Jump to a key chapter
Aeroengine Components Overview
Understanding the different components of an aeroengine is crucial for anyone interested in how aircraft are powered. Whether you're looking at a jet engine or a turbofan, knowing the terminology and the specifics of each part forms the backbone of aerospace engineering knowledge.
Aeroengine Component Terminology
In the world of aeroengines, specific terminology helps you understand and identify different parts and their functions. Here are some key terms you'll come across:
- Compressor: This component compresses the air to increase the pressure before it enters the combustion chamber.
- Combustion Chamber: The stage where fuel is mixed with high-pressure air and ignited to produce high-temperature exhaust gases.
- Turbine: Converts the energy from the high-temperature gases into mechanical work to spin the compressor.
- Nozzle: Accelerates exhaust gases leaving the jet engine to generate thrust.
The terms 'turbine' and 'compressor' often come up repeatedly in different engine types, as they play central roles in the engine's operation.
Jet Engine Parts Explained
A jet engine is a complex machine that operates on the principles of aerodynamics and thermodynamics. Its key components include:
- Intake: Responsible for taking in air at high speed and delivering it to the compressor.
- Compressor: Increases the air's pressure, making it ready for combustion.
- Combustion Chamber: Burns the air-fuel mixture to create high-energy output.
- Turbine: Makes use of the exhaust gases to drive the compressor and other accessories.
- Nozzle: Releases the exhaust gases to produce thrust, propelling the aircraft forward.
For example, when the jet engine is operating, air enters through the intake, gets compressed, fuel is added and ignited in the combustion chamber, then the resulting high-speed gases spin the turbine, which in turn spins the compressor, with the remaining gases expelled through the nozzle to create thrust.
Jet engines often operate at extreme temperatures and pressures; hence, they are built with advanced materials and technologies. Thermal efficiency and aerodynamic efficiency are key considerations in the design, allowing for greater thrust and lower fuel consumption. This is why newer jet engines utilize materials such as titanium alloys and ceramic composites, capable of withstanding the harshest of engine conditions while maintaining durability and performance.
TurboFan Engine Components
The turbofan engine is a variant of the jet engine and is widely used in commercial aircraft due to its efficiency and noise reduction capabilities. Key components include:
- Fan: A large diameter fan sits at the front of the engine, which directs air into the engine and bypasses some air around the engine core.
- Bypass Duct: The path around the core engine where the bypass air flows, increasing thrust without additional fuel burn.
- Low-pressure Compressor: First stage that compresses air moderately before it enters the high-pressure compressor.
- High-pressure Compressor: Further compresses the air to a higher pressure level.
- Low-pressure Turbine: Extracts energy from the exhaust gases to spin the fan and low-pressure compressor.
- High-pressure Turbine: Powers the high-pressure compressor through mechanical work extracted from exhaust gases.
Turbofan Engine: A type of jet engine with a fan that increases thrust by directing part of the airflow around the core engine, improving fuel efficiency and reducing noise.
Compressor and Turbine Blades in Aeroengine
Compressor and turbine blades are vital components in an aeroengine, influencing both the efficiency and performance of the engine. These blades undergo precise engineering to withstand extreme conditions such as high temperatures and rotational forces.
Functions of Compressor and Turbine Blades
Compressor and turbine blades function in tandem to manage the airflow and energy within an engine. Here’s a closer look into their roles:
- Compressor Blades: These blades increase the pressure of incoming air before it enters the combustion chamber. By steadily compressing the airflow, they contribute to optimal fuel mixing and combustion. The compression ratio can be expressed mathematically as \(\frac{P_{\text{out}}}{P_{\text{in}}}\), where \(P_{\text{out}}\) is the output pressure and \(P_{\text{in}}\)\ is the input pressure.
- Turbine Blades: Post-combustion, high-energy gases hit the turbine blades, transferring energy to drive the compressor. The energy transfer can be represented by the formula \(E = \frac{1}{2}mv^2\), where \(m\) is mass and \(v\) is velocity.
The angle and shape of both compressor and turbine blades are critical as they determine the efficiency of compression and energy extraction.
For instance, in a typical engine cycle: air enters the compressor, where blades increase its pressure. Inside the combustion chamber, this compressed air combines with fuel, igniting a reaction. The resultant high-energy gases turn the turbine, powering the compressor in a continuous cycle.
The design of the compressor and turbine blades is a marvel of engineering. Aeroelasticity plays a significant role, ensuring the blades do not fail under mechanical stress. Engineers use advanced computational techniques to simulate these stresses and vibrations, aiming for an optimal balance between strength and weight. Material science innovations, like the development of single-crystal superalloys, have significantly improved the efficiency of these components. These materials resist creep—slow deformation over time—under high temperatures and rotational speeds, critical for the longevity and reliability of the engine.
Aeroengine Design Engineering Insights
When designing aeroengines, engineers focus on numerous factors to optimize performance and safety. These factors often include:
- Thermal Efficiency: This involves maximizing the conversion of fuel energy into mechanical energy. It can be described mathematically as \(\frac{\text{Work Output}}{\text{Heat Input}}\).
