Jump to a key chapter
Introduction to Biomechatronics
Biomechatronics is a fascinating field where biology, mechanics, and electronics converge to improve human capabilities. In this field, the integration is often aimed at enhancing the mobility or functionality of individuals with disabilities. Biomechatronics combines elements of robotics, bioengineering, and human physiology to create devices that can seamlessly blend with the human body.
Core Concepts of Biomechatronics
At the heart of biomechatronics are several core concepts that allow technology and biology to work synergistically. Some of these concepts include:
- Sensors: Devices that collect information from the biological system.
- Actuators: Motive components that cause movement or response.
- Controllers: Systems that interpret sensor inputs to provide adaptive responses.
- Interfaces: Connections that allow communication between machines and biological systems.
Biomechatronics is the interdisciplinary study that focuses on the creation of intelligent devices that directly interact with human anatomy and physiology.
Applications and Devices
Biomechatronics has numerous applications that enhance quality of life. Its key focus is often on assistive devices. Examples include:
- Prosthetic Limbs: These are devices that replace lost limbs and offer functionality, often powered by motors mimicking muscle movement.
- Cochlear Implants: These devices convert sound waves into electrical signals to assist individuals with hearing impairments.
- Exoskeletons: Wearable machines that augment the strength or mobility of the wearer.
One real-world application of biomechatronics is the development of a robotic hand. This device can be controlled using nerve signals from the person's arm, enabling movements such as grasping or holding. The sensors in the hand provide feedback to the user, making it feel more natural.
The Science Behind Biomechatronics
The scientific principles of biomechatronics are deeply rooted in physics and biology. Typically, devices are designed based on biomechanics, which involves analyzing human movements and forces. Engineers use equations such as: \[ F = ma \] Where \( F \) is force, \( m \) is mass, and \( a \) is acceleration, to predict the forces necessary for movement in biomechanics.
Dive deeper into the technology by understanding myoelectric control. Myoelectric signals are electrical signals that muscles create upon contraction. These signals can be employed in prosthetics control. By using these signals, devices can be controlled by muscular contractions that are still present in the residual limb. Myoelectric prosthetics use an algorithm to interpret these signals and convert them into mechanical motions.
Challenges and Future Directions
Biomechatronics is a field faced with challenges such as ethical considerations, technical limitations, and the need for extensive research. Moving forward, engineers are exploring:
- Improved Materials: Advances in materials that mimic human tissue for better integration and comfort.
- Advanced Sensors: Developing sensors capable of more accurate human-machine interaction.
- AI Integration: Using artificial intelligence to optimize the performance of assistive devices.
Remember that in biomechatronics, the ultimate goal is often to create systems that can be as close to natural human function as possible.
Biomechatronics Definition and Principles
Biomechatronics is an interdisciplinary field that integrates the study of biology, mechanics, and electronics to develop enhanced assistive technologies. This field utilizes principles of engineering to merge humans and machines, creating devices that enhance human abilities.
Understanding Biomechatronics
Biomechatronics involves several key components that enable the interaction between machines and biological systems. Some important elements include:
- Sensors: Devices used to collect data from the biological system, such as muscle activity.
- Actuators: Mechanic components that produce movement or provide support.
- Controllers: Systems that process sensor data and generate responses.
- Interfaces: Connections ensuring seamless communication between device and user.
Consider a robotic prosthetic limb. By integrating sensors in the arm, this device detects electrical signals from the user's muscles. Actuators then mimic muscle contractions, providing the wearer with the ability to perform tasks like picking up objects.
Mathematical Principles
The development of biomechatronic devices greatly relies on fundamental biomechanics. Engineers use mathematical models to predict how devices will interact with the human body. An essential consideration is the force exerted by prosthetics, calculated using Newton's second law: \[ F = ma \] Where \( F \) is the force applied, \( m \) is the mass of the prosthetic, and \( a \) is the acceleration. Understanding these dynamics enables the creation of efficient and responsive devices.
A deeper exploration into biomechatronics reveals the use of myoelectric control. Muscles emit electrical signals upon contraction, and these can be translated into control commands for prosthetic limbs. Algorithms interpret these signals to determine the desired motion. This innovative approach allows users to control prosthetic devices more naturally and intuitively.
Future Challenges and Advancements
Biomechatronics faces numerous challenges, such as ensuring the comfort and fit of devices, developing materials that mimic human tissue, and enhancing the user-device interface. Future advancements may include:
- Refining material technology for better integration with the body.
- Improving sensor accuracy and responsiveness.
- Integrating artificial intelligence for smarter, adaptive controls.
