Jump to a key chapter
Feedback Loop Definition
Feedback loops are essential concepts in engineering that describe the process by which a system's output is returned to its input to maintain a desired result or improve the system's functioning. Understanding feedback loops is crucial in various fields, such as control systems, electronics, and biological systems, as they help regulate and stabilize processes.
Key Characteristics of Feedback Loops
- Feedback Mechanism: The output of a system is fed back into the system as input. This can either be done directly or through a series of components that adjust the signal accordingly.
- Stability: Feedback loops contribute to the stability of a system by compensating for any disturbances or changes.
- Response Time: The efficiency of a feedback loop often depends on how quickly it can respond to changes or disturbances in the system.
Types of Feedback Loops
In engineering, there are two primary types of feedback loops: positive feedback and negative feedback.
Positive Feedback Loop: This type of loop enhances or amplifies the initial input, potentially leading to exponential growth or output, like in the case of a microphone that causes a loud screech when the sound is fed back into it.
Negative Feedback Loop: This loop dampens or reduces the initial input to stabilize the output, commonly used in thermostats to maintain a consistent temperature.
Example of a Negative Feedback Loop: Consider the cruise control system in a car. The system monitors the vehicle's current speed and compares it to the desired speed. If a hill causes the car to slow down, the feedback loop detects the change and adjusts the engine power to maintain the set speed.
Mathematical Representation of Feedback Loops
Mathematically, feedback loops can be represented using equations. A simple example of a feedback loop in control theory is:The closed-loop transfer function (T) is represented as: \[ T = \frac{G}{1 + GH} \]where G is the forward gain, and H is the feedback gain. The denominator 1 + GH determines the stability and behavior of the entire system.
Feedback loops are not limited to engineering applications. They exist widely in nature and economics. For instance, the body's regulation of glucose levels is a feedback loop. It involves hormones like insulin and glucagon to maintain blood sugar levels. In economics, market corrections are feedback mechanisms that stabilize price fluctuations over time. Such loops reflect how universal the concept is and its importance in understanding dynamic systems.
Feedback Loop Explained
A feedback loop is a fundamental mechanism in systems where the output is monitored and adjusted to achieve a desired performance. Understanding feedback loops is vital for analyzing and controlling systems in various fields, from engineering to biology. It ensures stability and efficiency by modulating input based on the output.
Types of Feedback Loops
Feedback loops can generally be classified into two categories: positive and negative. Each type has distinct characteristics and applications.
Positive Feedback Loop: Increases the output by adding to the initial input, which can lead to growth or amplification in the system’s output.
Negative Feedback Loop: Reduces the difference between the desired and actual outputs by adjusting the input, thereby stabilizing the system.
Example of Positive Feedback:A classic example of a positive feedback loop is the process of childbirth. The hormone oxytocin increases contractions, which signals more oxytocin release, further enhancing contractions until delivery occurs.
Example of Negative Feedback:The regulation of body temperature in humans is controlled by a negative feedback loop. When the body temperature increases, it triggers sweating to cool down, subsequently lowering the temperature back to its set point.
Mathematical Representation
Feedback loops can be described using mathematical expressions to quantify their behavior. A typical control system feedback loop is represented by the formula: The transfer function of a feedback system is given by: \[ T = \frac{G}{1 + GH} \] where:
- G is the open-loop gain.
- H is the feedback factor.
Feedback loops are pivotal not only in engineering but also in nature and technology. For example, ecological systems use feedback loops to maintain balance. Predator-prey dynamics adjust populations to sustain ecosystems, serving as natural control mechanisms. Additionally, in economics, feedback loops regulate market forces. Supply-demand interactions create a natural balance of pricing, showcasing feedback's role in economic equilibrium. The understanding of such loops extends beyond theoretical studies, providing insights into real-world applications where such mechanisms are crucial.
Remember, feedback loops can sometimes lead to oscillations if improperly managed, especially when dealing with high gains in positive feedback systems.
Negative Feedback Loop in Mechanical Engineering
Negative feedback loops play a critical role in mechanical engineering, ensuring systems are stable and functioning efficiently. They help in adjusting the input automatically to reduce any discrepancies between the desired and actual outcomes, which is essential for precision and control in engineering applications.
Examples of Negative Feedback Loop
Negative feedback loops are found in a variety of mechanical systems:
- Thermostats: A thermostat uses a negative feedback loop to maintain a targeted temperature. When the temperature deviates from the desired setting, the system activates heating or cooling to bring it back to the set point.
- Cruise Control in Cars: This system maintains a car's speed by monitoring its current speed against the desired speed. If the car slows down, the system will increase power to the engine; if it speeds up, it will reduce power.
- Automatic Voltage Regulators: Employed in power systems to maintain steady voltage levels, they adjust the input voltage according to changes in the load to ensure consistent output.
