Jump to a key chapter
Clinical Biomechanics Definition
Clinical biomechanics is a specialized branch of biomechanics, applied specifically to clinical settings, helping to assess and treat patients with mobility issues or injuries. It combines the principles of engineering and biological sciences to analyze human movement and musculoskeletal mechanics.
Clinical Biomechanics: The study of motion and forces in the human body using principles of mechanics to solve health-related problems.
Importance in Healthcare
In healthcare, clinical biomechanics plays a crucial role in diagnosing injuries and improving treatment strategies. By understanding how forces interact with the body, healthcare professionals can design better treatment plans. Here are some ways it is applied:
- Rehabilitation: Enhancing recovery for patients following injuries or surgeries.
- Orthopedics: Assisting in the design of prosthetics and orthotic devices.
- Sports Medicine: Improving athletic performance and preventing injuries.
For instance, clinical biomechanics can help determine the optimal angle for joint replacement by analyzing the forces in the joint, which is crucial for the success of surgical procedures.
A deeper understanding of clinical biomechanics allows for the innovation of various medical devices and contributes significantly to the design process. For example, when engineering an artificial limb, one must consider not just the limb's mechanics, but also how the limb will interact with the human body as a whole system. Equations modeling this interaction often utilize principles from statics and dynamics. Considering dynamic balance for a leg prosthesis: \( F_{ground} + F_{muscle} = ma_{center-of-mass} \) where \( F_{ground} \) is the ground reaction force, \( F_{muscle} \) is the muscle force, and \( ma_{center-of-mass} \) is the mass times the acceleration of the body's center of mass. This formula assists in ensuring the prosthetic effectively supports and propels the user.
Technological Advancements
Advancements in technology have significantly enhanced the scope of clinical biomechanics. Modern imaging techniques like MRI and CT scans provide detailed insights into joint mechanics and soft tissue injuries, assisting in creating more precise biomechanical models. High-speed cameras and motion capture systems further allow for detailed movement analysis, identifying areas of stress and inefficiency in a patient's gait or movement pattern. These systems can be seamlessly integrated with software to simulate and modify treatment protocols.
The integration of AI in clinical biomechanics is a trending advancement, offering predictive analyses for patient movement patterns.
Clinical Biomechanics Applications in Engineering
Clinical biomechanics integrates engineering principles into healthcare to diagnose, treat, and improve human movement. Its applications are vast and impact various areas within the engineering discipline, providing innovative solutions to complex health issues.
Orthopedic Engineering
Orthopedic engineering greatly benefits from clinical biomechanics. By using mechanical principles, it aids in designing joint replacements, braces, and prosthetic limbs that mimic natural movement. Key concepts include:
- Joint Mechanics: Understanding forces acting in joints to improve artificial joint design.
- Load Distribution: Ensuring weight is evenly distributed through prosthetics to minimize discomfort.
In knee replacement surgery, clinical biomechanics examines the knee's motion to create a prosthetic that matches the patient's natural movement, using simulations to adjust the implant's design.
Further, in spinal biomechanics, understanding the forces during various movements helps in developing support devices for patients with back problems. Considering a patient bending over, the stress in the lumbar region can be analyzed using:\[ \tau = r \times F \times \text{sin}(\theta)\]where \( \tau \) is the torque, \( r \) is the radius or distance from the axis, and \( \theta \) is the angle of incidence.This approach aids in designing safer, more effective spinal implants.
Rehabilitation Devices
In rehabilitation engineering, devices like exoskeletons and ergometers are designed using clinical biomechanics principles. These devices aid patients in regaining muscle strength and coordination.
Modern rehabilitation devices often include sensors, providing real-time feedback and adjustments to improve patient outcomes.
Sports Biomechanics
Applying clinical biomechanics in sports engineering helps both injury prevention and performance enhancement. Engineers analyze athletes' movements and optimize gear, like shoes and clothing, for better aerodynamics and minimal injury risks. Analyzing sprinters reveals how force impact on the ground relates to velocity: \[ F_{net} = m \times a \]where \( F_{net} \) is the net force, \( m \) is the mass, and \( a \) is the acceleration.
Sports Biomechanics: The application of biomechanical principles to improve athletic performance and reduce injury.
Clinical Biomechanics of the Lower Extremities
Clinical biomechanics is pivotal for understanding the mechanics of the lower extremities, aiding in the diagnosis and treatment of various musculoskeletal disorders. The lower extremities, which include the hips, knees, ankles, and feet, play a crucial role in daily activities such as walking and running. By examining the forces, moments, and mechanical energy within these bodily segments, clinical biomechanics helps clinicians and engineers to devise solutions that enhance mobility and reduce pain for individuals with lower extremity issues.
Techniques for Analyzing Lower Extremities
There are various techniques applied in clinical biomechanics to analyze the lower extremities, each offering detailed insights into movement patterns and providing data critical for developing therapeutic interventions:
- Motion Capture Systems: Employ cameras and sensors to track movement accurately, producing quantitative data on gait and posture.
