Jump to a key chapter
Environmental Biomechanics Definition
Environmental biomechanics is a field that combines principles of mechanics with ecological and environmental considerations. It seeks to understand how living organisms interact with their physical surroundings, often with a focus on sustainability and adaptation to changing environments.
Understanding Biomechanics
Biomechanics is the study of forces and their effects on biological systems. It applies principles from physics, particularly mechanics, to analyze the movement and structure of living organisms. Key aspects include analyzing how bones, muscles, and joints coordinate to produce movement. Understanding these interactions helps in fields such as sports science, medicine, and prosthetics design.
Biomechanics: The scientific study of the mechanics of living organisms.
An example of biomechanics in action is the analysis of a runner's gait. By studying the forces exerted by the feet on the ground and the resulting movements, adjustments can be made to improve performance and reduce injury risk.
Biomechanics can be both a micro (cellular level) and macro (organism level) science.
Key Concepts of Environmental Biomechanics
In environmental biomechanics, you study how ecological factors influence the biomechanics of organisms. Key concepts include:
- Sustainability: Understanding how an organism's movements affect and are affected by its environment.
- Adaptation: How organisms evolve biomechanical strategies to survive in diverse environments, from aquatic habitats to urban landscapes.
- Resilience: Studying how organisms withstand environmental stresses and recover from extreme conditions.
The relationship between environmental biomechanics and climate change is a growing area of study. As environments change, organisms must adapt their biomechanical strategies to survive. For instance, polar bears have evolved to thrive in cold environments, with specialized paws for walking on ice. However, as ice caps melt, their biomechanics may need to adjust to new conditions, potentially affecting their survival.
Differences Between Engineering Biomechanics and Environmental Biomechanics
While both engineering biomechanics and environmental biomechanics involve the study of force interaction, they focus on different applications and scales:
- Engineering Biomechanics: Concentrates on designing systems and devices, such as prosthetics or machinery, that interface with the human body. It often emphasizes functionality and improving performance through mechanical intervention.
- Environmental Biomechanics: Emphasizes the interaction of organisms with their environments. It focuses on how natural surroundings influence physical capabilities and adaptations of organisms, often promoting sustainability.
A classic example differentiating the two is examining the stress on a bridge (engineering biomechanics) versus analyzing how a fish swims upstream against a current (environmental biomechanics). The former focuses on constructing a stable structure, while the latter looks at biological adaptation to environmental forces.
Both fields can complement one another. Analyzing human biomechanics in different environmental settings can enhance engineering designs for wearable technology.
Environmental Biomechanics Significance
The field of environmental biomechanics holds substantial significance in understanding how organisms interact within their ecosystems. This discipline integrates mechanical principles with environmental contexts, emphasizing sustainable solutions and adaptive strategies.
Importance in Mechanical Engineering
Environmental biomechanics has a profound impact on the field of mechanical engineering by offering insights into designing systems that blend with natural environments. Key areas include:
- Biomimicry: Engineers often mimic natural designs, such as the structure of a bird's wing in developing aerodynamic vehicles.
- Eco-friendly Materials: Utilizing materials and designs that minimize environmental impact, such as biodegradable components.
- Hydrodynamic Efficiency: Learning from aquatic organisms to develop efficient water-based transportation systems.
Biomechanics Definition: The interdisciplinary study combining principles of mechanics with the analysis of biological systems.
A notable example of environmental biomechanics in engineering is the development of wind turbines inspired by the fin movements of humpback whales. The unique design of these fins, featuring bumps or 'tubercles', has been found to increase the turbine's efficiency by 20%.
Engineers often use environmental biomechanics to study animal locomotion, which can lead to breakthroughs in robotics.
Environmental Biomechanics in Daily Life
In daily life, environmental biomechanics can be seen in various aspects of urban planning and personal mobility. Considerations include:
- Urban Design: Creating pedestrian-friendly spaces that encourage walking and cycling, modeled after natural human movement patterns.
- Recreational Equipment: Designing sports gear that minimizes injury risk, such as shoes that align with biomechanical principles for better foot support.
- Public Health: Using studies of human biomechanics to advocate for walkable cities that improve overall health and well-being.
In the context of public health, understanding environmental biomechanics allows urban planners to design cities that promote physical activity, potentially reducing the incidence of diseases like obesity. Consider the equilibrium equation used in biomechanics \[ F = ma \] where \( F \) is force, \( m \) is mass, and \( a \) is acceleration. This principle is vital when examining how various surfaces and incline levels in urban environments affect mobility and energy expenditure.
An application of environmental biomechanics in everyday life is the Segway, a two-wheeled personal transporter. Its balance control system is inspired by the human body's natural walking mechanics, using weight shifts to steer and accelerate.
From architecture to sports, environmental biomechanics offers insights that lead to innovative solutions and improved designs.
Engineering Biomechanics and Biomechanical Engineering
Engineering biomechanics involves applying principles of engineering to the study of the mechanical aspects of living organisms. It is essential for designing systems and technologies that synchronize with the human body, contributing to fields like medical device development and robotic applications.
