Jump to a key chapter
Definition of Biomechanical Systems
Biomechanical systems play a crucial role in the integration of biological and mechanical principles. These systems are designed to mimic or interact with the complex workings of living organisms. They combine engineering techniques with biological sciences to solve problems and create innovations in fields like healthcare, sports, and robotics. By understanding biomechanical systems, you gain insight into how mechanical laws apply to biological structures.
Understanding Biomechanical Systems
A biomechanical system can be described as an intersecting point between engineering, physics, and biology. These systems utilize mechanical elements and laws to study movements and forces in biological entities. The study of these systems can include analysis of joint movement, muscle function, and limb mechanics in both humans and animals.
A biomechanical system is an integrated framework that leverages mechanical principles within biological contexts to achieve certain functions or processes.
An excellent example of a biomechanical system is an artificial limb. These prosthetics are designed to replicate the movement and control of natural limbs, using principles of mechanics and electronics to enable motion and interaction with the environment.
When analyzing these systems, you often deal with complexities like:
- Muscle force calculations
- Joint angle analysis
- Pressure distribution mapping
In-depth analysis of joint kinematics—another aspect of biomechanical systems—often involves complex models that predict motion paths based on joint rotations and forces. Such models might use transformation matrices to describe orientational changes in a holistic 3D environment, which cater to specific needs like predicting a runner's gait or analyzing the ergonomic efficiency in workplace tasks. Often, you will find sophisticated simulations running computational paradigms known as the finite element method (FEM) that help in determining the stress and strain responses of biological tissues under various load conditions.
Interestingly, biomechanical systems are not confined solely to macroscopic elements. Molecular biomechanics investigates the mechanical properties of proteins and cells, bridging an intriguing field of nano-biomechanics.
Understanding Biomechanical Systems
Biomechanical systems are at the intersection of engineering and biology. These systems blend mechanical principles with biological processes, enabling the study of motion, forces, and energy within living organisms. From designing prosthetic limbs to creating body-support exoskeletons, understanding these systems is crucial for innovations in healthcare and technology.At their core, biomechanical systems involve analyzing parameters like movement, force, and torque. This involves complex mathematical modeling and physiological considerations to simulate natural biological processes.
Core Concepts of Biomechanical Systems
To delve into biomechanical systems, you must first grasp the key principles. These include:
- Kinematics - the study of motion without regard to mass or force.
- Kinetics - the examination of forces acting on bodies to cause movement.
- Dynamics - the analysis of forces and their effects on motion.
- Designing devices that assist or replace biological functions.
- Improving rehabilitation processes through better understanding of movement patterns.
- Creating realistic anatomical models for educational and surgical planning purposes.
Consider the design of orthotic devices as a biomechanical system in action. These devices support and stabilize body parts, often correcting musculoskeletal anomalies. For instance, a foot orthosis might be crafted to redistribute forces during walking, which is calculated using mechanics equations. The force exerted by the orthosis can be modeled by Hooke's Law: \[ F = -kx \], where \( k \) is the stiffness of the material, and \( x \) is the displacement from the neutral position.
A fascinating aspect of biomechanical systems is the intricacies involved in gait analysis. This involves monitoring walking patterns to understand the biomechanics of movement. Using modern technology, sensors can calculate parameters such as joint angles, stride lengths, and force distributions with remarkable precision. For full analysis, algorithms may involve inverse dynamics, where joint torques and muscle forces are calculated retrospectively. Formulations guiding these explorations might include the dynamic equilibrium equations: \[ \tau = I \times \frac{d\theta}{dt} \], where \( \tau \) is torque, \( I \) is the moment of inertia, and \( \theta \) is the angular displacement.
Did you know that some advanced biomedical devices are designed using principles from both biomechanical systems and artificial intelligence to predict and enhance functional performance?
Techniques in Biomechanical Engineering
In the fascinating field of biomechanical engineering, several techniques stand out for their effectiveness in analyzing and replicating biological processes. These techniques blend mechanical engineering with biological evidence to develop solutions that enhance quality of life. Here's a closer look at some pivotal approaches:
1. Computational Simulations
Computational simulations are fundamental in biomechanical engineering. They allow for the modeling of complex interactions within biological systems using computational algorithms. These simulations are used to:
- Predict how tissues might respond to different forces.
- Simulate biological movements in a virtual environment.
- Improve the design of medical implants by evaluating their interaction with the human body.
Finite Element Analysis (FEA) is a numerical method used in engineering to predict how objects react to external forces, heat, vibration, and other physical effects by dividing them into smaller, manageable parts known as elements.
