Jump to a key chapter
Definition of Condensation Heat Transfer
Condensation Heat Transfer is a process where vapor transforms into liquid while releasing heat energy to the surroundings. This process is important in many engineering systems and industries, particularly in cooling and heating mechanisms. Understanding the fundamental concepts of condensation helps in improving the efficiency of thermal systems.
Importance in Mechanical Engineering
Condensation heat transfer plays a crucial role in the field of mechanical engineering. It affects the efficiency and design of various mechanical systems such as:
- Heat exchangers
- Power generation systems
- Refrigeration cycles
- Air conditioning systems
Consider a steam power plant where steam exits a turbine and enters a condenser. Here, the steam is cooled to water by transferring its latent heat of vaporization to the cooling water. The latent heat energy released can be calculated using the formula:\[ Q = m \times h_{fg} \]where \( Q \) is the heat released, \( m \) is the mass of the steam, and \( h_{fg} \) is the latent heat of vaporization.
In the field of HVAC (Heating, Ventilation, and Air Conditioning), managing condensation effectively can significantly reduce energy costs.
Basic Principles
The core understanding of condensation involves grasping some underlying principles. A comprehensive look at these principles will help in understanding the overall mechanics:1. Saturation Temperature: This is the temperature at which vapor begins to condense. To initiate condensation, the surface temperature must be below the saturation temperature.2. Heat Transfer: The process involves significant heat transfer, commonly modeled by the formula:\[ Q = UA(T_{sat} - T_s) \]where \( Q \) is the heat transfer rate, \( U \) is the overall heat transfer coefficient, \( A \) is the area, \( T_{sat} \) is the saturation temperature, and \( T_s \) is the surface temperature.3. Film and Dropwise Condensation:
- Film Condensation: Characterized by a continuous film of liquid forming over the surface, which acts as a thermal barrier. Most engineering systems utilize film condensation.
- Dropwise Condensation: In this type, discrete droplets form on the surface, allowing more efficient heat transfer but harder to maintain uniformly.
Understanding the complexities of condensation heat transfer can give insights into its application in advanced systems like desalination plants where both heat and mass transfer occur. Desalination through condensation requires controlling the saturation pressure and temperature meticulously to optimize the recovery of water from vapor. The balance of energy equations and the manipulation of surface properties are critical to developing efficient condensers in such systems.
Condensation Heat Transfer Coefficient
Understanding the Condensation Heat Transfer Coefficient is essential for evaluating the performance of systems where heat exchange through condensation is critical. This coefficient reflects how efficiently heat is transferred during the condensation process. It is influenced by various factors and measuring techniques that define the design and efficiency of condensers.
Factors Affecting Coefficient Value
Several factors can affect the magnitude of the condensation heat transfer coefficient:
- Surface Type: Smooth, rough, or enhanced surfaces can alter the heat transfer rate by changing the mode of condensation from dropwise to filmwise.
- Temperature Difference: The larger the temperature difference between the vapor and the condensing surface, the higher the driving force for heat transfer.
- Vapor Properties: The properties of the vapor such as viscosity, thermal conductivity, and latent heat can significantly influence the efficiency of heat transfer.
- Gravity and Orientation: The orientation of the condensing surface (e.g., vertical, horizontal) can affect the thickness and behavior of the condensate film.
Using hydrophilic or hydrophobic coatings can manipulate surface characteristics to enhance condensation efficiency.
Let's calculate the heat transfer rate using given parameters:The formula for the heat transfer coefficient \( h \) can be expressed as:\[ h = \frac{Q}{A(\Delta T)L} \]Consider a surface with a heat transfer area \( A = 10 \) square meters, a temperature difference \( \Delta T = 20 \) K, and a transfer rate \( Q = 2000 \) Watts. The length of condensation \( L \) is 5 meters:\[ h = \frac{2000}{10 \times 20 \times 5} = 2 \: W/m^2K \]
Advancements in materials science have led to the development of specialized coatings that can dramatically alter the hydrodynamic behavior of condensate films. Coatings that promote dropwise condensation over filmwise can increase the heat transfer coefficient up to 10 times. Furthermore, nanostructured surfaces are being researched for potential breakthroughs in modifying surface energy and contact angles, which can lead to enhanced thermal performance in various industrial applications.
