Jump to a key chapter
Electromechanical Systems Definition
Electromechanical systems combine electrical and mechanical processes to perform various tasks. These systems use electrical control mechanisms to command and control mechanical operations. In essence, such systems involve the interaction of electrical and mechanical components to form an integrated unit.
Electromechanical Systems: An integrated assembly of electrical and mechanical elements that work together to perform designated tasks and operations.
Principles of Electromechanical Systems
To understand electromechanical systems, it's essential to familiarize yourself with their core principles:
- Electromagnetism: The foundation of most electromechanical systems, where electrical energy is converted into mechanical energy using magnetic fields.
- Control systems: These regulate the performance and operation of electromechanical devices, ensuring precise functioning.
- Energy conversion: This process typically involves the conversion of electrical energy into mechanical energy or vice versa.
Example: A simple example of an electromechanical system is an electric motor. In this device, electrical energy is converted into mechanical energy, producing rotational movement used in numerous applications like fans, pumps, and appliances.
Various components in electromechanical systems include:
Motors | Convert electrical energy to mechanical energy. |
Generators | Convert mechanical energy back into electrical energy. |
Sensors | Provide data for control systems to adjust operations. |
Did you know? Electromechanical systems are the backbone of modern robotics, enabling complex machines to move and react to their environment.
Mathematical Models in Electromechanical Systems
Mathematics plays a critical role in understanding and predicting the behavior of electromechanical systems. You may encounter models that involve:
- System Dynamics: Governing equations that describe the motions in the systems, usually represented by differential equations.
- Electrical Equations: Modeling the electrical part, often using Kirchhoff's laws and Ohm's law.
- Mechanical Equations: Involving forces and torques in mechanical components.
In their operation, electromechanical devices often require a feedback loop system. Consider the following:Feedback Control: These systems use feedback to adjust their operation continually. A common type of control is Proportional-Integral-Derivative (PID) control which adjusts the control input based on proportional, integral, and derivative information of error. This kind of control system is given by the equation:\[u(t) = K_p \cdot e(t) + K_i \int_{0}^{t} e(\tau) d\tau + K_d \frac{de(t)}{dt}\]where \(u(t)\) is the control input at time \(t\), \(K_p\), \(K_i\), and \(K_d\) are the proportional, integral, and derivative gains, and \(e(t)\) is the error signal between a desired setpoint and a measured process variable. This system enables a real-time response, ensuring accurate and efficient operation of electromechanical systems.
Explanation of Electromechanical Systems
Electromechanical systems are integral to modern life, combining mechanical engineering with electrical systems. These systems are used in everything from household appliances to robotic automation. They work through the integration of electrical and mechanical components; thus understanding them is vital for any aspiring engineer.
Electromechanical Systems: Systems that integrate electrical and mechanical processes and components to perform tasks, characterized by energy conversion between electrical and mechanical forms.
Key Components of Electromechanical Systems
Electromechanical systems consist of several core components, each playing an essential role in their operation:
- Motors and Actuators: Convert electrical energy into mechanical motion essential for device operation.
- Control Units: These regulate the system's operation, ensuring it performs tasks accurately and efficiently.
- Power Supply: Provides the necessary electrical energy for the system's operation.
- Sensors: Collect data from the environment to adjust system performance dynamically.
Example: Consider a robotic arm used in manufacturing. The arm's movement is controlled by electromechanical systems, with motors producing the required motion and sensors feeding back the arm's exact position to the control system.
In complex systems, keeping track of the components might be challenging. Typically, each part has a specific function and interaction:
Function | Component |
Energy Conversion | Motors, Generators |
Regulation | Control Systems |
Data Collection | Sensors |
Power Supply | Batteries, Transformers |
Understanding Electromechanical Equations
Analyzing these systems involves mathematical models that can describe behavior under various conditions. Let us see some examples of fundamental equations used:
- Electrical Dynamics: Represented using Kirchhoff's laws for circuits, such as \[ V = IR \] for Ohm's law.
- Mechanical Dynamics: Newton’s second law establishes the basic relationship for motion as \[ F = ma \] where \( F \) is the force, \( m \) is mass, and \( a \) is acceleration.