The use of FEA in biomechanics is incredibly versatile. For example, in modeling bone mechanics, the bone geometry is reconstructed from imaging data and meshed for analysis. Loading conditions mimicking real-world scenarios can be applied, making it possible to study the bone's response. The stress distribution can be visualized using \[ \sigma = \frac{F}{A} \] where \( \sigma \) is the stress, \( F \) is the force applied, and \( A \) is the cross-sectional area.
2. Motion Analysis Systems
Motion analysis systems are employed to study the dynamics of human movement. By capturing motion data using cameras and sensors, engineers and scientists can:
- Understand the mechanics of human gait and posture.
- Enhance sports performance through detailed analysis of athletes' movements.
- Design better assistive devices like prosthetics.
A practical example of motion analysis is the assessment of runner's stride. By using markers and tracking technology, every movement component is examined to optimize performance and reduce injury risk. This can be vital for designing training programs and custom footwear.
3. Biomechanics of Soft Tissue
Soft tissues, like muscles and ligaments, pose unique challenges due to their viscoelastic properties. Techniques to study these include:
- Rheology - examining the flow and deformation of matter.
- Stress-Strain Analysis - determining material deformation under stress.
Rheological studies in soft tissues are crucial for designing implants and surgical techniques that mimic natural behavior, improving effectiveness and biocompatibility.
Examples of Biomechanical Systems
Biomechanical systems are diverse and cater to a wide range of applications, harnessing mechanical principles to meet biological needs. Below are detailed examples showcasing the biomechanical systems that significantly impact engineering and healthcare.
Biomechanics of Cardiovascular System
The cardiovascular system serves as a prime example of biomechanical integration. It involves the study of how blood flow and vessel structure interact mechanically. Engineers aim to create models that replicate the behavior of blood within arteries and veins.Blood Flow Dynamics: The flow of blood can be modeled using the principles of fluid dynamics. A prevalent model is based on the Navier-Stokes equations to simulate blood flow velocity and pressure within vessels. For instance, the flow rate \( Q \) can be expressed as \[ Q = \frac{\text{Stroke Volume}}{\text{Cardiac Cycle Duration}} \].Vascular Resistance: It refers to the resistance faced by blood while flowing through the vessels. The formula is given by \[ R = \frac{\text{Change in Pressure}}{\text{Flow}} \]. This helps predict how changes in vessel diameter affect blood pressure.
An example of the application is in the development of stents, which are used to prevent artery blockage. These devices are designed to withstand hemodynamic forces and are often tested using models that simulate the pulsatile flow of blood.
In-depth computational models of the heart often employ advanced simulations that integrate electrical and mechanical behavior. This involves solving coupled partial differential equations (PDEs), representing the electrophysiological activity and mechanical contraction of heart tissues. Such an approach allows for examining arrhythmic events, potential therapeutic targets, and device interventions under varied physiological conditions.
Did you know that the mathematical modeling of ventricular dynamics can predict heart disease patterns and aid in surgical planning?
Biomechanical Principles in Engineering
Engineers apply biomechanical principles across many disciplines, ensuring systems are safe, efficient, and compatible with biological tissues. Here are some core principles:
- Mechanotransduction: The process by which cells convert mechanical stimulus into chemical activity. This principle is critical in designing scaffolds for tissue engineering.
- Load-Bearing Analysis: Used in the design of prosthetics and orthopedic devices, calculated using stress and strain formulas such as \[ \text{Stress } \sigma = \frac{\text{Force } F}{\text{Area } A} \].
- Energy Efficiency: In robotics, biomimetic systems are designed to minimize energy consumption while maximizing performance.
Mechanotransduction is a cellular process where mechanical stress is converted into biochemical signals, which influence cellular functions such as growth, differentiation, and apoptosis.
A biomimetic robot arm is an engineering marvel where these principles are put into practice. It mimics human arm movements using actuators and control systems that simulate muscle contractions and joint rotations. The torque calculation in each joint can be modeled using \[ \tau = I \cdot \alpha \], where \( \tau \) is torque, \( I \) is the rotational inertia, and \( \alpha \) is the angular acceleration.
Biomechanical engineering principles are increasingly used in designing consumer electronics, offering ergonomic solutions that prevent injury.
biomechanical systems - Key takeaways
- Biomechanical systems: Integration of biological and mechanical principles to solve problems and create innovations in fields like healthcare, sports, and robotics.
- Definition of biomechanical systems: An intersecting point of engineering, physics, and biology, using mechanical laws within biological contexts.
- Examples of biomechanical systems: Artificial limbs and orthotic devices, designed to mimic natural limb movements using mechanical and electronic principles.
- Understanding biomechanical systems: They involve complex mathematical modeling and physiological considerations to analyze parameters like movement, force, and torque.
