work hardening can be a real game-changer.
Here's a practical, step-by-step breakdown of how to use the different types of equipment:
- Rolling Mills: The first step is to select the appropriate mill based on the application. Once the mill is ready, the material to be hardened is placed between the rolls. As the rolls turn, they apply compressive stress on the material causing it to deform plastically. The process can be repeated until the desired hardness and strength are achieved.
- Presses: With a mechanical press, a die with the desired shape is chosen, and the material is placed on it. When the press is activated, it applies force on the workpiece, causing it to deform and take the shape of the die. This process can be performed multiple times (known as multiple-pass drawing) to achieve increased hardness and strength.
- Hammers: When using a hammer, the workpiece is held firm, and the hammer is used to strike the material, causing deformation. The precision and repeatability of this method can be lower, but for some applications, it's a beneficial choice.
- Swaging Machines: The workpiece is placed in a swaging machine and the dies are set up in such a way to achieve the desired shape. When the machine is turned on, the dies apply radial force on the workpiece, causing it to deform and take the shape of the die.
Work Hardening - Key takeaways
- Definition of Work Hardening: This refers to the process that occurs in ductile materials when they are mechanically deformed, leading to an increase in dislocation density within the material and thereby significantly boosting its hardness and strength.
- Work Hardening Process: This process involves an initial strain stage where dislocations within the material move and dislocation density increases. It is followed by a hardening phase where the material's hardness and strength increase, and finally a saturation stage occurs where an equilibrium state is reached.