In future applications of biomechatronics, the goal is often to enhance human-machine integration to improve human capabilities and quality of life.
Biomechatronics Engineering
In the realm of engineering, biomechatronics stands out as a unique discipline, merging biology, mechanics, and electronics to enhance human functionality. The primary aim is to develop technical solutions that can improve physical capabilities and the quality of life for individuals who rely on assistive devices.
Components of Biomechatronic Systems
Biomechatronics systems are comprised of several integral components which interact seamlessly:
- Sensors: These components gather data from the body, such as temperature or muscle contractions.
- Actuators: These are responsible for producing physical movements.
- Controllers: The brains of the system, interpreting sensor data to facilitate movement.
- Interfaces: They allow for communication between human and machine elements to ensure harmony.
Biomechatronics is defined as the fusion of biological techniques and systems with mechanical and electronic engineering components to create devices that augment or enhance human capabilities.
An application of biomechatronics is in developing advanced prosthetic limbs. These limbs incorporate actuators that replicate muscle function and sensors for detecting movement intention, resulting in natural and intuitive use.
Mathematical Foundations
The success of a biomechatronic system often relies heavily on its mathematical foundations. For example, precise control of a prosthetic limb can be achieved using equations from mechanics, such as: \[ F = ma \] Where \( F \) denotes force, \( m \) represents mass, and \( a \) is acceleration. This equation helps determine the necessary force for prosthetic movements, ensuring effective performance.
A notable aspect of biomechatronics is the use of neural interfacing. This advanced technique involves capturing brain or nerve signals, which are then used to control biomechatronic devices. Innovations in neural interfacing hold the potential to revolutionize user-device interaction, leading to devices that respond instantaneously to thoughts, thereby creating a more natural user experience.
Overcoming Challenges in Biomechatronics
Despite its promising benefits, biomechatronics is not without challenges. Key areas in need of advancement include:
- Material Compatibility: Finding biocompatible materials that seamlessly integrate with the human body.
- Signal Processing: Developing more sophisticated algorithms to accurately interpret biological signals.
- Power Supply: Creating efficient energy sources for prolonged device operation.
For those exploring biomechatronics, always consider the ethical implications of enhancing human capabilities, as this field continually pushes the boundaries of what's possible.
Biomechatronics Applications and Examples
Biomechatronics stands as a pioneering field that merges biology and technology, presenting remarkable applications that improve human life. This section explores how biomechatronic systems are applied in real-world scenarios and offers examples that illustrate their utility.
Prosthetic Devices
Prosthetic limbs are a prime example of biomechatronic applications that restore mobility and function. These devices often incorporate:
- Sensors: Detect muscle signals to convert them into movements.
- Actuators: Replicate muscle dynamics to mimic natural limb behavior.
- Controllers: Coordinate input from sensors, optimizing the response.
A typical example involves a lower leg prosthesis powered by electric motors. It utilizes feedback from sensors to maintain balance and stability on different surfaces. This allows users to walk, run, and even climb stairs naturally.
Exoskeletons
Exoskeletons are wearable devices that enhance strength and endurance. Their biomechanics are structured to:
- Support muscle activity by bearing load.
- Aid mobility for individuals with disabilities or injuries.
- Assist in lifting heavy objects, reducing strain on the user.
A deeper look into exoskeletons reveals the integration of neural feedback systems. These systems can interpret brain signals to control limb movement, essentially allowing the wearer to move the machine as they would their own muscles. Such technology encompasses the use of myoelectric sensors that provide immediate response to neurological commands, making exoskeletons an extension of the body.
Assistive Hearing Devices
In the realm of auditory aids, biomechatronics has led to the creation of complex devices such as cochlear implants. These devices:
- Translate sound waves into electrical signals.
- Send signals directly to the auditory nerve.
- Facilitate clear sound perception for those with profound hearing loss.
For instance, a child born with hearing loss can perceive sounds and even develop speech through the consistent use of a cochlear implant. These devices empower users to engage more fully with the auditory world around them.
Understanding the broad applications of biomechatronics opens the door to limitless possibilities in enhancing human capabilities and improving quality of life.
biomechatronics - Key takeaways
- Biomechatronics Definition: The interdisciplinary study focusing on integrating biology, mechanics, and electronics to develop intelligent devices that interact directly with human anatomy and physiology.
- Biomechatronics Engineering: A field merging biological techniques with mechanical and electronic engineering to create devices that enhance human functionality.
- Core Concepts: Key elements include sensors, actuators, controllers, and interfaces to enable technology and biology to interact synergistically.
- Biomechatronics Applications: Includes prosthetic limbs, cochlear implants, and exoskeletons aimed at assisting individuals with disabilities.
