Jump to a key chapter
Aeroelastic Flutter Definition
Aeroelastic Flutter is a dynamic instability that occurs in structures subject to aerodynamic forces, particularly when flexibility and wind forces interact. This phenomenon often leads to oscillations that can increase in amplitude, potentially causing structural failure if not appropriately managed.
Aeroelastic Flutter Explained
Understanding aeroelastic flutter is crucial for designing stable and safe structures, especially in the field of aerospace engineering. It occurs when aerodynamic forces, structural elasticity, and inertial effects combine in a way that amplifies vibrations instead of damping them.
- Structural Components Involved: Typically involves wings, tails, and control surfaces in aircraft.
- Conditions: Critical aeroelastic flutter conditions are reached when increases in structural deformation lead to increased aerodynamic force.
- Risks: Sustained oscillations can result in structural damage or catastrophic failure.
The interaction between these forces can create dangerous oscillations when the natural frequency of the structure aligns with the aerodynamic forces at certain speeds. This is known as reaching a flutter speed.
Aeroelastic Component | Description |
---|---|
Elasticity | Refers to the flexibility of the structure |
Inertia | Mass and distribution of mass in the structure |
Aerodynamics | External forces due to airflow |
Example: In 1940, the Tacoma Narrows Bridge collapsed due to aeroelastic flutter. Strong winds caused torsional oscillations that increased in amplitude, eventually leading to the bridge's failure.
Hint: Engineers use high-fidelity simulations to predict and avoid aeroelastic flutter in the design phase of structures.
A deeper understanding of aeroelastic flutter involves sophisticated mathematical models. These models incorporate the principles of fluid dynamics and structural dynamics. The flutter speed is a crucial analytic component, calculated using complex eigenvalue analyses, which provides insights into the onset of the instability. By understanding these principles, engineers can innovate advanced materials and design strategies to mitigate the risks associated with aeroelastic flutter.
Aeroelastic Flutter Causes
Aeroelastic flutter is a complex interaction of aerodynamic, elastic, and inertial forces leading to potentially destructive oscillations in structures. To understand the causes of aeroelastic flutter, we must delve into the mechanical and environmental factors that contribute to this phenomenon.
Mechanical Factors in Aeroelastic Flutter
Mechanical factors play a critical role in the onset of aeroelastic flutter. These primarily involve the inherent properties of the structure and how they interact with dynamic forces.
- Structural Elasticity: The flexibility of a structure determines its natural frequencies and mode shapes, which are critical in flutter. A high degree of elasticity might increase susceptibility.
- Mass Distribution: The distribution of mass affects the inertial forces experienced by the structure, influencing how it responds to external aerodynamic forces.
- Structural Damping: This property helps reduce vibrations. Low damping can enhance flutter, increasing instability risk.
The balance between these factors determines the flutter speed, exceeding which could trigger flutter. Engineers calculate the critical flutter speed using formulas that consider these mechanical properties.
For instance, the equation for estimating the flutter speed can be represented as:
\[V_f = \frac{1}{2} \times \frac{K}{\rho \times b} \times \frac{\text{Structural Stiffness}}{\text{Mass per Unit Length}}\]
Example: In aircraft wings, too much flexibility without adequate damping can lead to flutter at lower speeds, requiring engineers to either stiffen the wing or increase damping.
Environmental Influences on Aeroelastic Flutter
Besides mechanical factors, environmental conditions significantly impact the occurrence of aeroelastic flutter. Various atmospheric conditions and external forces can exacerbate or mitigate flutter risk.
- Wind Speed and Direction: Variability in wind speed and direction can instantly alter the aerodynamic forces on a structure, such as a bridge or an aircraft wing.
- Temperature Variations: Changes in temperature can affect the material properties, such as elongation or contraction, altering stiffness and flutter characteristics.
- Turbulence: Atmospheric turbulence adds random, dynamic loads that can push structures to their flutter boundaries.
To predict and manage these influences, engineers employ computational fluid dynamics (CFD) to simulate fluid-structure interactions in variable environmental conditions.