- Energy Conversion Efficiency: Efficiency can be described by ratios such as \[ \eta = \frac{P_{out}}{P_{in}} \]
Interested in robotics? Electromechanical systems form the backbone of robotic design and engineering.
Design of Electromechanical Systems
Designing electromechanical systems involves integrating mechanical elements and electrical circuits to create efficient solutions for tasks like automation, machinery control, and more. Understanding the underlying principles and influential factors is key to successful design.
Principles of Design
When designing electromechanical systems, you should consider several core principles. These principles ensure that the design is both effective and practical for its intended purpose.
- System Integration: The seamless combination of electrical and mechanical components to form a cohesive unit.
- Energy Efficiency: Optimizing the design to minimize energy consumption while maintaining performance through equations like \[ \text{Efficiency} = \frac{P_{out}}{P_{in}} \]
- Modularity: Designing with interchangeable components to facilitate maintenance and upgrades.
- Reliability: Ensuring the system performs consistently under various conditions through robust design and testing methodologies.
Always consider the environmental impact of your design, focusing on sustainability when possible.
Example: A drone serves as an excellent example of electromechanical system design. Key design considerations include motor selection for lift, battery efficiency for extended flight time, and control systems for navigation.
Factors Influencing Design
Several factors affect the design of electromechanical systems. Recognizing these factors will enable you to make informed decisions about your design objectives.
- Environmental Conditions: Temperature, humidity, and pressure can affect system components and overall performance.
- Cost Constraints: Budget considerations may limit material choices or component specifications.
- Space Limitations: The physical size of components can influence design in applications where space is constrained.
- User Requirements: The end-use of the system often dictates the necessary features and functionalities.
Balancing Act: Design is often a compromise between conflicting requirements. For example, cost considerations may necessitate selecting less expensive materials, which could impact performance or durability. These trade-offs require careful analysis to achieve a balanced solution. Models like the weighted decision matrix can aid in this process, quantifying the trade-offs by scoring different criteria such as cost, performance, etc. The formula is given by: \[ S = \left( \sum_{i=1}^{n} w_i \cdot v_i \right) \] where \( S \) is the score, \( w_i \) are the weights of the criteria, and \( v_i \) are the values of the criteria for given design options.
Tools for Designing Electromechanical Systems
Numerous tools and software facilitate the design and simulation of electromechanical systems. These tools help verify designs before physical prototyping, saving both time and cost.
- CAD Software: Computer-Aided Design (CAD) tools like SolidWorks and AutoCAD for creating detailed model designs.
- Circuit Simulation Software: Tools such as SPICE or LTspice allow testing and validating electrical circuit designs.
- Mathematics Software: MATLAB offers comprehensive mathematical modeling for analyzing system dynamics.
- Finite Element Analysis: ANSYS and similar tools model and simulate mechanical stress and strain on components.
Finite Element Analysis (FEA): A computational method for predicting how structures react to environmental conditions, such as force, heat, and vibration by breaking down a large system into smaller, simpler parts (elements).
Electromechanical Systems Examples
Electromechanical systems play a crucial role in both everyday life and industrial setups. They provide essential functionalities through the integration of mechanical elements with electronic control mechanisms, leading to efficient and complex operations.
Everyday Examples
In daily life, you encounter several electromechanical systems. These systems demonstrate the synergy between electrical circuits and mechanical movements. Here are some common examples:
- Electrical Fans: Utilize electric motors to convert electrical energy into mechanical motion, offering airflow for cooling.
- Washing Machines: Combine motors, sensors, and logic control to wash clothes efficiently.
- Elevators: Use motors and control systems to transport people or goods vertically within buildings.
Example: Consider an electric toothbrush. It is an efficient electromechanical device that converts electrical energy from a battery into rapid mechanical oscillations or rotations to effectively clean teeth.
Curious how elevators remain stable? The balance between the counterweight and cabin ensures safe and efficient operation.
A more interesting everyday application occurs in 3D Printers. These devices use stepper motors controlled by precise electrical signals to move print heads in exact patterns, layer by layer, creating three-dimensional objects. The principle of layered manufacturing is leveraged by advanced software that translates digital models into mechanical movement, showcasing the complexity of modern electromechanical systems. This requires a high degree of accuracy in both motion control and timing, highlighting the intricate relationship between the electrical and mechanical components.