Measuring Techniques
Accurately measuring the condensation heat transfer coefficient involves several sophisticated techniques:
- Calorimetric Method: Involves measuring the heat absorbed by cooling water and the corresponding temperature differences within the system to determine the heat transfer rate.
- Thermocouple Probes: Small thermocouples are placed at various points on the condensate surface to gather temperature data, from which the coefficient can be calculated.
- Infrared Thermography: This non-contact method uses infrared cameras to visualize and calculate surface temperatures and thus find the heat transfer coefficient.
- Computational Fluid Dynamics (CFD): A numerical approach that simulates the conditions of condensation, helping to estimate heat transfer coefficients under controlled virtual environments.
Film Condensation Heat Transfer
In many industrial applications, understanding film condensation heat transfer is vital for designing efficient thermal systems. This process occurs when a vapor in contact with a cooler surface condenses into a liquid film. The film flows along the surface due to gravity, and how efficiently it transfers heat depends on various factors.
Characteristics and Applications
Film condensation is characterized by several key traits:
- Film Formation: A continuous liquid film forms on the condensing surface, acting as an insulating layer, which affects the heat transfer rate.
- Gravity Influence: The movement and thickness of the film are heavily influenced by gravity and the angle of the surface.
- Surface Temperature: The temperature of the surface must be below the saturation temperature of the vapor for condensation to occur.
- Thermal Conductivity: The thermal properties of the vapor and the liquid here determine how quickly heat is able to flow across the film.
- Steam Condensers: In thermal power plants, steam condensers convert exhausted steam from turbines to water for reuse.
- Desalination: Facilitates freshwater production by condensing steam in a controlled environment.
- Refrigeration: Essential in evaporator coils where the refrigerant condenses on coils during the refrigeration cycle.
A fascinating area of study is enhanced film condensation through surface treatments. Researchers explore surfaces with microstructures or nano-coatings to reduce the thermal resistance of the liquid film. These surfaces can change how droplets form and coalesce, possibly enhancing dropwise condensation and improving efficiency by several folds compared to traditional methods.
The transition from film to dropwise condensation on surfaces with special coatings can greatly increase the efficiency of heat exchangers.
Mathematical Models
Mathematical modeling of film condensation helps predict and optimize heat transfer rates in various systems. Here are key models and equations used:
- Nusselt's Film Condensation Model: Provides a foundational approach to quantify heat transfer in film condensation. It assumes laminar flow of the film and constant properties.
- Heat Transfer Coefficient: Calculated using:\[ h = \frac{k}{D} \left( \frac{4g \rho^2 h_{fg} \Delta T}{\mu_l} \right)^{1/3} \]
- Reynolds Number: Indicates whether the flow is laminar or turbulent:\[ Re = \frac{\rho_l u L}{\mu_l} \]
- \( h \) is the heat transfer coefficient
- \( k \) is the thermal conductivity of the film
- \( D \) is the diameter of the condenser tube
- \( g \) is the acceleration due to gravity
- \( \rho \) is the density of the liquid
- \( h_{fg} \) is the latent heat of vaporization
- \( \Delta T \) is the temperature difference between the vapor and surface
- \( \mu_l \) is the dynamic viscosity of the liquid
- \( L \) is the characteristic length
- \( u \) is the velocity of the liquid film flow
Example of calculating heat transfer using the Nusselt film condensation relation:Assume a vertical condenser tube with a diameter \( D = 0.05 \) m, submerged in steam at an average temperature difference \( \Delta T = 25 \) K. Given values: \( k = 0.6 W/m.K \), \( g = 9.81 m/s^2 \), \( \rho = 1000 kg/m^3 \), \( h_{fg} = 2260 kJ/kg \), \( \mu_l = 0.001 Pa.s \). Plugging these into the Nusselt equation:\[ h = \frac{0.6}{0.05} \left( \frac{4 \times 9.81 \times (1000)^2 \times 2260000 \times 25}{0.001} \right)^{1/3} \]Solving gives the heat transfer coefficient for this scenario.