In a deeper exploration, it's essential to consider the complex relationship between Mach number and flutter onset. The Mach number represents the speed of an object relative to the speed of sound. At high subsonic and transonic speeds, aerodynamic forces vary vastly, affecting the structure differently. To calculate the flutter margin, a refined interplay between the Mach number and structural frequencies must be considered:
\[\frac{\text{Flutter Margin}}{\text{Mach number}^2} = \text{Adjusted Dynamic Pressure} \times \text{Structural Parameters}\]
Managing these is critical in designing aircraft wings and other flexible structures operating at high speeds, ensuring the structural integrity and safety of the systems.
Hint: Proper maintenance and inspections can detect early signs of potential flutter-related issues, preventing costly repairs or accidents.
Aeroelastic Flutter Analysis
Aeroelastic flutter analysis is vital in determining the stability of engineering structures exposed to aerodynamic forces. It involves understanding the dynamic interaction between the structure's elasticity, inertia, and environmental forces.
Techniques for Aeroelastic Flutter Analysis
Several techniques are employed to assess and mitigate aeroelastic flutter. Each technique offers unique insights into the structural behavior under aerodynamic loads.
- Computational Fluid Dynamics (CFD): This technique uses simulations to model airflow over a structure, predicting potential flutter conditions.
- Wind Tunnel Testing: Physical models are tested under controlled conditions to observe flutter behavior and validate computational models.
- Analytical Methods: This involves solving equations that describe the aerodynamic forces and structural responses to determine critical flutter speeds.
Each method requires consideration of potential nonlinear behaviors that could affect the reliability of the predictions.
Example: A combination of CFD simulation and wind tunnel experiments is used in the aerospace industry to ensure that new aircraft designs do not experience flutter at operational speeds.
Hint: The integration of machine learning in flutter analysis is growing, offering potential improvements in predicting non-linear aeroelastic behaviors.
Aeroelastic Flutter Equations
The mathematical representation of aeroelastic flutter involves complex equations that link structural dynamics and fluid dynamics. A typical flutter equation might include terms for damping, stiffness, and aerodynamic forces.
Consider the simplified flutter speed equation:
\[V_f = \sqrt{\frac{2 \times K}{\rho \times S}} \]
where:
- \(V_f\) is the flutter speed.
- \(K\) represents stiffness of the structure.
- \(\rho\) is the air density.
- \(S\) is the reference area of the structure.
Such equations help predict the speeds at which aeroelastic instability may occur.
To further delve into the subject, one may consider the modal analysis approach in flutter study. This method involves the decomposition of structural responses into mode shapes, which are then analyzed under aerodynamic loading:
\[\text{Modal Damping Ratio (}\zeta\text{)} = \frac{1}{2} \times \frac{\text{Aerodynamic Force}}{\text{Structural Stiffness}}\]
This advanced method allows for a thorough understanding of which mode shapes are primarily responsible for initiating flutter, enabling targeted design modifications to increase structural stability and delay the onset of adverse aeroelastic effects.
Aeroelastic Flutter vs Resonance
Exploring the distinct differences and interactions between aeroelastic flutter and resonance is crucial for understanding structural dynamics in engineering applications. While both phenomena involve oscillations, their causes and effects on structures differ significantly.
Differences Between Aeroelastic Flutter and Resonance
Aeroelastic flutter and resonance are two different forms of dynamic instability in structures. Here’s how they differ:
- Causes: Aeroelastic flutter results from a complex interaction between aerodynamic forces, structural flexibility, and inertia. In contrast, resonance occurs when a structure is subjected to periodic forces at a frequency matching its natural frequency.
- Nature of Oscillations: Flutter leads to self-excited oscillations, growing in amplitude even without external periodic forces. On the other hand, resonance amplifies oscillations due to external periodic forces.
- Impact on Structures: Aeroelastic flutter can cause catastrophic failure rapidly, whereas resonance may gradually lead to damage if not controlled.
An example of resonance could be when a child swings higher after being pushed at the correct intervals, which matches the swing's natural frequency.
