Jump to a key chapter
Biomechanical Properties Definition
When understanding the biomechanical properties, it's essential to recognize that this term refers to the study of material properties of biological tissues. These properties are foundational in joining the principles of mechanical engineering with biological systems, allowing the evaluation of how these natural materials respond under various forms of stress and strain.
Biomechanical Properties: It encompasses the study and analysis of physical properties such as elasticity, strength, and adaptability of biological tissues under mechanical forces.
Key Aspects of Biomechanical Properties
Biomechanical properties are classified into several key aspects that help in answering questions related to the behavior of biological tissues under specific conditions:
- Elasticity: This refers to the ability of a material to return to its original shape after deformation. For biological tissues, this is crucial as it determines their functional effectiveness.
- Viscoelasticity: Most biological tissues exhibit viscoelastic properties, meaning they have both viscous and elastic characteristics. This results in time-dependent strain.
- Strength: This measures the maximum stress that a material can withstand. Biological tissues have varying strengths based on their function and composition.
Consider the human bone which exhibits distinct biomechanical properties. Its strength allows it to support the body's weight and withstand physical activities, while its elasticity helps in absorbing shocks.
Delving deeper into viscoelasticity, the behavior can be expressed mathematically using the Kelvin-Voigt model which combines both spring (elastic) and dashpot (viscous) elements in parallel. The governing equation is given by: \[\sigma(t) = E \epsilon(t) + \eta \frac{d\epsilon(t)}{dt}\]where \(\sigma(t)\) is the stress, \(E\) is the modulus of elasticity, \(\eta\) is the viscosity coefficient, and \(\epsilon(t)\) is the strain.
Always consider the biological function of the tissue to better understand its biomechanical properties.
Biomechanical Properties of Bone
Understanding the biomechanical properties of bone is crucial, as it enables the analysis of how bones sustain the forces they encounter daily. Bones serve as the framework of our bodies and their structural integrity relies on mechanical properties like stiffness, compressive strength, and toughness.
Biomechanics Mechanical Properties of Living Tissues
Living tissues, such as bones and ligaments, demonstrate a unique set of mechanical properties that are essential for their function. These include:
- Stiffness: The resistance of a material to deformation. For bones, stiffness is necessary to maintain body posture and support weight.
- Compressive Strength: The ability to withstand compressive forces. This property is essential for the load-bearing nature of bones.
- Toughness: The capacity to absorb energy and plastically deform without fracturing.
Stress-Strain Relationship: This relationship expresses how a material deforms under stress. Mathematically, it's defined as \[ \sigma = E \epsilon \] where \( \sigma \) is the stress, \( E \) is the modulus of elasticity, and \( \epsilon \) is the strain.
Consider the tibia bone in the human leg that experiences significant compressive forces during activities such as walking and running. Its role highlights the importance of compressive strength in preventing fractures.
Biomechanical properties of tissues often vary between individuals depending on factors like age, activity level, and health condition.
In-depth analysis of bones can be done using computational modeling techniques such as Finite Element Analysis (FEA). FEA allows simulating different loading conditions to predict how stress and strain distribute across bone structures. The governing equations blend material properties with geometry to provide detailed insights.
Biomechanical Properties Techniques Applied to Bone
Examining the biomechanical properties of bone involves several techniques that provide both qualitative and quantitative insights:
- Material Testing: Laboratory tests that apply various forces to bone samples to measure their strength and elasticity.
- Imaging Techniques: Such as MRI and CT scans, which help visualize the internal structure of bones and assess their quality.
- Mathematical Modeling: Utilizing equations like \[ \sigma = E \epsilon \] to predict bone behavior under different conditions.
Mathematical modeling is often used in orthopedic surgery planning to tailor implants and ensure they match the mechanical properties of a patient's bone.
Biomechanical Properties of Connective Tissue
Connective tissues play a crucial role in the human body, providing support and structure. Their biomechanical properties are integral to their function. Understanding these properties helps in predicting how these tissues behave under different conditions, essential in areas like biomedical engineering and physical therapy.
Understanding Biomechanical Properties of Ligaments
Ligaments are key components of connective tissues, mostly known for their role in connecting bones at joints. They are flexible yet strong, and understanding their biomechanical properties requires focusing on several factors:
- Elasticity: Refers to the ability of ligaments to stretch and return to their original shape, important for joint flexibility.
- Tensile Strength: The capacity of a ligament to withstand tension, supporting joint stability.