Industrial Applications
Industrial uses of electromechanical systems are widespread, encompassing automation and heavy machinery to improve manufacturing and operational efficiency. Some key applications include:
- Automated Assembly Lines: Robots equipped with sensors and motors perform repetitive tasks accurately and rapidly.
- CNC Machines: Computer Numerical Control (CNC) machining uses precise electromotors to shape and cut materials according to programmed instructions.
- Power Generation: Electromechanical systems like turbines convert kinetic energy of wind or water into electrical power.
Example: Automated systems in car manufacturing plants use robotic arms to weld, paint, and assemble components, significantly enhancing production speed and reducing manual labor.
In power plants, turbines are critical electromechanical systems. These turbines convert mechanical energy to electrical energy via generators. Their blades capture kinetic energy, which is then converted to rotational energy. This rotation drives the generator to produce electricity according to Faraday’s law of electromagnetic induction. This principle is mathematically represented through the generator of EMF:\[ E = -N \cdot \frac{d\Phi}{dt} \]where \( E \) is the induced electromotive force, \( N \) is the number of coils, and \( \frac{d\Phi}{dt} \) is the rate of change of magnetic flux. Such systems play a pivotal role in energy sectors, demonstrating massive scales of electromechanical application.
Dynamics of Mechanical and Electromechanical Systems
Understanding the dynamics of mechanical and electromechanical systems is crucial for optimizing their design and functionality. These dynamics refer to the study of forces and torques and their effect on motion within systems, helping predict and improve performance in varied conditions.
Importance of Dynamics
The importance of dynamics lies in its influence on how systems operate at different levels. By studying dynamics, you can:
- Enhance system performance in terms of speed, efficiency, and accuracy.
- Predict system behavior under different forces and loads.
- Optimize design to accommodate environmental factors.
- Prevent failures by understanding stress and fatigue limits.
Remember, dynamics is not just about motion; it's about understanding the causes of motion and how they affect systems.
Key Dynamic Concepts
To fully grasp system dynamics, familiarize yourself with several key concepts:
- Newton's Laws of Motion: Fundamental laws that describe the relationship between a body and the forces acting upon it, forming the basis of classical mechanics.
- Force and Torque: Measurement and influence of forces can be calculated using equations such as \[ F = ma \] and the rotational analog \[ \tau = r \times F \].
- Momentum and Inertia: Momentum \( p = mv \) relates to moving bodies' mass and velocity, while inertia gives insight into resistance to change in motion.
- Damping and Vibration: Analyzing oscillations in systems and finding ways to control or utilize them is a big part of studying dynamics.
Example: In a rotating system, study of dynamic concepts allows you to model its behavior by considering every force acting across its axis. You can define equations like \( \tau = I \cdot \alpha \) where \( \alpha \) is angular acceleration and \( I \) is moment of inertia.
Analyzing System Dynamics
Analyzing system dynamics is about using mathematical models and simulations to predict how a system behaves under various conditions. To conduct a thorough dynamic analysis, you often employ:
- Mathematical Modeling: Representing system motion with differential equations.
- Computer Simulations: Using software tools such as MATLAB or Simulink to simulate the system's behavior over time.
- Experimental Testing: Validating models and simulations by comparing with real-world experiments.
Advanced Dynamics: In more advanced systems, engineers often deal with non-linear dynamics, where simple equations can't fully describe system behavior. Chaos theory, for instance, studies how very small changes in initial conditions can vastly affect outcomes. Such complexities require sophisticated numerical methods and computational power for modeling and analysis. Equation of chaos might resemble:\[ x_{n+1} = r x_n (1 - x_n) \]This logistic map demonstrates how systems can evolve unpredictably, even under deterministic rules, posing exciting challenges and research areas.
Electromechanical Systems Engineering Technology
Electromechanical Systems Engineering Technology is a field that bridges the gap between electrical engineering and mechanical engineering, focusing on the integration of these two disciplines. This area involves designing, creating, testing, and improving devices that combine electrical components with moving parts. From simple household appliances to complex industrial machinery, electromechanical engineering plays a pivotal role.