- Force Plates: Measure ground reaction forces when walking or running, helping determine load distribution over time.
Advanced imaging techniques, such as MRI and CT scans, provide a layered perspective on joint and soft tissue conditions, contributing to clinical assessments and interventions. Consideration of muscle forces and joint moments can be modeled as: \[ \sum M = I \times \alpha \] where \( \sum M \) is the sum of moments, \( I \) is the moment of inertia, and \( \alpha \) is the angular acceleration, providing insights into rotational kinematics of joints.
As an example, when analyzing the gait cycle using motion capture, joints like the knee are closely examined to identify any abnormal patterns. The analysis uses the equation: \[ \theta = \theta_0 + \omega_0 t + \frac{1}{2} \alpha t^2 \] where \( \theta \) is the angular displacement. This identifies discrepancies that may lead to corrective procedures or orthotic recommendations.
Integrating wearable technology with traditional analytical techniques provides continuous real-time monitoring of limb movements, facilitating personalized treatment plans.
Clinical Biomechanics of the Spine
The study of clinical biomechanics of the spine provides essential insights into spinal function and dysfunction, crucial for the diagnosis and treatment of spinal disorders. It involves understanding the mechanical behavior of the spine, from the vertebrae and joints to the intervertebral discs and ligaments. This field applies mechanical principles to develop better surgical interventions and rehabilitation strategies.
Techniques for Clinical Biomechanics of the Spine
Several techniques are employed to assess and analyze spinal biomechanics effectively. These methods combine technological advancements with biomechanical principles to provide comprehensive evaluations. Below are some widely used techniques:
- Imaging Techniques: MRI and CT scans provide detailed views of spinal structures and help identify pathological conditions.
- Finite Element Analysis (FEA): A computational method used to simulate spinal biomechanics under various conditions, predicting stress distribution across the spine.
- Electromyography (EMG): Measures electrical activity of muscles around the spine to assess muscular response and coordination.
An example of FEA usage in spinal biomechanics involves simulating the stress distribution on an intervertebral disc during various movements, which can be modeled using equations like:\[\sigma = \frac{F}{A}\]where \(\sigma\) is the stress, \(F\) is the force, and \(A\) is the area over which the force is distributed.
Further exploration into spinal biomechanics includes understanding spinal load tolerance. Misalignments or excess stress can lead to degenerative diseases. Using models, researchers can predict how weight or movement affects vertebrae over time. Considering a section of the spine, the bending moment can be expressed as:\[M = E \cdot I \cdot \kappa\]where \(M\) is the moment, \(E\) is Young's modulus, \(I\) is the moment of inertia, and \(\kappa\) is the curvature, providing insights into potential failure points under mechanical loads.
Understanding individual spinal biomechanics is essential for designing personalized back support devices or surgical strategies aimed at enhancing spinal health and comfort.
Biomechanical Engineering Explained
Biomechanical engineering combines principles from biology and engineering to create tools that improve health and well-being. By analyzing how biological structures can be applied in designing medical devices, this field enhances our understanding of complex biological systems. Within biomechanical engineering, various disciplines converge to optimize the development of prosthetics, orthopedic implants, and diagnostic tools that mimic natural body forms. This approach aims to enhance both functionality and comfort in medical devices.
Core Principles of Biomechanical Engineering
The core principles of biomechanical engineering involve an interplay between mechanics and biological functions.
- Mechanics: Principles of motion and forces are crucial for analyzing body movements.
- Material Science: Selecting materials that are biocompatible and durable.
- Systems Integration: Merging mechanical systems with biological ones for optimal functionality.
Biomechanical Engineering: The field that applies principles of mechanics to biological systems to solve problems related to human health.
In developing a new prosthetic limb, biomechanical engineers may use computational models to predict how forces distribute across the device. This involves analyzing the stress-strain relationship in materials to ensure the limb withstands daily activities. Consider the following stress equation:\[\sigma = \frac{F}{A}\]where \(\sigma\) is the stress experienced by a material, \(F\) is the force applied, and \(A\) is the cross-sectional area. This helps in selecting materials that prevent failure under load.
For instance, in designing an artificial knee, engineers use dynamic simulations to study the knee's range of motion under various loads. This process may involve solving equations of motion like:\[\theta = \theta_0 + \omega_0 t + \frac{1}{2} \alpha t^2\]where \(\theta\) represents angular displacement, helping ensure the prosthetic replicates natural knee movement effectively.
Computational modeling often involves using software that can predict device performance under physiological conditions, leading to more reliable designs before physical prototypes are tested.
clinical biomechanics - Key takeaways
- Clinical biomechanics definition: A specialized branch of biomechanics applied in clinical settings to assess and treat mobility issues using engineering and biological sciences.
- Clinical biomechanics applications in engineering: Integrates engineering principles into healthcare, impacting areas like prosthetic design and joint mechanics analysis.