Core Principles of Biomechanical Engineering
The core principles of biomechanical engineering focus on understanding and replicating the mechanical dynamics of biological systems. Key principles include:
Mechanical Dynamics: The study of forces and motions acting on a system. In biomechanics, this often involves forces within the human musculoskeletal system.
- Force Analysis: Analyzing forces within biological systems is crucial. This includes calculating net forces using equations like \(\sum F = ma\), where \( F \) is force, \( m \) is mass, and \( a \) is acceleration.
- Materials Science: Selecting appropriate materials that mimic or interact well with biological tissues, ensuring compatibility and functionality.
- Mathematical Modeling: Using equations and computational models to simulate biological behaviors and predict outcomes, such as the stress-strain relationship within tissues.
Consider prosthetic limb designs: they leverage the principle of force analysis by using sensors to detect user movement, enabling the limb to move in coordination with the user's body.
Advanced biomechanical engineering involves creating exoskeletons for rehabilitation. These devices often use complex algorithms to interpret neural signals and adjust movement assistance, offering a realistic interaction between human intent and mechanical function. The design integrates feedback loops and control systems, often modeled through differential equations such as \( Mx''(t) + Dx'(t) + Kx(t) = F(t) \), where \( M \), \( D \), and \( K \) represent mass, damping, and stiffness matrices, and \( F(t) \) represents applied forces over time.
Integration of Engineering and Environmental Biomechanics
The integration of engineering and environmental biomechanics is crucial in creating systems that not only adhere to mechanical requirements but also respect environmental constraints. This integration supports sustainable development and ecological balance.
Key aspects of this integration include:
- Sustainable Engineering: Designing systems that minimize environmental impact while being effective in their biomechanical roles. This includes using recyclable materials and energy-efficient processes.
- Adaptation to Environmental Constraints: Understanding how natural ecosystems operate allows engineering solutions that harmonize with them. For instance, developing transportation systems that mimic natural animal movement can reduce energy consumption.
Studying bird flight mechanics has inspired the design of aerodynamic vehicles, demonstrating the synergy between engineering and environmental biomechanics.
Biomechanics Applications
Biomechanics finds its applications across multiple fields, combining mechanical principles with biological systems to create solutions that enhance both life quality and environmental sustainability.
Real-world Applications of Environmental Biomechanics
Real-world applications of environmental biomechanics highlight the practical uses of biomechanical principles to solve environmental challenges and improve living conditions. These applications include:
- Eco-friendly Architecture: Designing buildings that align with natural human movement and reduce energy waste. For example, structures may utilize passive solar designs that position windows and ventilation to maximize natural light and airflow.
- Sustainable Transport Systems: Improving public transportation models by studying human dynamics, such as how pedestrian flow can be optimized in subway systems.
- Wildlife Conservation: Developing conservation strategies that take into account the biomechanics of animals. For example, designing wildlife corridors to ensure safe passage for species whose natural movements are hindered by human developments.
Environmental Biomechanics: A field focusing on the interaction between biological systems and their physical environment with sustainability in mind.
A practical example is the design of biomimetic wind turbines inspired by the fins of humpback whales. These turbines' innovative aerodynamic designs increase efficiency and reduce noise, making them more viable for use near residential areas.
Environmental biomechanics also plays a role in developing ergonomic urban furniture, which enhances comfort and usability for diverse populations.
The integration of biomechanics in environmental planning extends to smart cities, which seek to optimize resources by modeling their infrastructure on the biomechanics of natural ecosystems. This includes utilizing algorithms to predict urban growth patterns and designing transportation systems that mimic efficient biological pathways. Researchers use mathematical models, such as differential equations, to analyze and predict these complex systems. Consider the equation for human locomotion efficiency: \[ W = \frac{F \times d}{t} \] where \( W \) is work done, \( F \) is force applied, \( d \) is distance traveled, and \( t \) is time taken.
Innovations in Biomechanical Engineering
The field of biomechanical engineering is continually evolving, introducing cutting-edge technologies and methodologies to enhance how human and artificial systems interact. Innovations include:
- Wearable Technology: Developing devices that monitor and analyze biomechanical data in real time, providing insights into human movement and health.
- Advanced Prosthetics: Creating prosthetic limbs that closely mimic the range of motion and responsiveness of natural limbs. These prosthetics often include actuators and sensors that adjust movements based on user feedback.
- Exoskeletons: Designing robotic suits that aid human movement, particularly for rehabilitation or enhancing strength in laborious tasks.
In the medical field, robotic exoskeletons are used to assist patients with spinal cord injuries in regaining mobility, providing support and feedback through integrated computer systems.
One of the most intriguing developments is using 3D printing technology in biomechanical engineering. This process allows the creation of customized implants and prosthetics tailored to individual anatomical features. Engineers use biomechanical data to design components with precise geometries that mimic natural structures, optimizing fit and function. Biocompatible materials used in 3D printing reduce the likelihood of rejection and increase the longevity of implants. Considerations of stress distribution are crucial, analyzed through equations like:\[ \tau = \frac{F}{A} \]where \( \tau \) represents shear stress, \( F \) is the force exerted, and \( A \) is the area over which the force is applied.