Example:Consider an air conditioning system where a thermostat measures room temperature. If the room becomes too warm, the thermostat activates the cooling mechanism, reducing the temperature, and vice versa if it gets too cold.
In many engineered systems, negative feedback is crucial to prevent components from overheating.
Benefits of Negative Feedback Loop
The implementation of negative feedback loops in mechanical systems offers several advantages:
- Stability: Negative feedback stabilizes systems by automatically correcting any deviations from the desired operation.
- Increased Accuracy: By continuously adjusting inputs, these loops enhance the precision of system outputs.
- Energy Efficiency: They help in conserving energy by only making necessary adjustments, reducing power consumption.
- Reduced Wear and Tear: Because operations are smoother and more controlled, these loops can lessen mechanical stress and prolong equipment life.
Negative feedback loops are not just a tool for stabilization. In control systems, they are fundamental in the design of PID controllers (Proportional-Integral-Derivative controllers), which are used across engineering disciplines. These controllers adjust the system to correct errors by considering past, present, and future consequences of changes, offering a comprehensive approach to control theory that can be applied to complicated dynamic systems. Such integrative feedback mechanisms are critical in industries ranging from automotive to aeronautics, illustrating the importance of negative feedback across diverse applications.
In the context of electronics, negative feedback can substantially widen the bandwidth of amplifiers, improving their performance.
Positive Feedback Loop in Mechanical Engineering
Positive feedback loops are mechanisms in engineering where the output of a system is amplified or magnified by feeding the output back into the system as an input. These loops can lead to growth or escalation in the system's operation, and understanding them is crucial in both theoretical studies and practical applications, as they can sometimes lead to instability if not controlled properly.
Examples of Positive Feedback Loop
Positive feedback loops are commonly encountered in several mechanical systems, even though they are less frequent than negative feedback loops due to their propensity to cause instability. Here are some notable examples:
- Microphone and Speaker Feedback: When a microphone feeds sound into a speaker, and the sound is picked up again by the microphone, it creates an increasing sound loop, known as the feedback loop, often resulting in a high-pitched squeal.
- Engineering Oscillators: Positive feedback is used intentionally in oscillators to maintain oscillations without decay, which is fundamental for generating consistent waveforms in electronic circuits.
- Heating Systems: Though less common, certain heating systems may use positive feedback to rapidly bring a system to operating temperature.
Example: In electronic oscillators, positive feedback maintains oscillations. By feeding a portion of the output back to the input without inversion, the oscillations sustain themselves. Mathematically, this can be represented as:\[ V_{out} = G(V_{in} + V_{feedback}) \]where G is the gain, illustrating how the input voltage is added to the feedback voltage to maintain continuous operation.
In engineering, controlling a positive feedback loop requires careful design to avoid unwanted escalation and potential system failure.
Impacts of Positive Feedback Loop
The presence of a positive feedback loop can have a range of effects on a mechanical system, which include:
- Increased Output: A key characteristic of positive feedback is its ability to increase system output, sometimes exponentially. This can be beneficial in systems designed for rapid response or action.
- Potential Instability: Without proper control, an unchecked positive feedback loop can cause a system to become unstable, leading to possible damage or failure.
- Shock Amplification: Sudden changes in input can be greatly magnified, which might be advantageous or detrimental, depending on the application.
In nature and technology, positive feedback loops can lead to fascinating phenomena. For example, in climatology, ice-albedo feedback is a positive loop where melting ice reduces reflectivity, causing more heat absorption and further ice melting. In the realm of technology, digital revolution advancements have exponentially increased due to positive feedback effects, where technology advancements feed further developments in a cycle of rapid innovation. Both instances highlight not only the importance but the potential risks and rewards associated with understanding and harnessing positive feedback mechanisms.
Positive vs Negative Feedback Loop
Feedback loops are categorized into positive and negative types, each serving unique roles in system dynamics and stability. Both are vital across engineering disciplines for enhancing or stabilizing system performance.
Differences Between Positive and Negative Feedback Loop
While both feedback loops involve feeding output back into the system, their effects are markedly different:
- Positive Feedback Loop: Amplifies changes or deviations in the system, often leading to exponential growth. For instance, in electronics, it can sustain oscillations in circuits.
- Negative Feedback Loop: Mitigates changes or deviations, promoting stability. A thermostat is a common example, maintaining constant room temperatures.
Positive Feedback Loop: Increases the system's output by reinforcing initial conditions. Represented mathematically by:\[ A_{system} = \frac{A_{open}}{1 - A_{open}H} \] where a high value of A_{open}H can lead to instability.
Example: Consider an electronic amplifier circuit where positive feedback is applied. The feedback increases the amplifier's gain, potentially leading to self-oscillation if not controlled.