- Viscoelasticity: Ligaments exhibit both viscous and elastic characteristics, which influence their time-dependent strain response.
Stress-Strain Relationship in Ligaments: Expressed as \( \sigma = E \epsilon \), where \( \sigma \) is stress, \( E \) is the modulus of elasticity, and \( \epsilon \) is strain.
An example of ligament analysis can be seen in anterior cruciate ligament (ACL) studies where its elasticity and tensile strength are vital in preventing knee injuries during movements like jumping or abrupt changes in direction.
The viscoelastic nature of ligaments can be further explored by considering the Maxwell model, which represents them using a series of a spring and a dashpot. The constitutive equation \[\sigma(t) = \eta \frac{d\epsilon(t)}{dt} + E \epsilon(t)\] helps to predict the complex interplay between stress and strain under dynamic loads, crucial for designing rehabilitation protocols.
Changes in ligament properties often occur due to aging or physical activity levels, affecting overall joint function.
Techniques for Analyzing Connective Tissue
Analyzing the biomechanical properties of connective tissues, including ligaments, involves a range of techniques and approaches:
- Mechanical Testing: Experiments using uniaxial tensile tests to measure the stress-strain behavior.
- Imaging Tools: High-resolution MRI or ultrasound to monitor structural changes over time.
- Computational Modeling: Methods like Finite Element Analysis to simulate tissue response under various loading conditions.
Finite Element Analysis serves as a comprehensive tool in predicting how connective tissues like ligaments respond to surgical interventions or external supports.
Biomechanical Properties Techniques
The analysis of biomechanical properties is critical in understanding how biological tissues respond to various forces. Numerous techniques are employed for this purpose, each offering unique insights into material behavior.
Methods to Measure Biomechanical Properties
There are diverse methods used to measure the biomechanical properties of tissues. These approaches help in evaluating parameters such as strength, elasticity, and deformability:
- Tensile Testing: This method involves stretching a material to understand its elasticity and tensile strength. The stress-strain curve obtained is essential to describe the material's behavior.
- Compression Testing: It assesses compressive strength by applying pressure until the sample deforms or fractures.
- Shear Testing: This determines how a material reacts to shear stress, important for soft tissues like skin or cartilage.
Each of these methods yields a stress-strain graph, which is fundamental in defining material properties mathematically:
The stress-strain relationship is given by \( \sigma = E \epsilon \), where \( \sigma \) represents stress, \( E \) is the modulus of elasticity, and \( \epsilon \) denotes strain.
Young's Modulus: This is a measure of the stiffness of a material, given by the ratio of stress to strain in the linear elastic region of the stress-strain curve.
Consider a tensile test on a biological material such as a ligament. By applying a known force and measuring the deformation, you can utilize the stress-strain curve to determine its mechanical properties like elastic and failure strengths.
The mathematical characterization of these properties can be further analyzed using viscoelastic models like the Maxwell and Kelvin-Voigt models. These models consider the material's response as a combination of elastic springs and viscous dashpots. The Maxwell model equation is: \[\sigma(t) = \eta \frac{d\epsilon(t)}{dt} + E \epsilon(t)\]. This provides insight into the time-dependent behavior of the material under dynamic conditions.
High precision instruments and computational techniques significantly enhance the accuracy of these measurements.
Advances in Techniques for Living Tissues
Recent technological advances have revolutionized the study of living tissues with regards to their biomechanical properties:
- Non-Invasive Imaging: Techniques like MRI and ultrasonography allow for the assessment of internal structures without physical intervention, leading to better understanding of tissue properties in their natural state.
- Computational Models: Computer simulations using Finite Element Analysis (FEA) are now commonplace to predict tissue behavior and plan medical interventions.
- Biomechanical Sensors: Devices that provide real-time data on tissue performance, aiding in areas such as sports science and rehabilitation.
Finite Element Analysis often serves as the backbone for advanced simulations in biomechanics. For example, predicting the stress distribution in joint replacements can guide biomedical engineers in improving prosthetic designs.
biomechanical properties - Key takeaways
- Biomechanical Properties Definition: The study of material properties of biological tissues, combining mechanical engineering principles with biological systems to analyze responses to stress and strain.
- Biomechanical Properties of Bone: Key mechanical properties include stiffness, compressive strength, and toughness, crucial for supporting weight and withstanding physical activities.
- Biomechanical Properties of Connective Tissue: Focus on elasticity, tensile strength, and viscoelasticity essential for functionality, particularly in ligaments connecting bones at joints.