Innovations in biomechanical engineering can also lead to breakthroughs in sport performance enhancements and injury prevention.
environmental biomechanics - Key takeaways
- Environmental biomechanics is a field that combines mechanics with ecological considerations to understand organism-environment interactions, focusing on sustainability and adaptation.
- Biomechanics applies physics to biological systems, analyzing movement and structure, crucial in sports science and medical fields.
- Difference: Engineering biomechanics focuses on designing biomechanical systems, while Environmental biomechanics emphasizes organism-environment interactions.
- Significance of Environmental Biomechanics lies in promoting sustainable ecosystem interactions and influencing designs in mechanical engineering.
- Applications of environmental biomechanics include eco-friendly architecture, sustainable transport, and wildlife conservation considering biomechanics.
- Biomechanical engineering integrates mechanical dynamics and biological systems for innovations in prosthetics and robotics, enhancing human-machine interaction.
Learn faster with the 12 flashcards about environmental biomechanics
Sign up for free to gain access to all our flashcards.
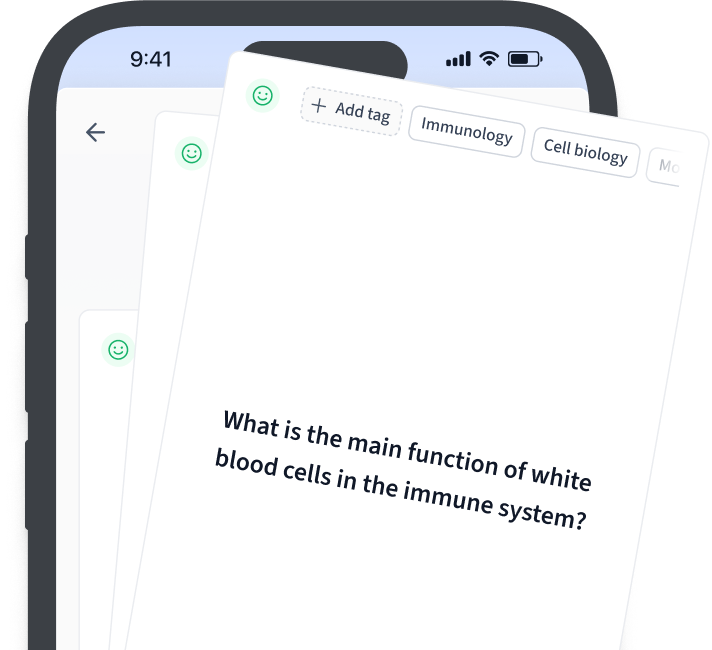
Frequently Asked Questions about environmental biomechanics
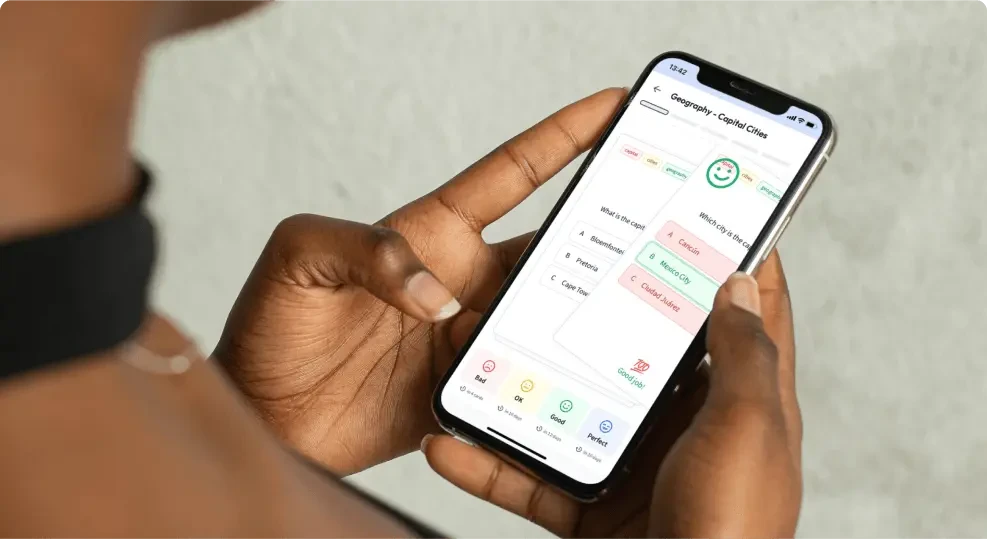
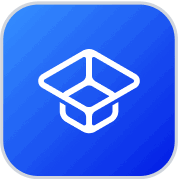
About StudySmarter
StudySmarter is a globally recognized educational technology company, offering a holistic learning platform designed for students of all ages and educational levels. Our platform provides learning support for a wide range of subjects, including STEM, Social Sciences, and Languages and also helps students to successfully master various tests and exams worldwide, such as GCSE, A Level, SAT, ACT, Abitur, and more. We offer an extensive library of learning materials, including interactive flashcards, comprehensive textbook solutions, and detailed explanations. The cutting-edge technology and tools we provide help students create their own learning materials. StudySmarter’s content is not only expert-verified but also regularly updated to ensure accuracy and relevance.
Learn more