Role of Engineering Technology
Engineering technology in electromechanical systems encompasses a wide range of activities, from conceptual design to practical implementation and maintenance of systems. This discipline is crucial for driving innovation and improving the efficiency of mechanical systems through the application of electrical engineering principles.
- Design and Development: Engineering technologists engage in the design of products, ensuring that components and assemblies fit together to function as intended. They use software tools like CAD for designing components and assemblies.
- Troubleshooting and Maintenance: Technologists perform ongoing testing and troubleshooting of electromechanical devices, ensuring they meet performance requirements and function reliably over time.
- Automation and Control: Implementation of control systems that allow machines to operate autonomously or semi-autonomously, often involving programming PLCs (Programmable Logic Controllers) for industrial use.
Think of engineering technology as the practical application of theoretical engineering principles to solve real-world problems.
Example: Consider an automated packaging system in a factory. Here, engineering technologists design and implement conveyor systems powered by electric motors, program control systems for precise packaging tasks, and ensure everything runs smoothly with minimal downtime.
Applications in Electromechanical Systems
The range of applications for electromechanical systems is vast and includes both consumer and industrial contexts. Such systems leverage the synergy between mechanical mechanisms and electrical control to perform a variety of tasks.
- Consumer Electronics: Devices like washing machines or digital cameras incorporate motors, sensors, and circuits to deliver functionality efficiently.
- Transportation: Vehicles, especially electric and hybrid cars, utilize electromechanical systems for drivetrain components, regenerative braking, and more.
- Healthcare Devices: Equipment such as MRI machines or robotic surgery tools combine precise mechanical actions controlled by sophisticated electronic systems.
- Industrial Automation: Robotics in manufacturing plants automate monotonous or dangerous tasks, leveraging electromechanical principles to operate effectively.
In today's automotive industry, electromechanical systems are crucial for innovation in electric vehicles (EVs). These systems are central to their propulsion and energy recovery processes. EV batteries provide power to electric motors, converting electrical energy into mechanical energy for vehicle motion. Advanced electromechanical systems within EVs also enable energy recovery during braking periods through regenerative braking.This braking system functions by reversing the function of the electrical motor; instead of turning electrical energy into motion, the vehicle's inertia drives the motor, converting kinetic energy back to electrical energy stored in the battery. This recovery process is mathematically modeled by:\[ E_{regen} = \frac{1}{2}mv^2\]where \( E_{regen} \) is the energy recovered, \( m \) is mass, and \( v \) is velocity. Mastery of these energy conversions is integral to advancing EV efficiency and sustainability.
Future Trends in Technology
The future of electromechanical systems is poised for growth and innovation, driven by technological advances and increasing demand for efficient and sustainable systems.
- Smart Systems: The development of smart electromechanical systems that incorporate AI and IoT for enhanced automated control and monitoring.
- Renewable Energy Applications: Integration of electromechanical systems in solar and wind power to optimize energy conversion processes.
- Improved Materials: Advances in materials science lead to lighter and more durable components, enhancing system efficiency.
- Micro Electromechanical Systems (MEMS): Future applications in miniaturized systems like sensors and actuators used in medical, automotive, and consumer technologies.
electromechanical systems - Key takeaways
- Definition of Electromechanical Systems: Integrated systems combining electrical and mechanical elements to perform tasks via energy conversion.
- Design of Electromechanical Systems: Involves integrating electrical circuits and mechanical components to create efficient solutions in automation and machinery.
- Electromechanical Systems Examples: Electric motors, generators, robotic arms, and household appliances like washing machines and electric fans.
- Dynamics of Mechanical and Electromechanical Systems: Study of forces and motions in systems to optimize performance, often modeled with differential equations.
- Electromechanical Systems Engineering Technology: Focuses on merging electrical and mechanical engineering to design, develop, and maintain systems like automated machinery.
- Explanation of Electromechanical Systems: Systems that convert and integrate mechanical energy and electrical control to perform various operations, emphasizing efficiency and precision.