- Biomechanics Mechanical Properties of Living Tissues: Include stiffness, compressive strength, and toughness, with stress-strain relationships critical for understanding deformation under stress.
- Biomechanical Properties Techniques: Methods like tensile, compression, and shear testing, along with advanced imaging and computational modeling, are employed to evaluate tissue mechanics.
- Biomechanical Advanced Techniques: Advances involve non-invasive imaging, computational models like Finite Element Analysis (FEA), and biomechanical sensors for assessing living tissue behavior.
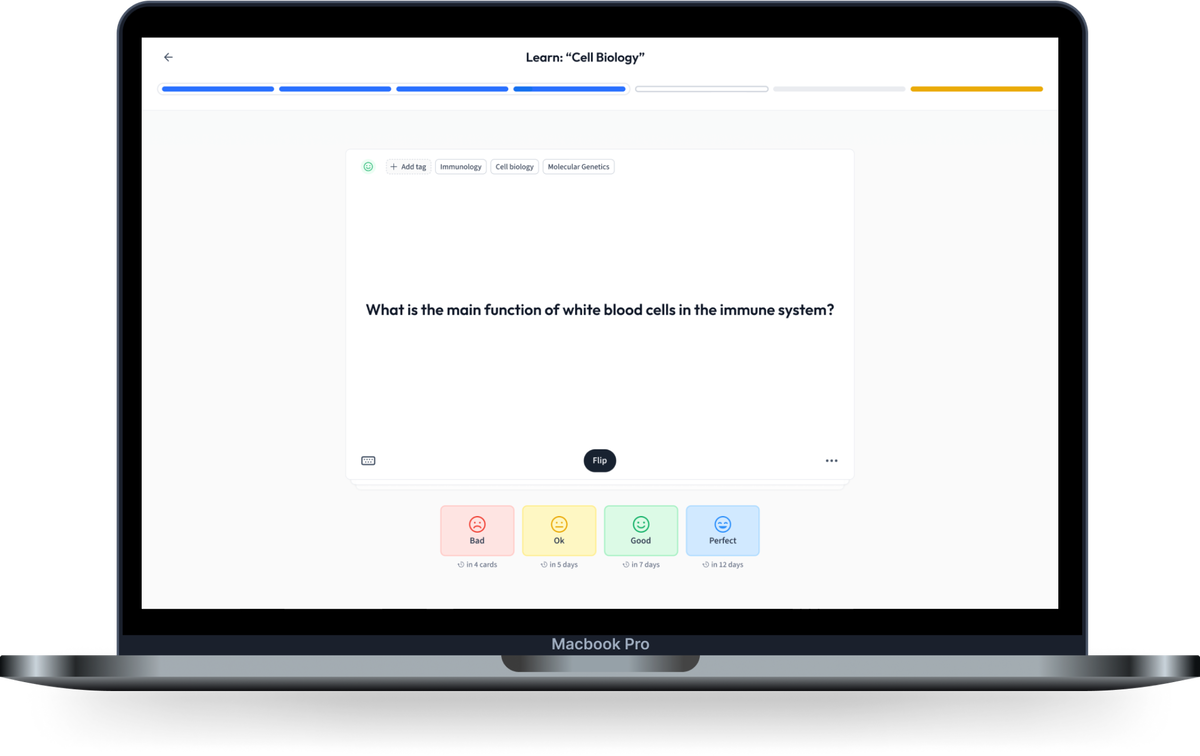
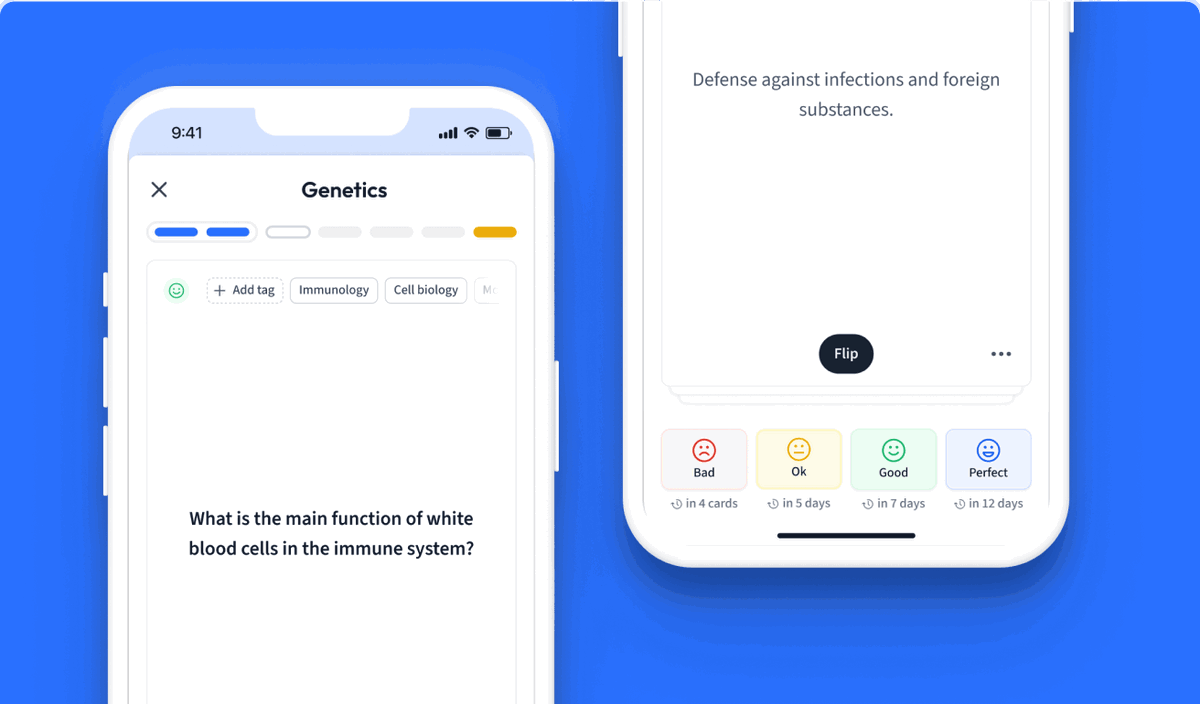
Learn with 12 biomechanical properties flashcards in the free StudySmarter app
Already have an account? Log in
Frequently Asked Questions about biomechanical properties
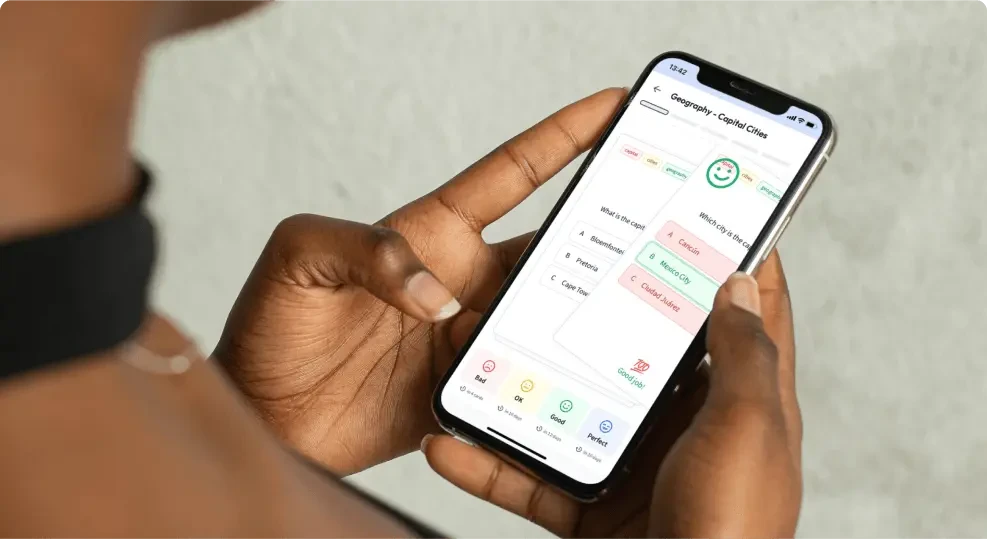
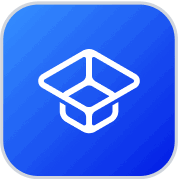
About StudySmarter
StudySmarter is a globally recognized educational technology company, offering a holistic learning platform designed for students of all ages and educational levels. Our platform provides learning support for a wide range of subjects, including STEM, Social Sciences, and Languages and also helps students to successfully master various tests and exams worldwide, such as GCSE, A Level, SAT, ACT, Abitur, and more. We offer an extensive library of learning materials, including interactive flashcards, comprehensive textbook solutions, and detailed explanations. The cutting-edge technology and tools we provide help students create their own learning materials. StudySmarter’s content is not only expert-verified but also regularly updated to ensure accuracy and relevance.
Learn more