Negative Feedback Loop: Reduces discrepancies by adjusting input to stabilize the output. Commonly used to maintain desirable states in a system.
Example: In an audio system, negative feedback can be used to reduce distortion by comparing the system's output to the desired input.
Understanding feedback loops extends beyond engineering. For instance, biological feedback mechanisms like insulin regulation in the human body showcase negative feedback at work, maintaining homeostasis. Conversely, in social systems, crowd behavior can reflect positive feedback, where individual actions amplify communal responses, affecting trends and decision-making processes on a larger scale. Both examples illustrate how comprehending feedback loops can provide deeper insights into natural and artificial systems.
Choosing the Right Feedback Loop
Selecting the appropriate feedback loop for a system depends on the desired outcome: stability or amplification. Key considerations include:
- Objective: Determine whether stabilization or signal amplification is needed.
- System Characteristics: Analyze system dynamics and external disturbances.
- Potential Instability: Consider the risk of over-amplification in positive loops, affecting system integrity.
- Response Time: Negative feedback systems typically have faster response times to disturbances.
In precision applications, such as instrumentation, negative feedback is often preferred due to its ability to provide more accurate and stable outputs.
Feedback Loop Applications in Engineering
Feedback loops are integral components in engineering systems. By applying feedback loops, engineers can enhance system stability, efficiency, and performance. The use of these loops is crucial in various real-world applications and contributes significantly to advancements and innovations in engineering.
Real-World Feedback Loop Applications
Feedback loops are utilized widely across multiple engineering disciplines to optimize and control processes. Their applications include:
- Automotive Engineering: Feedback loops are used in cruise control systems to maintain the vehicle's speed by adjusting throttle positions based on speed sensor inputs.
- Electronics: In electronic amplifiers, negative feedback helps in reducing distortion and increasing the bandwidth of the signals processed.
- Aerospace: Autopilot systems in aircraft use feedback loops to maintain desired flight paths by adjusting control surfaces in response to environmental changes.
- Robotics: Feedback loops enable robots to adapt to their environment by altering movements based on sensory inputs.
Example: In a thermostat used within HVAC systems, the desired temperature is maintained via a feedback loop. The current room temperature is compared to the desired setpoint, and adjustments to heating or cooling are made accordingly.
Positive Feedback Loop: Increases the system's output by amplifying the initial conditions. Common in oscillatory systems to sustain certain states or operations.
Negative Feedback Loop: Functions to stabilize systems by reducing deviations from desired outputs. Common in control systems where precise outcomes are needed.
Example:Consider an audio amplifier circuit employing negative feedback. Here, part of the output is inverted and fed back to the input, reducing distortion and stabilizing gain. This can be mathematically expressed as: \[ V_{out} = G \left( V_{in} - V_{feedback} \right) \] where G represents the system gain.
In the realm of bioengineering, feedback loops are even modeled to replicate biological processes. For example, synthetic gene circuits are often designed using electronic circuit principles, where feedback mechanisms control protein synthesis rates, mimicking natural biological systems. Such integration highlights the versatility and cross-disciplinary applications of feedback loops beyond conventional engineering.
In automotive applications, adaptive cruise control systems employ feedback loops to maintain a safe following distance by automatically adjusting speed.
Innovations Using Feedback Loops in Engineering
Feedback loops have spurred numerous innovations in engineering, reshaping how systems are designed and operated.
- Smart Grids: These use feedback loops to balance energy load and supply dynamically, ensuring efficient energy distribution and robustness.
- 3D Printing: Incorporates feedback to adjust printing parameters on-the-fly, improving the accuracy and quality of printed objects.
- Environmental Control: Feedback is employed to regulate climate systems within greenhouses or large buildings, optimizing plant growth and energy use.
- Autonomous Systems: Feedback loops are integral for the development of autonomous vehicles and drones, allowing them to make real-time decisions based on environmental feedback.
Example:The implementation of feedback in smart grids can be demonstrated in load balancing algorithms. These systems analyze energy data and automatically adjust production levels to match consumption needs, furthering efficiency and reducing waste.
Innovative advancements, such as feedback loops in nanotechnology, have opened new possibilities in materials processing and medicine. In nanofabrication, feedback mechanisms at the nanoscale are used to rectify process deviations, ensuring precision in material structuring. Similarly, feedback-regulated drug delivery systems in medical engineering provide controlled release of therapeutics in response to biological signals, demonstrating cutting-edge applications of feedback principles.
Feedback loops in environmental engineering allow for the monitoring and adaptive control of pollution levels in urban areas, offering real-time solutions to environmental challenges.
feedback loops - Key takeaways
- Feedback Loops Definition: A mechanism in systems where the output is recycled back to the input to stabilize or enhance system performance.
- Negative Feedback Loop: Reduces discrepancies by adjusting the input to stabilize the output, used in systems like thermostats and cruise control.