Boiling and Condensation Heat Transfer
Understanding both boiling and condensation heat transfer is crucial for designing effective thermal systems. While boiling involves liquid turning into vapor, condensation is the reverse process where vapor transitions into liquid, releasing heat.
Comparison between Boiling and Condensation
Boiling and condensation are two opposite heat transfer processes but share some similarities. Here's how they compare:
Boiling | Condensation |
Vapor forms from liquid at its latent heat. | Liquid forms from vapor, releasing latent heat. |
Occurs in heating processes. | Occurs in cooling processes. |
Influenced by surface tension and external pressure. | Influenced by surface and temperature difference. |
Commonly used in boilers and evaporators. | Used in condensers and refrigerants. |
In both processes, the efficiency can be significantly enhanced by using surfaces with high thermal conductivities or special coatings.
Boiling and condensation interact in complex systems such as heat pumps. In these, both processes are linked in a cyclic operation. In certain heat pump applications, optimizing the boiling process can enhance condensation efficiency and vice versa. Surface enhancements, like microfin designs, aim to increase the surface area for boiling or dropwise condensation, enhancing heat transfer rates vastly. Detailed understanding of these interactions helps in designing sustainable and energy-efficient thermal systems.
Real-World Examples of Condensation Heat Transfer
Condensation heat transfer is crucial in numerous real-world applications. Here's how it's applied:
- Steam Power Plants: In these, cooling towers use condensation to recycle steam into water, ready for reheating and continuous energy production.
- Air Conditioning Systems: Condensation is used to remove moisture and heat from air, providing a cooling effect.
- Refrigeration: Heat is expelled from inside refrigeration units via condensation on external coils.
Consider an air conditioning system where the warm indoor air contacts a cool evaporator coil, causing moisture to condense. This phase change releases latent heat, captured and expelled by the system to cool air further. The efficiency is heavily reliant on the maintenance of the coil surface, ensuring effective condensation without scaling or dust accumulation.
Condensing Steam Heat Transfer Coefficient
The Condensing Steam Heat Transfer Coefficient is a key parameter in optimizing thermal systems, especially where steam is the working fluid. It measures how effectively heat is exchanged during the condensation of steam, impacting energy efficiency in systems such as power plants, refineries, and HVAC (Heating, Ventilation, and Air Conditioning) systems.
Role in Industrial Systems
In industrial systems, the role of the condensing steam heat transfer coefficient is significant:
- Condensers in Power Plants: These utilize steam's latent heat release to convert it back to water, facilitating a continuous operational cycle.
- Refineries: Crude oil heaters and distillation columns use condensing steam to transfer heat and help separate various hydrocarbons.
- HVAC Systems: Utilize condensing heat exchangers to recycle thermal energy, enhancing efficiency.
Example Calculation of Thermal Efficiency:Consider a condenser in a power plant operating at a steam temperature of 373 K and a cooling water temperature of 293 K. Calculate the thermal efficiency using:\[ \text{Thermal Efficiency} = \left(1 - \frac{T_{cool}}{T_{steam}}\right) \times 100 \]%where \(T_{cool}\) is the cooling water temperature and \(T_{steam}\) is the steam temperature:\[ \text{Thermal Efficiency} = \left(1 - \frac{293}{373}\right) \times 100 \approx 21.5\]%
condensation heat transfer - Key takeaways
- Condensation Heat Transfer: Process where vapor transforms into liquid, releasing heat energy.
- Condensation Heat Transfer Coefficient: Reflects efficiency of heat transfer during condensation, influenced by surface type, temperature difference, vapor properties, and more.
- Film Condensation Heat Transfer: Occurs when vapor condenses into a liquid film on a cooler surface, critical in thermal systems like power generation and refrigeration.
- Example of Condensation Heat Transfer: Steam condensing in power plant condensers to recycle water back into the boiler.
- Boiling and Condensation Heat Transfer: Opposite processes; boiling converts liquid to vapor, condensation converts vapor to liquid while releasing heat.
- Condensing Steam Heat Transfer Coefficient: Measures efficiency of heat exchange during steam condensation, vital for optimizing thermal systems like HVAC and power plants.