Example: The collapse of the Tacoma Narrows Bridge in 1940 was primarily due to aeroelastic flutter, not resonance, as the wind-induced torsional oscillations weren't due to matching frequencies but rather a dynamic instability between aerodynamic forces and the bridge's natural mode.
Hint: Understanding both phenomena is essential in designing structures that can withstand various dynamic loads without failing.
Interactions of Aeroelastic Flutter and Resonance
Although aeroelastic flutter and resonance are distinct, they can sometimes interact in complex ways in engineering systems. This interaction can complicate the analysis of structural stability.
- Combined Effects: Flutter might induce conditions that enhance resonance or vice-versa. For instance, residual energy from flutter could instigate resonant behavior in a different mode.
- Aerodynamic Damping: Changes in aerodynamic damping caused by flutter can alter the dynamic response of structures, potentially affecting resonance characteristics.
- Critical Speeds: The speed at which aeroelastic flutter occurs may be close to resonant frequencies, requiring precision in speed management in systems like aircraft or bridges.
For a deeper understanding, it's useful to consider the formula for critical flutter speed and natural frequency:
Flutter Speed Formula: \[V_c = \sqrt{\frac{2 \pi \times E \times I}{\rho \times C_D \times L^2}}\]
Whereas Resonance Frequency Formula: \[f_r = \frac{1}{2\pi} \sqrt{\frac{K}{M}}\]
Understanding how speed affects both flutter and resonance is crucial for safety in aviation and structural engineering.
A deeper dive into their interaction reveals that the simultaneous occurrence of aeroelastic flutter and resonance might lead to a phenomenon called flutter resonance coupling. This occurs when one mode of vibration excites another leading to a compounded instability effect. Engineers use coupled mode analysis to dissect this complex interaction.
Consider the primary equation for coupled mode analysis:
\[\begin{bmatrix} M_{11} & M_{12} \ M_{21} & M_{22} \end{bmatrix} \begin{bmatrix} \ddot{q}_1 \ \ddot{q}_2 \end{bmatrix} + \begin{bmatrix} C_{11} & C_{12} \ C_{21} & C_{22} \end{bmatrix} \begin{bmatrix} \dot{q}_1 \ \dot{q}_2 \end{bmatrix} + \begin{bmatrix} K_{11} & K_{12} \ K_{21} & K_{22} \end{bmatrix} \begin{bmatrix} q_1 \ q_2 \end{bmatrix} = \begin{bmatrix} F_1 \ F_2 \end{bmatrix}\]
This matrix equation shows the coupled interaction of modal coordinates \(q_1\) and \(q_2\), offering insights into the complex vibration modes that can’t be captured by analyzing each mode independently.
aeroelastic flutter - Key takeaways
- Aeroelastic Flutter Definition: Dynamic instability in structures due to aerodynamic forces, interacting with structural flexibility and inertia, leading to potentially destructive oscillations.
- Aeroelastic Flutter Causes: Caused by a complex interaction of aerodynamic, elastic, and inertial forces, with critical flutter speed determined by structural properties and external conditions.
- Aeroelastic Flutter Analysis: Involves assessing stability through Computational Fluid Dynamics, wind tunnel testing, and analytical methods to predict and mitigate flutter conditions.
- Aeroelastic Flutter Equations: Mathematical models incorporate damping, stiffness, and aerodynamic forces, with simplified flutter speed equations used to gauge instability onset.
- Aeroelastic Flutter vs Resonance: Flutter involves self-excited oscillations from aerodynamic interaction, whereas resonance arises from periodic forces matching natural frequency, causing different failure impacts.
- Aeroelastic Flutter Explained: Critical in aerospace design, it involves vibrations amplified by aerodynamic and structural dynamics, with engineers using simulations to prevent flutter-induced failures.