- Positive Feedback Loop: Amplifies the initial input, potentially causing exponential growth, such as in childbirth or microphone feedback.
- Positive vs Negative Feedback Loop: Positive loops enhance deviations leading to growth, while negative loops stabilize by counteracting changes.
- Feedback Loop Applications in Engineering: Used in automotive engineering, electronics, aerospace, and robotics to control and optimize processes.
- Mathematical Representation: Feedback systems can be described mathematically, affecting system stability and response via formulas like the closed-loop transfer function.
Learn faster with the 12 flashcards about feedback loops
Sign up for free to gain access to all our flashcards.
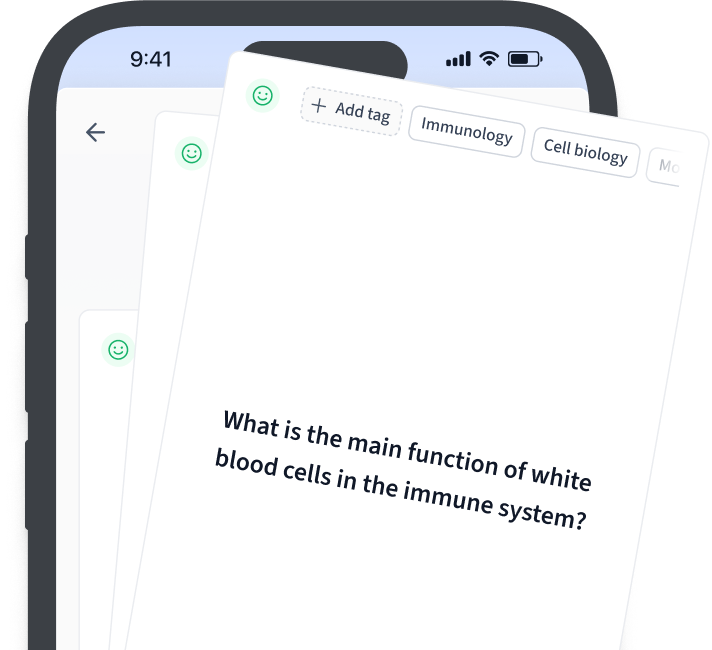
Frequently Asked Questions about feedback loops
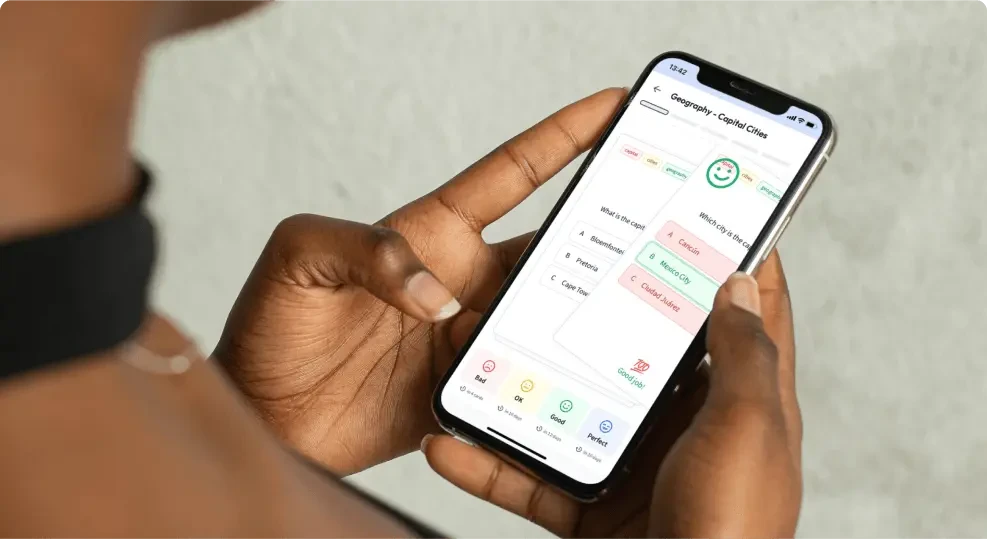
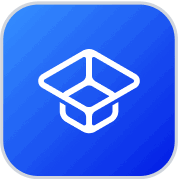
About StudySmarter
StudySmarter is a globally recognized educational technology company, offering a holistic learning platform designed for students of all ages and educational levels. Our platform provides learning support for a wide range of subjects, including STEM, Social Sciences, and Languages and also helps students to successfully master various tests and exams worldwide, such as GCSE, A Level, SAT, ACT, Abitur, and more. We offer an extensive library of learning materials, including interactive flashcards, comprehensive textbook solutions, and detailed explanations. The cutting-edge technology and tools we provide help students create their own learning materials. StudySmarter’s content is not only expert-verified but also regularly updated to ensure accuracy and relevance.
Learn more