Jump to a key chapter
Understanding Biomechanical Simulation
In the realm of engineering, biomechanical simulation plays a crucial role in understanding how mechanical principles apply to biological systems. It combines the principles of physics with the complexities of biological organisms.
Definition of Biomechanical Simulation
Biomechanical Simulation refers to the computer-based modeling and simulation of biological systems using the principles of mechanics. These simulations allow researchers to analyze how forces interact within the human body or other biological entities.
The primary objective of biomechanical simulation is to predict the movement and forces within a biological structure. By using mathematical models, scientists can simulate:
- Joint and muscle function
- Stress distribution
- Fluid dynamics in biological tissues
Suppose you are simulating a running figure. You would need to consider the various forces acting on the joints and muscles, similar to calculating the force on each footstep using Newton's Second Law: \( F = m \cdot a \). Here, if the mass is constant and acceleration changes, the force exerted by muscles and joints can be calculated to optimize movement and minimize injury.
History of Biomechanical Simulation
Biomechanical simulation has evolved significantly over the years. Initially, human and animal biomechanics were studied using simple mechanical models and physical experiments.
The field of biomechanics dates back to the early 16th century. However, the technical concept of simulating biological movements began taking shape in the mid-20th century with advances in computer technology. As computers became more powerful, they allowed for more complex modeling and simulation tasks.
In the 1970s, the concept of using finite element analysis (FEA) provided new opportunities for simulating mechanical behaviors of biological tissues. Biomechanical simulation was further advanced by the introduction of 3D imaging techniques like MRI and CT scans, leading to more accurate representations of the anatomical structures. With continuous technological advancements, these models continue to become more sophisticated, catering to personalized medicine and robot-assisted surgeries.
Biomechanical Simulation Models
Exploring the intricacies of biomechanical simulation models offers invaluable insights into how mechanical principles operate within biological systems. Such models are extensively utilized in medical research and athletic performance analysis.
Introduction to Biomechanical Simulation Model
A biomechanical simulation model is a computer-generated model that replicates biological systems to study motion and various forces. These models often integrate advanced computational methods to predict behaviors under a variety of conditions.
Biomechanical Simulation involves the systematic application of mechanical laws to biological systems, using digitally crafted models to replicate these interactions. A common application includes analyzing the stress and strain on biological tissues like bones and cartilages.
To construct a biomechanical simulation model, a range of data inputs is required:
- Geometric representation of anatomical features using 3D imaging.
- Material properties for simulating tissue characteristics.
- Boundary conditions to define how forces are applied.
- Contact mechanics to model interactions between structures.
Mathematically, these models can use the equation for stress \( \sigma = \frac{F}{A} \), where \( \sigma \) is stress, \( F \) is the force applied, and \( A \) is the area over which the force is distributed.
Consider simulating the knee joint during a squat movement. The force exerted by muscles and ligaments can be represented by calculating the torque around the joint. Using the formula \( \tau = r \times F \), where \( \tau \) is the torque, \( r \) is the lever arm (distance from the pivot point), and \( F \) is the force.
Utilizing motion capture technologies can enhance the accuracy of the initial geometric representation in biomechanical simulation models.
Development of Biomechanical Models for Soft Tissue Simulation
Developing biomechanical models specifically for soft tissue simulation requires an in-depth understanding of tissue mechanics and physiological responses. These models are pivotal in applications such as surgical simulation and injury prevention.
The mechanical behavior of soft tissues such as muscles, ligaments, and skin is considerably complex due to their heterogeneous and anisotropic nature. Simulating soft tissues often relies on the implementation of hyperelastic material models to capture non-linear behaviors: \( W = C_1 (I_1 - 3) + C_2 (I_2 - 3) \), where \( W \) is the strain energy function, \( C_1 \) and \( C_2 \) are material constants, and \( I_1 \), \( I_2 \) are the principal invariants of the strain tensor.
The computational approach often employs the finite element method (FEM) to discretize the tissues, enabling detailed insight into how internal structures deform under various conditions. Additionally, advances in artificial intelligence are increasingly being incorporated to enhance the predictive accuracy of soft tissue models.
Biomechanical Human Simulation Techniques
Biomechanical human simulation techniques allow us to replicate and analyze human motion, assisting in diverse fields such as medicine, sports, and robotics. Through different modeling approaches, these techniques simulate the complexities of human biomechanics.
Approaches to Biomechanical Human Simulation
There are multiple approaches to biomechanical human simulation, each designed to cater to specific requirements and areas of study. Here are some common methods:
- Finite Element Analysis (FEA): Utilizes computational algorithms to model and analyze complex structures under various conditions by breaking them into smaller, finite parts.