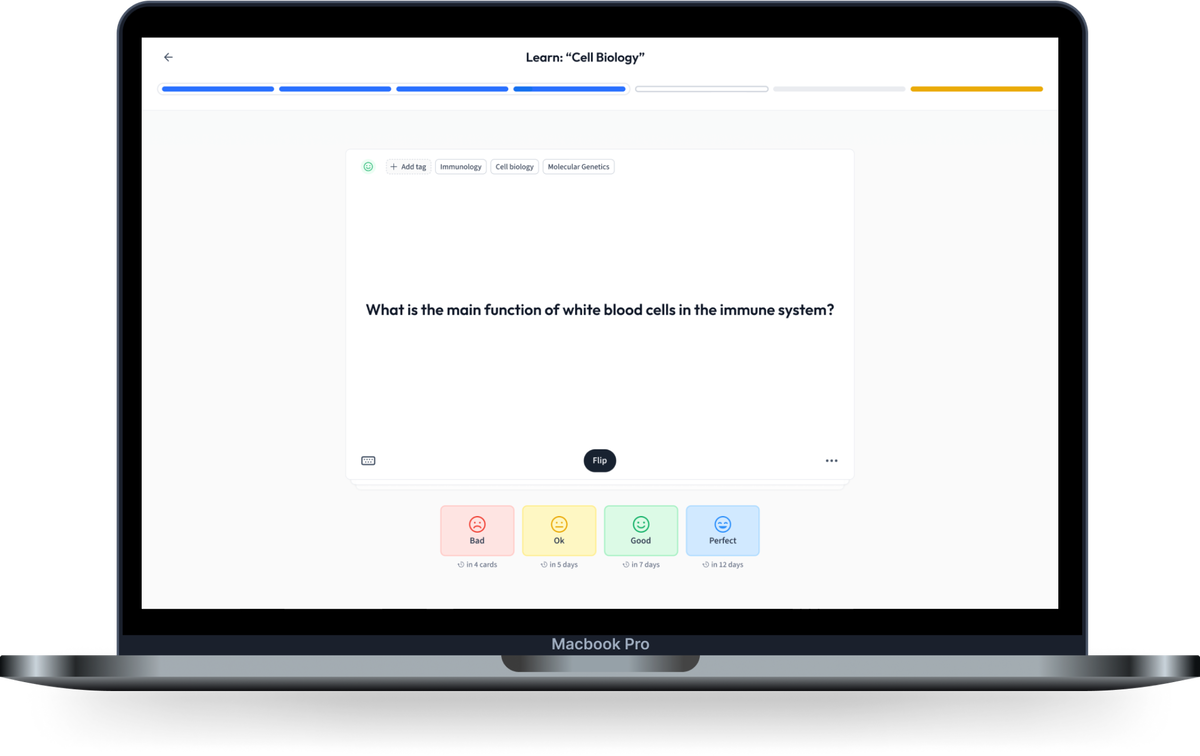
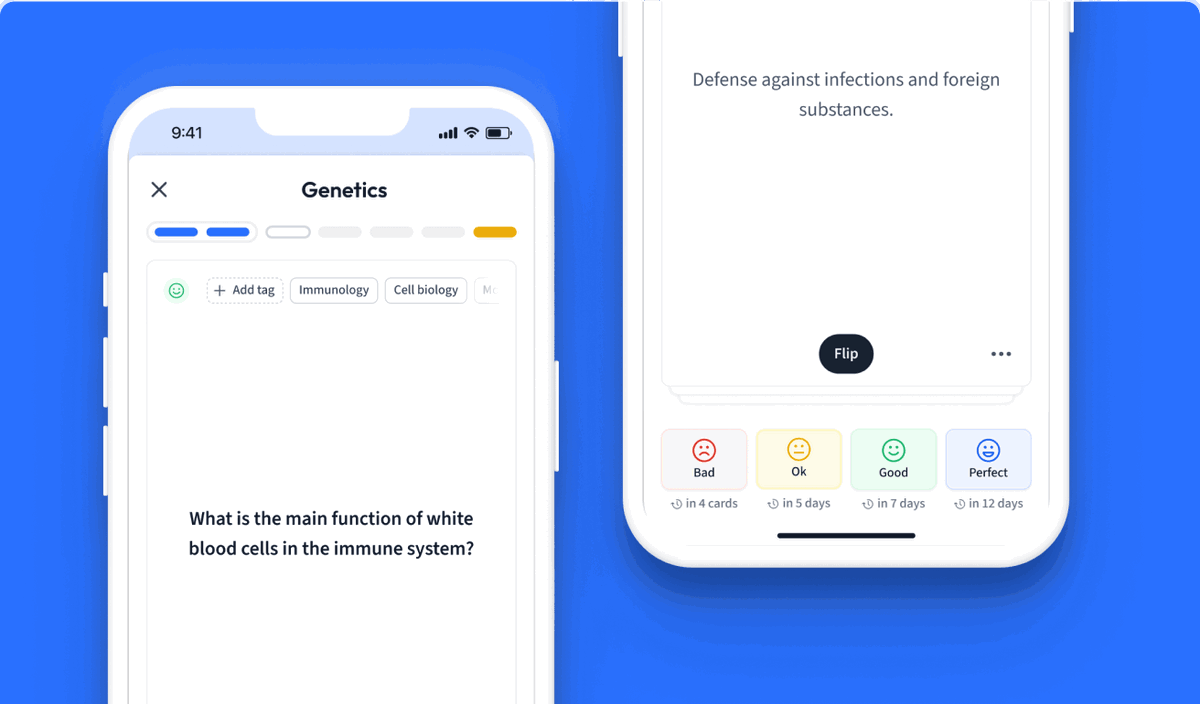
Learn with 12 electromechanical systems flashcards in the free StudySmarter app
Already have an account? Log in
Frequently Asked Questions about electromechanical systems
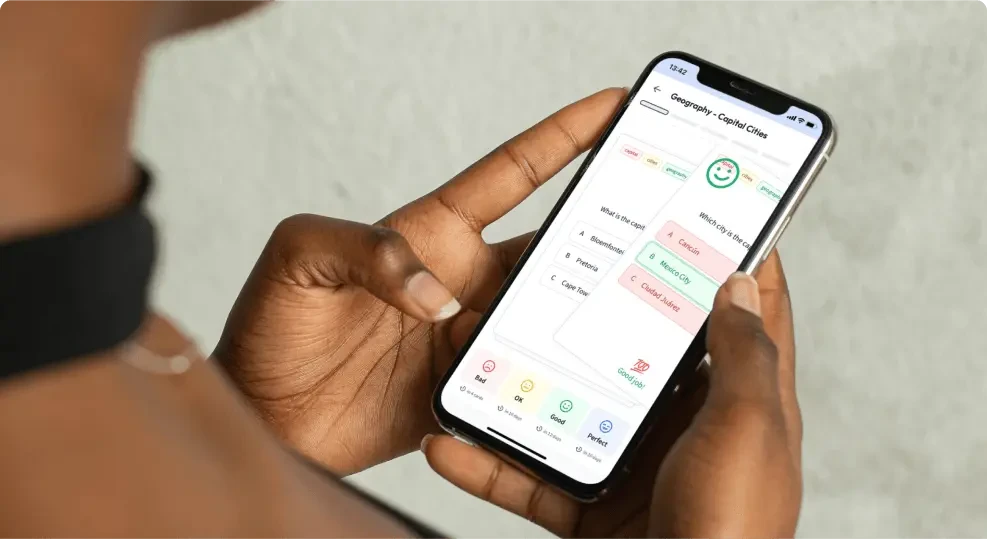
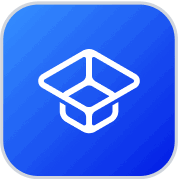
About StudySmarter
StudySmarter is a globally recognized educational technology company, offering a holistic learning platform designed for students of all ages and educational levels. Our platform provides learning support for a wide range of subjects, including STEM, Social Sciences, and Languages and also helps students to successfully master various tests and exams worldwide, such as GCSE, A Level, SAT, ACT, Abitur, and more. We offer an extensive library of learning materials, including interactive flashcards, comprehensive textbook solutions, and detailed explanations. The cutting-edge technology and tools we provide help students create their own learning materials. StudySmarter’s content is not only expert-verified but also regularly updated to ensure accuracy and relevance.
Learn more