- Aerodynamic Design: Ensuring that air flows smoothly within the engine minimizes drag and increases thrust.
- Material Selection: Choosing materials that withstand high stresses and temperatures, like titanium and nickel-based alloys, is crucial.
Thermal Efficiency: A measure of how well an engine converts heat into useful work.
Aeroengine Component Functions
Every aeroengine component has a specific function crucial to the operation of the entire system. Understanding these functions provides a better grasp of how airplanes fly and the engineering marvels behind them.
Understanding Key Aeroengine Functions
In an aeroengine, each component plays a unique role, contributing to the engine's overall operation and efficacy. Here’s a breakdown of key functions:
- Air Intake: Captures incoming air needed for combustion.
- Compressor: Increases the pressure of the air to prepare it for the combustion process.
- Combustion Chamber: Provides the area where fuel is mixed with compressed air and ignited, generating high-energy gases.
- Turbine: Converts heat energy from the exhaust gases into mechanical energy, driving the compressor.
- Exhaust: Releases combustion remnants and aids thrust production by accelerating expelled gases.
Compressor: A key component that pressurizes air before it enters the combustion chamber, crucial for achieving high energy output.
Consider the compressor: As the aircraft flies, air is drawn into the engine at high velocity. The compressor increases the air pressure before it meets the fuel in the combustion chamber. This ensures a powerful combustion process, leading to efficient propulsion.
A deeper dive into engine thermodynamics reveals the importance of the Brayton cycle in aeroengine operations. This cycle, consisting of constant pressure heating and cooling, helps in understanding the efficiency of the engine. Compressor efficiency, defined by the ability to increase air pressure without excessive energy loss, is critical. Engineers focus on minimizing entropy increases in the compressor to maximize overall engine performance. Innovations such as axial and centrifugal compressors have furthered this efficiency, allowing higher pressure ratios essential for modern aviation needs.
How Aeroengine Components Work Together
Aeroengine components do not operate in isolation. They are intricately linked to ensure seamless power generation and aircraft propulsion.Let’s see how these components interact:
- Air Intake and Compressor: These two components work hand-in-hand to ensure that an adequate supply of compressed air is ready for the combustion chamber.
- Compressor and Combustion Chamber: The compressor provides high-pressure air necessary for efficient fuel mixing and ignition in the chamber.
- Combustion Chamber and Turbine: The combustion process generates high-speed gases that drive the turbine, converting thermal energy into mechanical energy.
- Turbine and Exhaust: The turbine transfers used energy into the compressor, while the residual gas is accelerated out through the exhaust to generate additional thrust.
Maintaining precise synchronization among components is crucial for optimal aeroengine performance and efficiency. Even small deviations can significantly affect engine thrust and fuel consumption.
Studying Aeroengine Design Engineering
Studying aeroengine design engineering involves understanding the intricate workings of aircraft engines. It merges principles of thermodynamics, materials science, and fluid dynamics to develop efficient and reliable engines for aviation.
Importance of Aeroengine Components in Design
Aeroengine components play a pivotal role in the design and functionality of aircraft engines. Each part needs to work harmoniously for the engine to perform efficiently.
- Compressor: Increases air pressure before the combustion phase, impacting engine output and efficiency.
- Combustion Chamber: Mixes fuel with compressed air, igniting a reaction to produce thrust-driving gases.
- Turbine: Converts exhaust gas energy into mechanical work, running the compressor cycle.
- Nozzle: Directs high-velocity gases outwards, creating necessary thrust for flight.
Aeroengine Components: Parts such as the compressor, turbine, combustion chamber, and nozzle that collectively enable the engine's function to provide thrust in aircraft.
A practical example demonstrates the importance: in a jet engine, as air is compressed, it means more oxygen is present, enabling complete combustion of fuel, which results in greater thrust. Efficiency is represented by the formula: \[\text{Efficiency} = \frac{\text{Work Output}}{\text{Heat Input}}\]. Increasing compressor efficiency directly influences this equation.
The design process of aeroengine components includes simulations using Computational Fluid Dynamics (CFD) to optimize airflow and heat transfer. Material selections like single-crystal superalloys are critical due to their capability to withstand extreme temperatures and prolong engine lifespan. Additionally, engineers utilize Finite Element Analysis (FEA) to model stress responses within engine components, ensuring designs can handle diverse operational stresses. Modern engines also incorporate lightweight composite materials to boost fuel efficiency without compromising strength, showcasing the complex interactions between materials and design architecture.
Future Trends in Aeroengine Design Engineering
The future of aeroengine design engineering is focused on enhancing fuel efficiency, reducing emissions, and incorporating new technologies.
- Hybrid-Electric Engines: Combining traditional engines with electric power to improve fuel economy and reduce carbon footprints.
- Advanced Materials: Utilizing materials like ceramic matrix composites that withstand higher temperatures while reducing weight.
- 3D Printing: Facilitating complex component designs with reduced manufacturing time and cost.
- AI and Machine Learning: Used for predictive maintenance and design optimization to increase reliability and performance.