- Multi-body Dynamics (MBD): Focuses on the interaction of rigid body systems and accounts for connected parts through joints and forces.
- Musculoskeletal Modeling: Bridges the gap between motion and forces, modeling muscles, bones, and joints to understand movement mechanisms.
- Computational Fluid Dynamics (CFD): Analyzes the interaction between fluid flows and biological tissues, important for cardiovascular and respiratory systems.
Consider the application of multi-body dynamics in sports. An athlete's motion during a sprint can be broken down using MBD models, where each limb is treated as a rigid body, allowing the calculation of joint torques and muscle forces to optimize performance and reduce the risk of injury.
Combining multiple simulation approaches often results in more comprehensive models that can simulate intricate biological interactions.
Examples of Biomechanical Simulations in Human Studies
Biomechanical simulations have been profoundly impactful in human studies, providing insights across various domains. Below are some noteworthy examples:
1. Orthopedic Research: Simulations are used to predict stress on implants and bone structures, improving the design and longevity of artificial joints.2. Gait Analysis: Biomechanical simulations assist in identifying abnormal gait patterns in patients with prosthetics, leading to better rehabilitation strategies.3. Injury Prevention: By simulating the strain on ligaments during athletic activities, biomechanical studies help develop guidelines to reduce sports injuries.
In-depth simulations in orthopedics involve creating patient-specific models for surgery planning. These models can include precise geometric representations obtained from imaging techniques like MRI and CT scans. Utilizing finite element analysis, these models can evaluate stress distribution over time after an implant is placed. The equation for evaluating stress frequently used is \( \sigma = \frac{F}{A} \), where \( \sigma \) is stress, \( F \) is force, and \( A \) is the area the force is applied to. The capability to analyze the longevity of implants pre-surgery helps in reducing the risk of post-surgical complications significantly.
Engineering Applications and Principles
The implementation of biomechanical simulation in engineering paves the way for advancements in both medical and technological fields. By understanding the interaction of mechanical forces with biological systems, engineers can innovate across diverse applications.
Applications of Biomechanical Simulation in Engineering
In engineering, biomechanical simulation facilitates the development of technologies that enhance human quality of life. Whether applied in medical devices or the automotive sector, these simulations have broad potential.
- Medical Device Design: Simulations can predict how devices interact with human tissues, thus improving their design and reducing the risk of complications. This is vital in creating devices like pacemakers and prosthetics.
- Ergonomic Design: In product design, simulations help in modeling human interaction with tools and furniture to maximize comfort and efficiency.
- Automotive Safety: Crash simulations involve biomechanical models to predict injuries and improve car safety features.
Consider a scenario where a prosthetic limb is being developed. By using computational models, engineers can simulate limb movements, verify the structural integrity of the design, and predict the energy costs of walking with the prosthetic. This involves calculating the biomechanical efficiency using the relation: \[ e = \frac{W_{\text{output}}}{W_{\text{input}}} \] Where \( e \) is efficiency, \( W_{\text{output}} \) is the work done by the prosthetic, and \( W_{\text{input}} \) is the energy supplied by the user.
In automotive simulations, incorporating virtual crash test dummies with detailed biomechanics can significantly improve safety outcomes.
Key Engineering Principles in Biomechanical Simulation
Several engineering principles are foundational to biomechanical simulations. Understanding these principles enhances the simulation's accuracy and applicability in real-world scenarios.
- Mechanics of Materials: Recognizes how materials deform under various loads, expressed mathematically by stress and strain relations such as Hooke's Law: \[ \sigma = E \epsilon \] Where \( \sigma \) is stress, \( E \) is the modulus of elasticity, and \( \epsilon \) is strain.
- Fluid Dynamics: Crucial in simulating blood flow and respiratory systems, utilizing the Navier-Stokes equations to model fluid behavior.
- Control Systems: In robotic applications, understanding feedback loops and control laws is vital for replicating realistic movements.
Advanced simulations may integrate multi-physics environments, addressing interactions between mechanical, thermal, and fluid systems. Techniques such as the finite element method (FEM) allow these intricate simulations to be thoroughly analyzed. The FEM process involves discretizing the continuum model into finite elements, facilitating the resolution of complex stress and displacement fields through the equation: \[ \text{K} \text{u} = \text{F} \] Where \( \text{K} \) is the stiffness matrix, \( \text{u} \) is the displacement vector, and \( \text{F} \) is the force vector. This enables engineers to predict behaviors at different scales and optimize design accordingly.
biomechanical simulation - Key takeaways
- Biomechanical Simulation: Computer-based modeling using mechanics to analyze forces in biological systems.