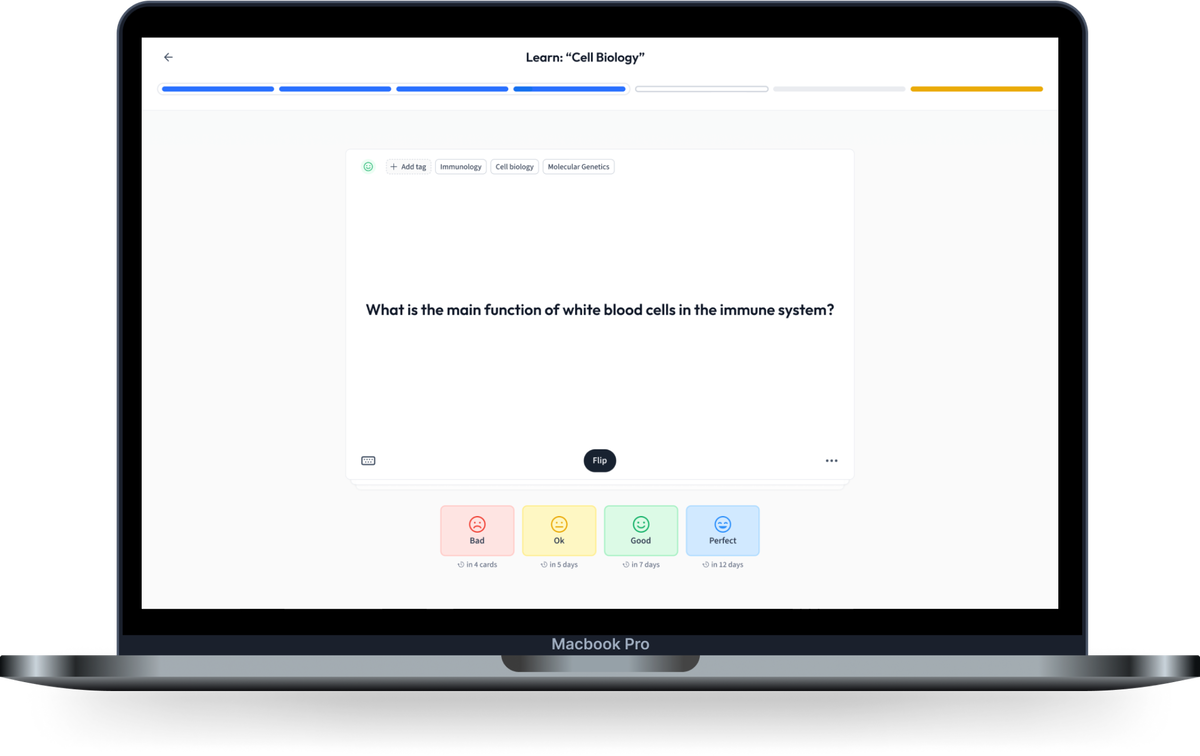
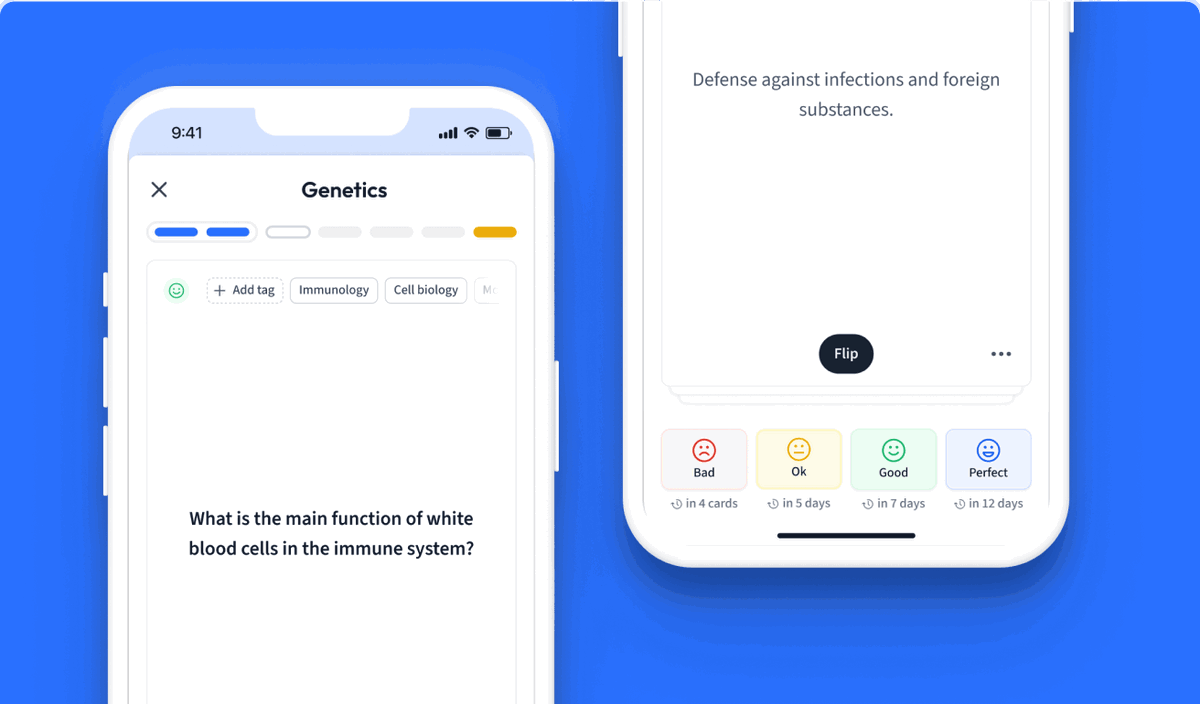
Learn with 12 aeroelastic flutter flashcards in the free StudySmarter app
Already have an account? Log in
Frequently Asked Questions about aeroelastic flutter
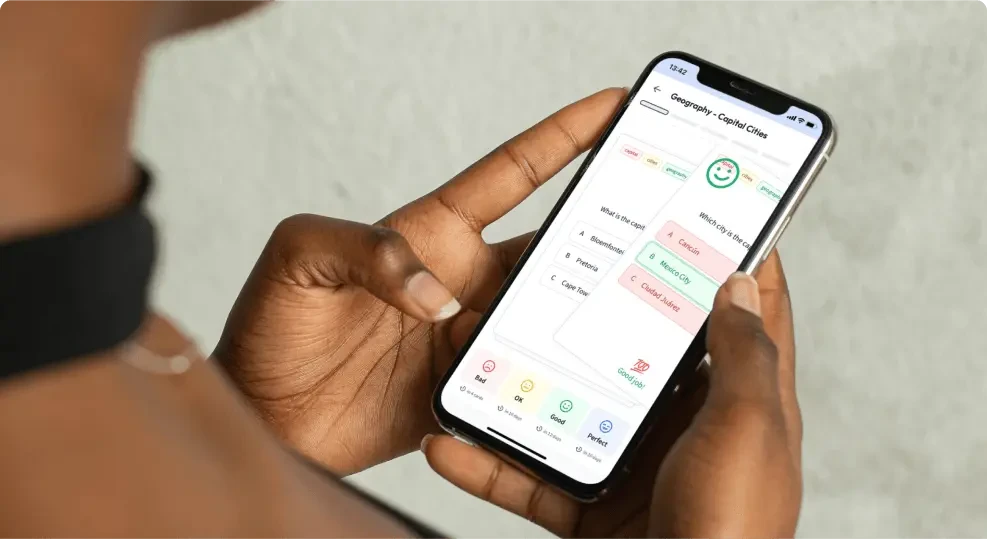
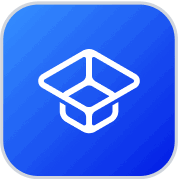
About StudySmarter
StudySmarter is a globally recognized educational technology company, offering a holistic learning platform designed for students of all ages and educational levels. Our platform provides learning support for a wide range of subjects, including STEM, Social Sciences, and Languages and also helps students to successfully master various tests and exams worldwide, such as GCSE, A Level, SAT, ACT, Abitur, and more. We offer an extensive library of learning materials, including interactive flashcards, comprehensive textbook solutions, and detailed explanations. The cutting-edge technology and tools we provide help students create their own learning materials. StudySmarter’s content is not only expert-verified but also regularly updated to ensure accuracy and relevance.
Learn more