- Clinical biomechanics of the lower extremities: Focuses on understanding the mechanics of the hips, knees, ankles, and feet to improve mobility and reduce pain.
- Clinical biomechanics of the spine: Involves studying the mechanics of the spine for the diagnosis and treatment of spinal disorders using techniques like MRI and finite element analysis.
- Clinical biomechanics techniques: Includes methods like motion capture, force plates, MRI, and electromyography to analyze human movement and forces.
- Biomechanical engineering explained: Combines biology and engineering principles to create medical devices that improve health, emphasizing mechanics, material science, and systems integration.
Learn faster with the 10 flashcards about clinical biomechanics
Sign up for free to gain access to all our flashcards.
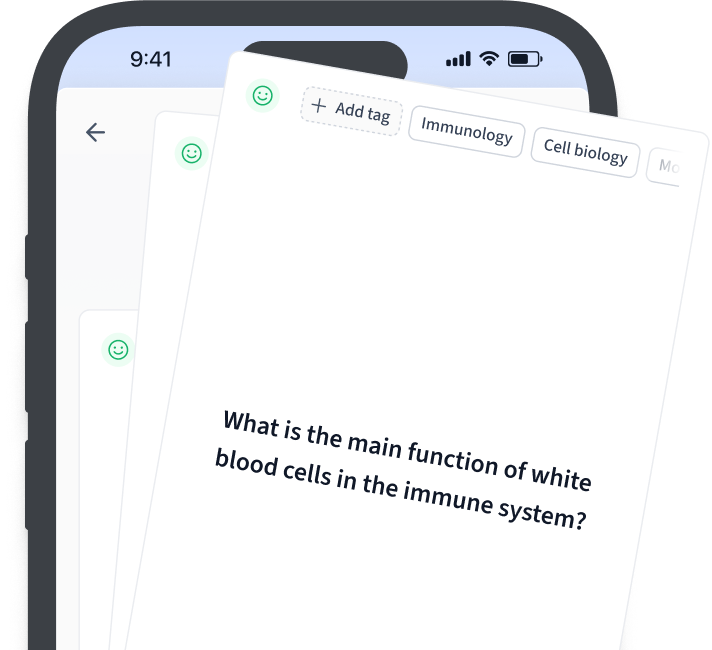
Frequently Asked Questions about clinical biomechanics
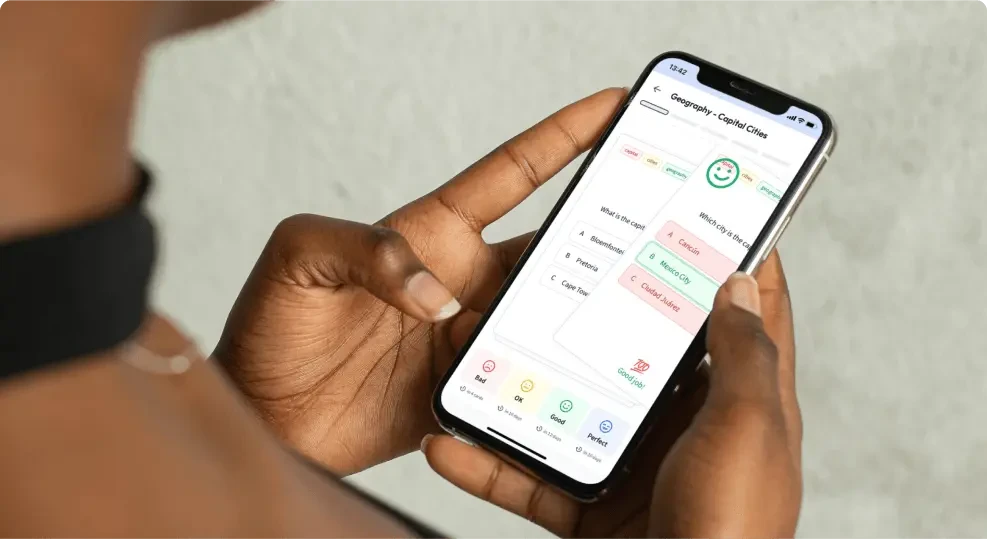
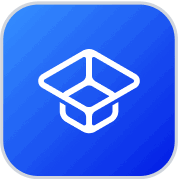
About StudySmarter
StudySmarter is a globally recognized educational technology company, offering a holistic learning platform designed for students of all ages and educational levels. Our platform provides learning support for a wide range of subjects, including STEM, Social Sciences, and Languages and also helps students to successfully master various tests and exams worldwide, such as GCSE, A Level, SAT, ACT, Abitur, and more. We offer an extensive library of learning materials, including interactive flashcards, comprehensive textbook solutions, and detailed explanations. The cutting-edge technology and tools we provide help students create their own learning materials. StudySmarter’s content is not only expert-verified but also regularly updated to ensure accuracy and relevance.
Learn more