- Biomechanics of cardiovascular system: Involves the study of blood flow and vessel mechanics, modeled with fluid dynamics and equations for blood flow dynamics and vascular resistance.
- Biomechanical principles in engineering: Include mechanotransduction, load-bearing analysis, and energy efficiency, crucial for designing prosthetics, orthopedic devices, and biomimetic robots.
Learn faster with the 12 flashcards about biomechanical systems
Sign up for free to gain access to all our flashcards.
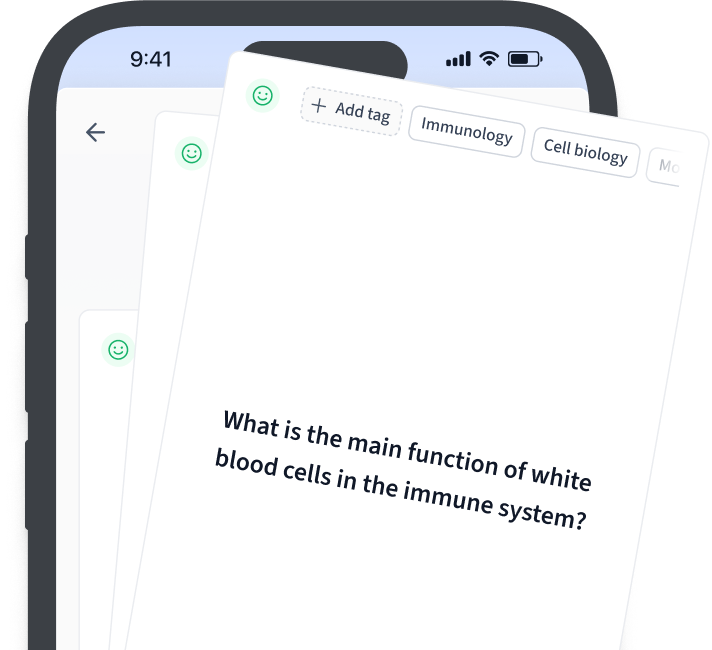
Frequently Asked Questions about biomechanical systems
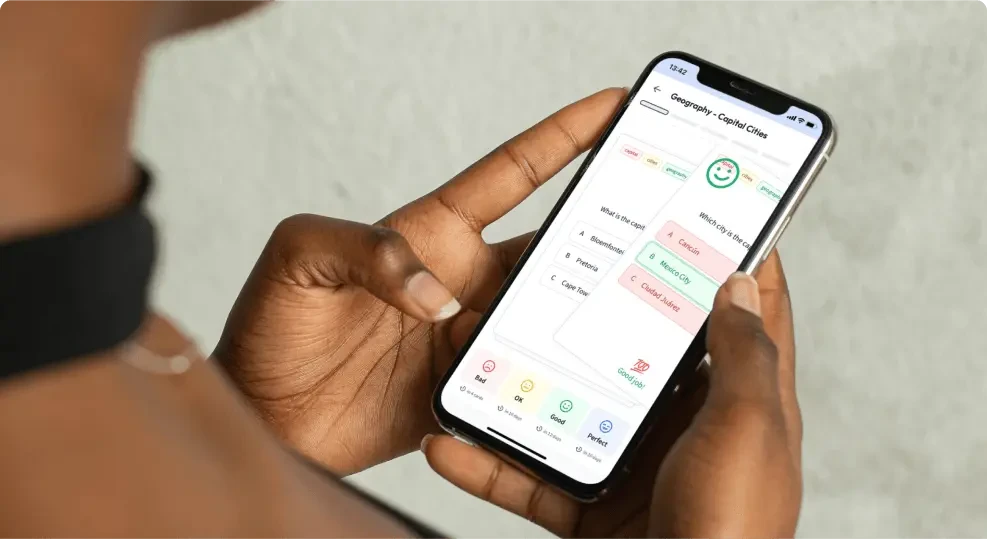
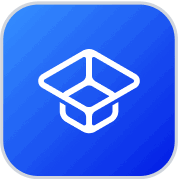
About StudySmarter
StudySmarter is a globally recognized educational technology company, offering a holistic learning platform designed for students of all ages and educational levels. Our platform provides learning support for a wide range of subjects, including STEM, Social Sciences, and Languages and also helps students to successfully master various tests and exams worldwide, such as GCSE, A Level, SAT, ACT, Abitur, and more. We offer an extensive library of learning materials, including interactive flashcards, comprehensive textbook solutions, and detailed explanations. The cutting-edge technology and tools we provide help students create their own learning materials. StudySmarter’s content is not only expert-verified but also regularly updated to ensure accuracy and relevance.
Learn more