- Work Hardening Equation: Represented as \(\sigma = K \cdot \epsilon^n\), this formula is a mathematical perspective of the work hardening process. The stress (\(\sigma\)) at a particular deformation level, the strength coefficient (K), the true plastic strain (\(\epsilon\)), and the strain-hardening exponent (n) are variables that explain the relationship between stress and plastic strain.
- Work Hardening Examples: Examples in everyday life include the production of coins and metal wires, both of which involve deforming a metal through a series of dies, inducing work hardening and resulting in a product that's durable and resilient.
- Work Hardening Equipment: Main tools include rolling mills, presses, hammers, and swaging machines. The choice of tool depends on the type of material and the desired outcome. Understanding how to use these tools is crucial in materials engineering.
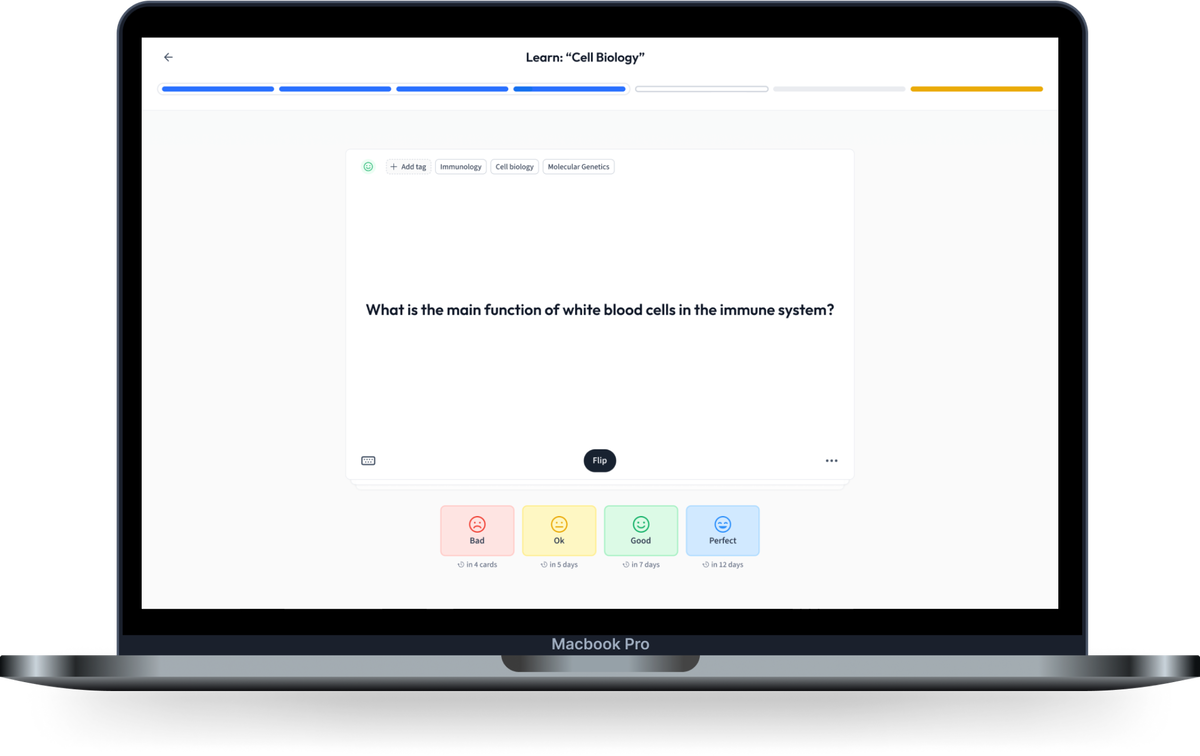
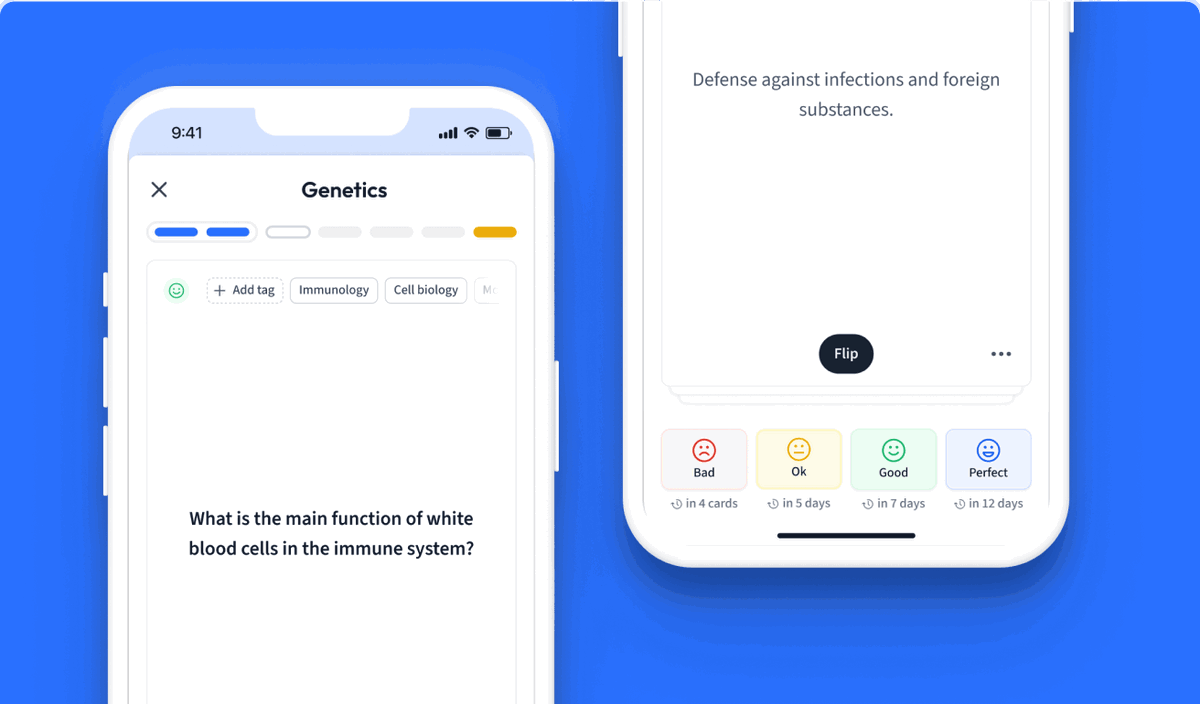
Learn with 12 Work Hardening flashcards in the free StudySmarter app
We have 14,000 flashcards about Dynamic Landscapes.
Already have an account? Log in
Frequently Asked Questions about Work Hardening
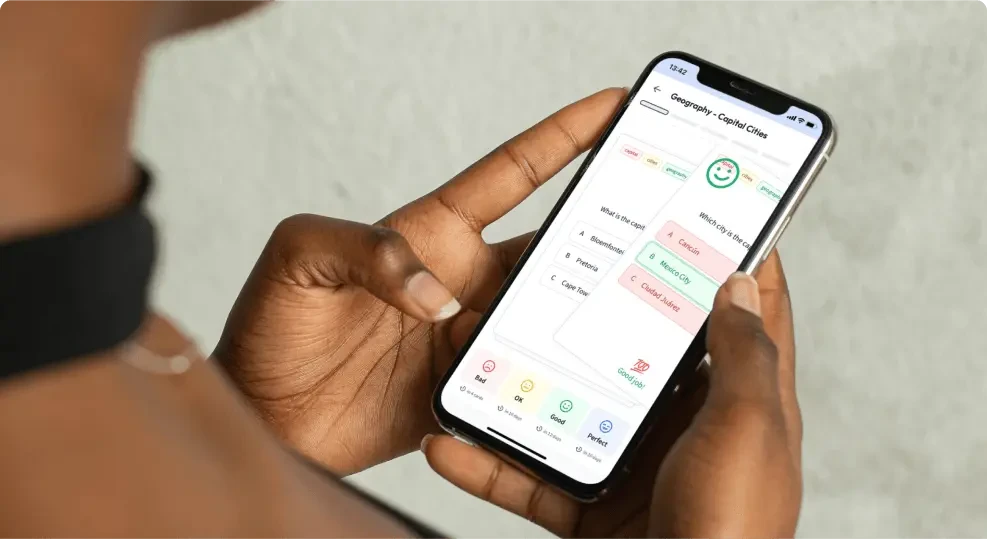
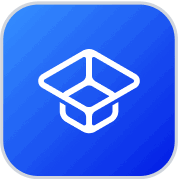
About StudySmarter
StudySmarter is a globally recognized educational technology company, offering a holistic learning platform designed for students of all ages and educational levels. Our platform provides learning support for a wide range of subjects, including STEM, Social Sciences, and Languages and also helps students to successfully master various tests and exams worldwide, such as GCSE, A Level, SAT, ACT, Abitur, and more. We offer an extensive library of learning materials, including interactive flashcards, comprehensive textbook solutions, and detailed explanations. The cutting-edge technology and tools we provide help students create their own learning materials. StudySmarter’s content is not only expert-verified but also regularly updated to ensure accuracy and relevance.
Learn more