Incorporating AI in engine design and maintenance holds the potential to not only predict and prevent failures but also optimize design for aerodynamic improvements.
aeroengine components - Key takeaways
- Aeroengine components play a crucial role in aircraft propulsion, including parts like compressors and turbines.
- Compressor and turbine blades are essential in regulating airflow and energy within an engine.
- Aeroengine design engineering involves thermodynamics, materials science, and fluid dynamics for efficient engine performance.
- Key jet engine parts include the compressor, combustion chamber, turbine, and nozzle.
- Turbofan engine components feature a fan, bypass duct, and low/high-pressure compressors and turbines, boosting efficiency.
- Understanding aeroengine component functions and terminology is vital for aerospace engineering.
Learn faster with the 12 flashcards about aeroengine components
Sign up for free to gain access to all our flashcards.
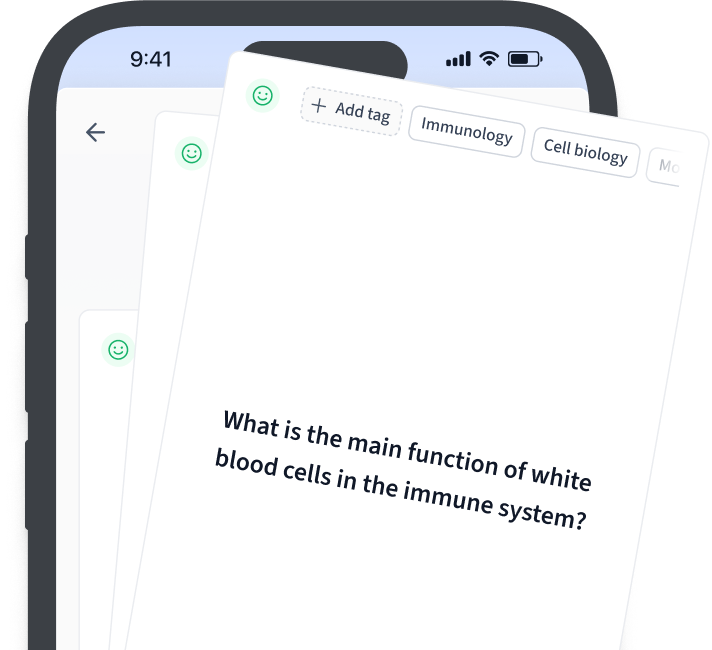
Frequently Asked Questions about aeroengine components
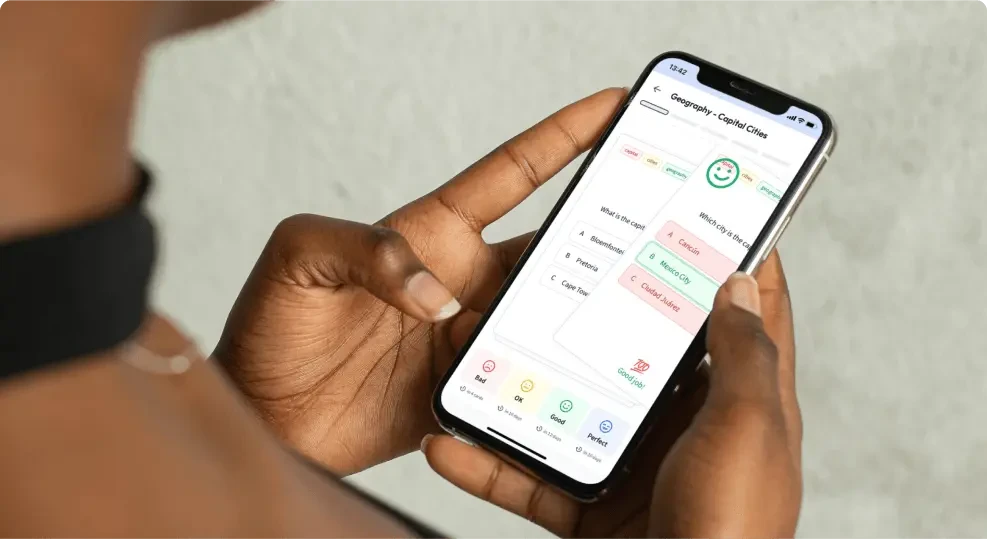
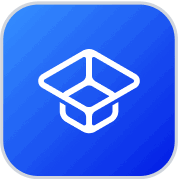
About StudySmarter
StudySmarter is a globally recognized educational technology company, offering a holistic learning platform designed for students of all ages and educational levels. Our platform provides learning support for a wide range of subjects, including STEM, Social Sciences, and Languages and also helps students to successfully master various tests and exams worldwide, such as GCSE, A Level, SAT, ACT, Abitur, and more. We offer an extensive library of learning materials, including interactive flashcards, comprehensive textbook solutions, and detailed explanations. The cutting-edge technology and tools we provide help students create their own learning materials. StudySmarter’s content is not only expert-verified but also regularly updated to ensure accuracy and relevance.
Learn more