- Biomechatronics Principles: Based on biomechanics, using mathematical models such as Newton's second law (F = ma) to predict device interaction with the human body.
- Biomechatronics Examples: Notable mention of a robotic hand controlled by nerve signals, showcasing integration and feedback for natural usage.
Learn faster with the 12 flashcards about biomechatronics
Sign up for free to gain access to all our flashcards.
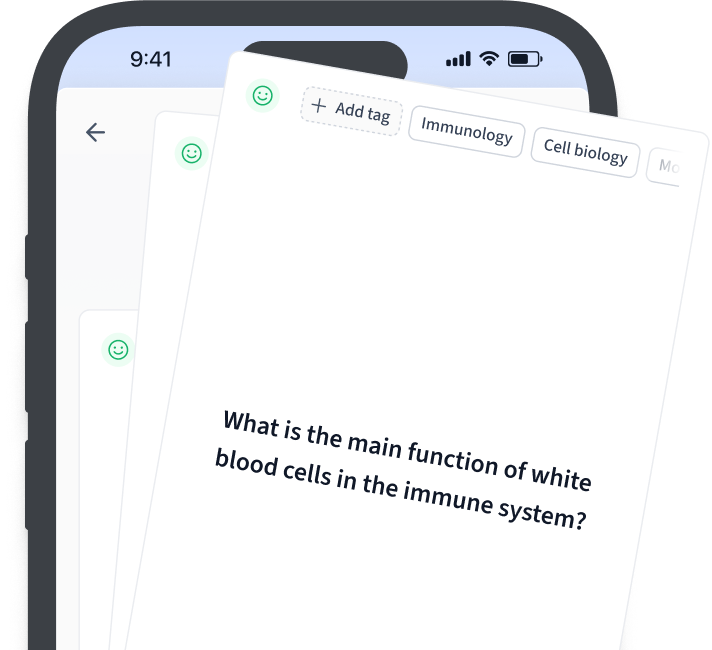
Frequently Asked Questions about biomechatronics
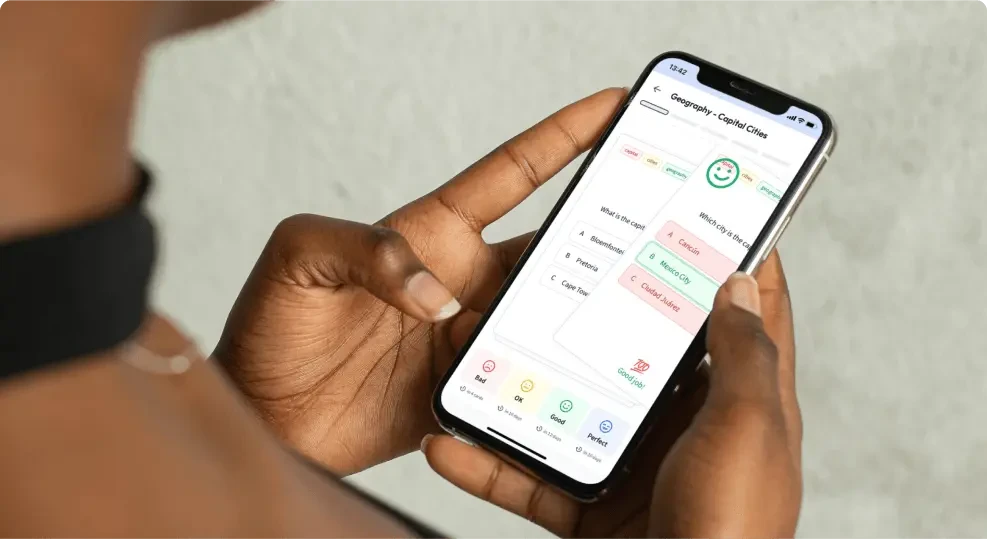
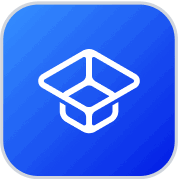
About StudySmarter
StudySmarter is a globally recognized educational technology company, offering a holistic learning platform designed for students of all ages and educational levels. Our platform provides learning support for a wide range of subjects, including STEM, Social Sciences, and Languages and also helps students to successfully master various tests and exams worldwide, such as GCSE, A Level, SAT, ACT, Abitur, and more. We offer an extensive library of learning materials, including interactive flashcards, comprehensive textbook solutions, and detailed explanations. The cutting-edge technology and tools we provide help students create their own learning materials. StudySmarter’s content is not only expert-verified but also regularly updated to ensure accuracy and relevance.
Learn more