- Biomechanical Simulation Models: Computer-generated models replicating biological systems to study motion and forces.
- Biomechanical Models for Soft Tissue Simulation: Use hyperelastic material models to capture non-linear behavior of tissues like muscles and skin.
- Biomechanical Human Simulation Techniques: Include approaches like Finite Element Analysis, Multi-body Dynamics, and Musculoskeletal Modeling.
- Examples of Biomechanical Simulations: Used in orthopedic research, gait analysis, and injury prevention for improved outcomes.
- Applications in Engineering: Used in medical device design, ergonomic design, and automotive safety, utilizing principles like mechanics of materials and fluid dynamics.
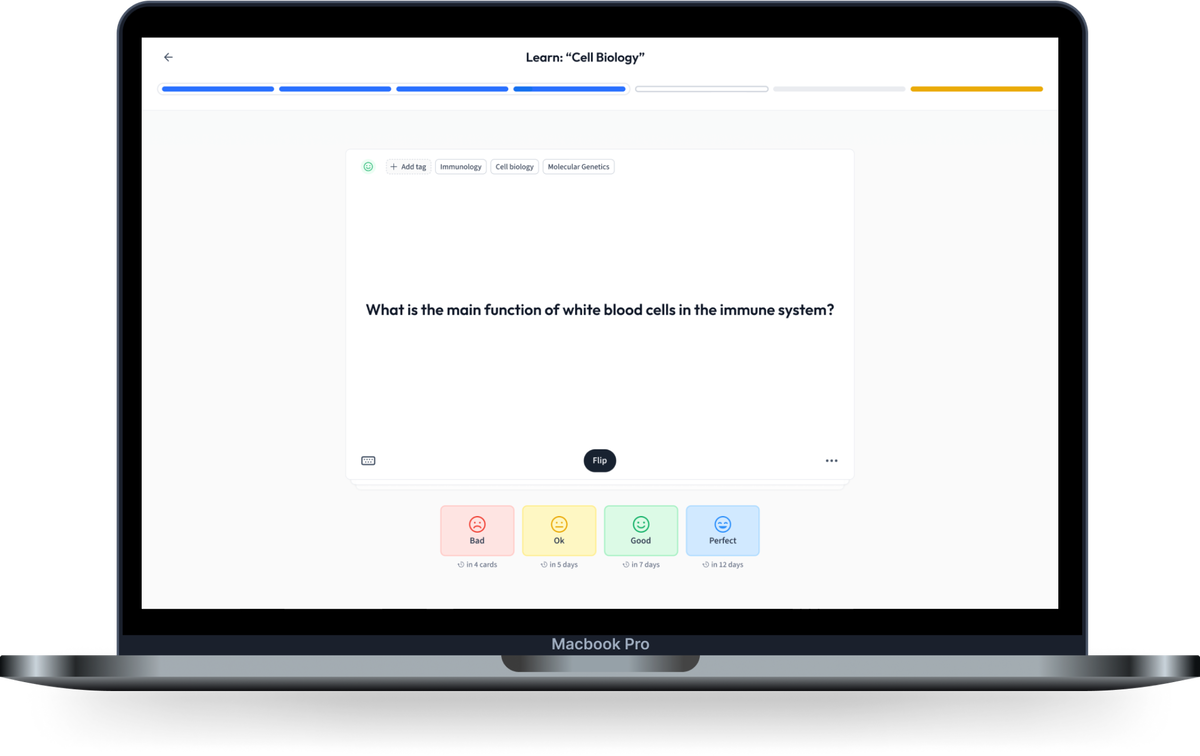
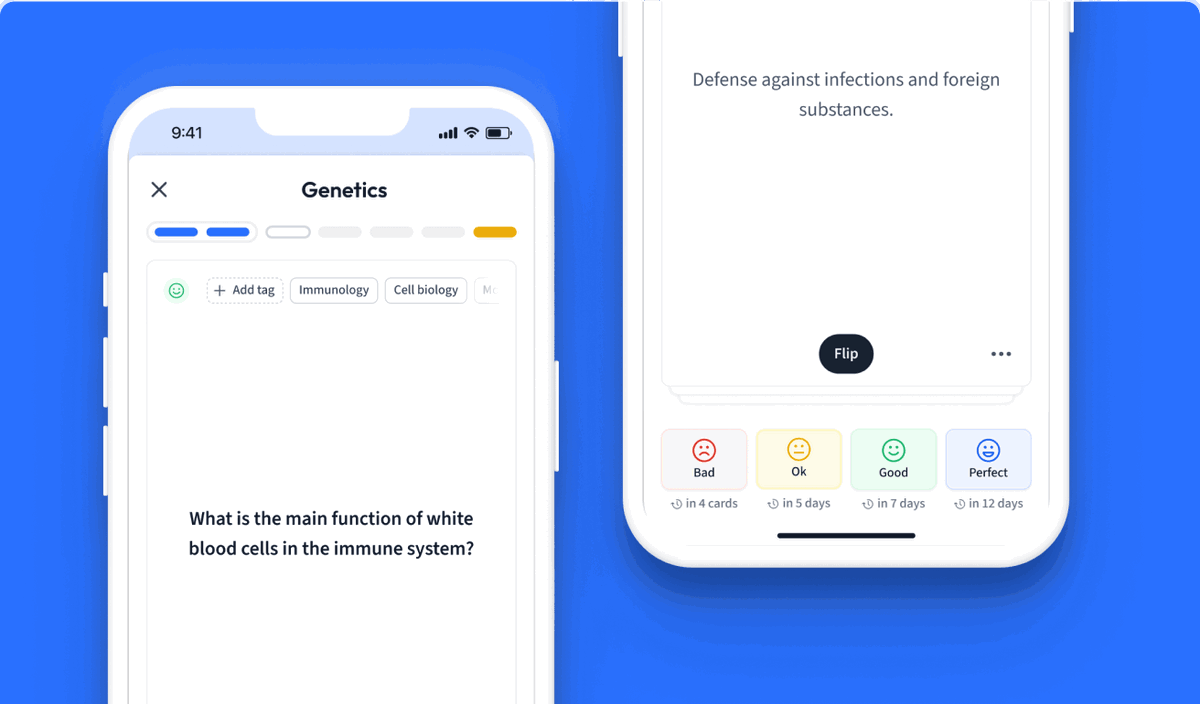
Learn with 12 biomechanical simulation flashcards in the free StudySmarter app
Already have an account? Log in