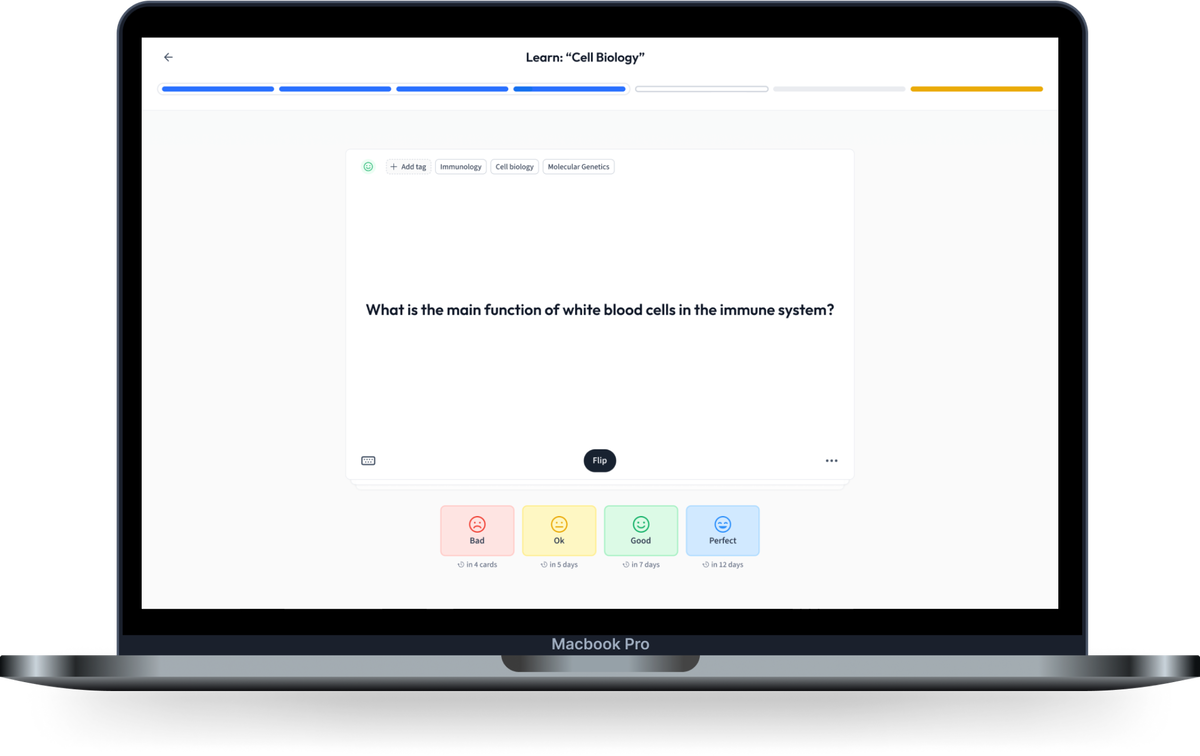
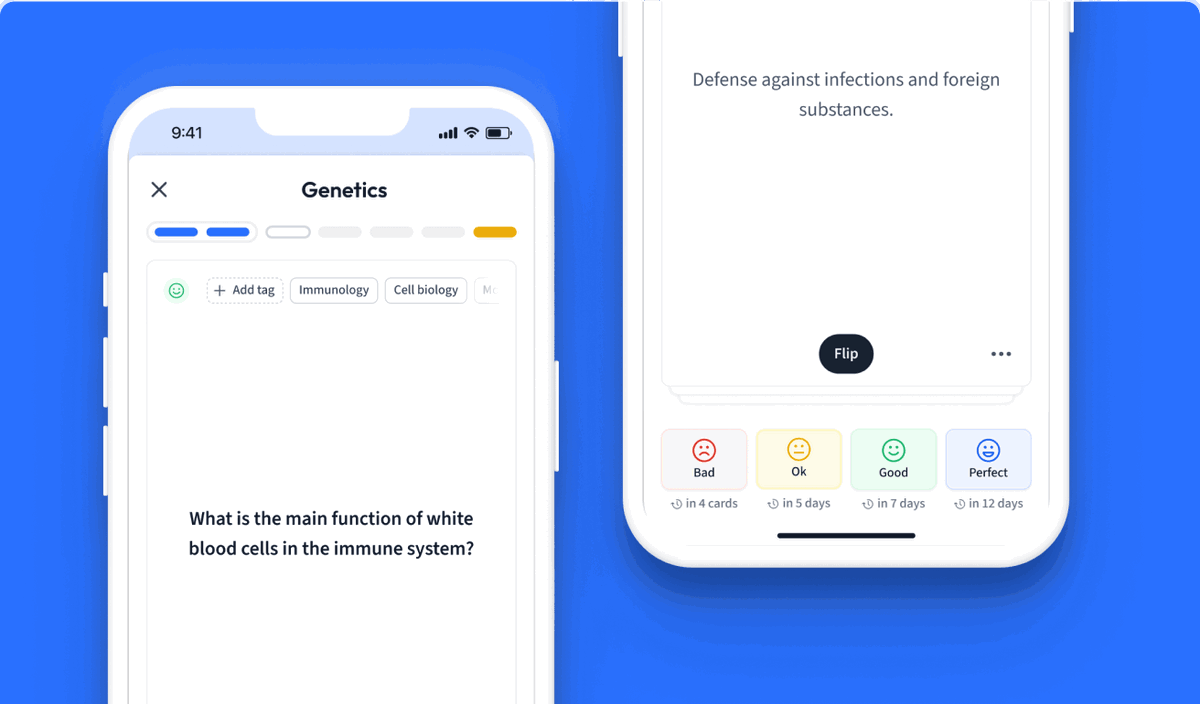
Learn with 10 condensation heat transfer flashcards in the free StudySmarter app
Already have an account? Log in
Frequently Asked Questions about condensation heat transfer
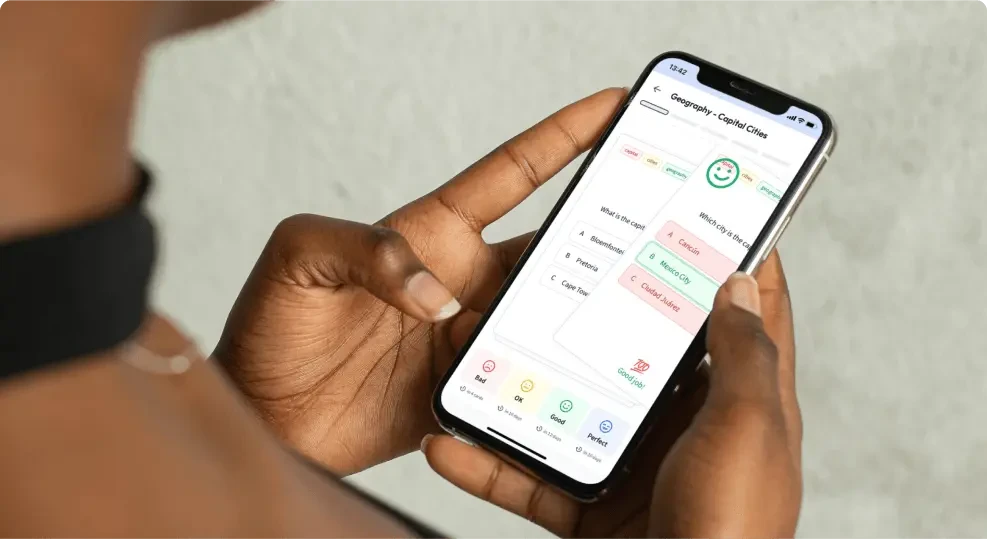
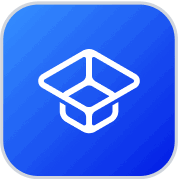
About StudySmarter
StudySmarter is a globally recognized educational technology company, offering a holistic learning platform designed for students of all ages and educational levels. Our platform provides learning support for a wide range of subjects, including STEM, Social Sciences, and Languages and also helps students to successfully master various tests and exams worldwide, such as GCSE, A Level, SAT, ACT, Abitur, and more. We offer an extensive library of learning materials, including interactive flashcards, comprehensive textbook solutions, and detailed explanations. The cutting-edge technology and tools we provide help students create their own learning materials. StudySmarter’s content is not only expert-verified but also regularly updated to ensure accuracy and relevance.
Learn more