Frequently Asked Questions about biomechanical simulation
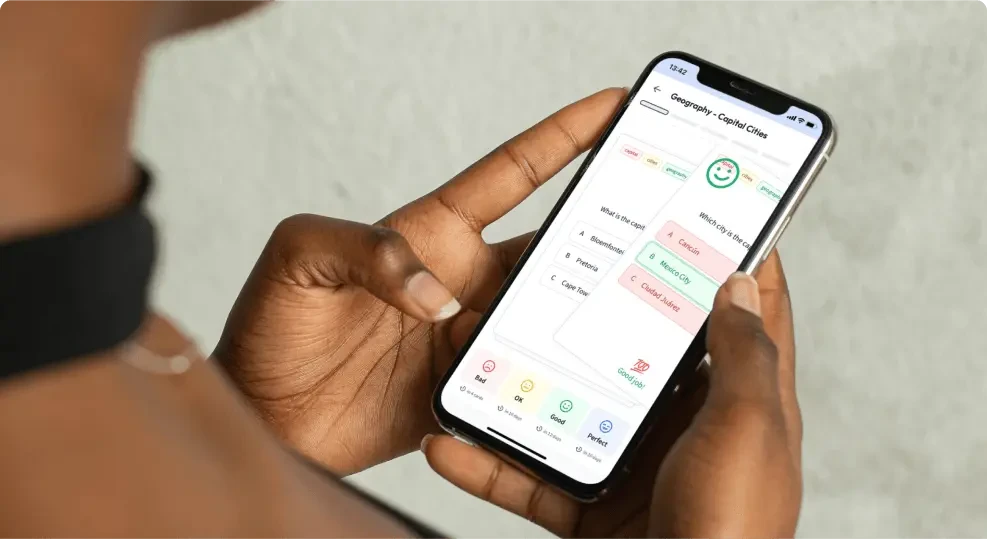
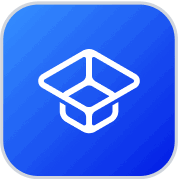
About StudySmarter
StudySmarter is a globally recognized educational technology company, offering a holistic learning platform designed for students of all ages and educational levels. Our platform provides learning support for a wide range of subjects, including STEM, Social Sciences, and Languages and also helps students to successfully master various tests and exams worldwide, such as GCSE, A Level, SAT, ACT, Abitur, and more. We offer an extensive library of learning materials, including interactive flashcards, comprehensive textbook solutions, and detailed explanations. The cutting-edge technology and tools we provide help students create their own learning materials. StudySmarter’s content is not only expert-verified but also regularly updated to ensure accuracy and relevance.
Learn more