Jump to a key chapter
Meaning of Flexible Mechanisms in Engineering
Flexible mechanisms in engineering refer to systems that can change shape or characteristics to perform various functions without the need for traditional joints or hinges. Flexibility within these systems allows for simpler and often lighter designs, which can lead to increased efficiency and lower production costs.
Key Characteristics of Flexible Mechanisms
Flexible mechanisms possess unique properties that make them advantageous in numerous applications:
- Adaptability: Ability to change shape according to the required function without additional tools or components.
- Reduced Complexity: Fewer moving parts mean fewer chances for mechanical failure.
- Material Efficiency: Lightweight materials can be used, saving costs and resources.
In the context of engineering, a flexibility mechanism is defined as a system or component that can perform multiple functions or actions by changing its configuration, often without separate or additional articulation methods.
Applications of Flexible Mechanisms
Flexible mechanisms can be applied in various engineering fields due to their distinct advantages:
- Robotics: Robots with flexible grippers that can handle delicate objects without damaging them.
- Aerospace: Wings that can change shape to optimize for different flight conditions.
- Medical Devices: Instruments that adapt to the contours of the body.
Consider a compliant mechanism like a pair of tweezers. These are engineered to flex and transform force without extra mechanical joints, showing a practical application of flexibility in everyday tools.
Recent advances in Smart Materials have expanded the realm of flexible mechanisms. These materials can change properties in response to environmental stimuli such as temperature, light, and pressure. They offer exciting possibilities for creating more responsive and interactive systems.In fields like soft robotics, the development of flexible mechanisms has revolutionized tasks that involve unpredictable environments or sensitive operations. For instance, search-and-rescue robots with soft exteriors can navigate rubble without causing harm to survivors or themselves.Another emerging innovation involves origami-inspired designs, where folding patterns are crucial in creating flexible systems. These designs can be compact when not in use and expand when needed, reflecting efficiency and space-saving functionality.
Techniques for Designing Flexible Mechanisms
Understanding how to design flexible mechanisms is crucial for innovation in various engineering fields. These designs often require a creative approach to balance flexibility with structural integrity.By considering different aspects of engineering principles, you can optimize the efficiency and reliability of these systems.
Material Selection
The choice of material is a key factor when designing flexible mechanisms:
- Elastomers: Ideal for applications needing significant deformation, such as seals or vibration damping.
- Metals: Used in structures where durability under strain is important, like in spring systems.
By utilizing finite element analysis (FEA), you can simulate how different materials react under the same conditions. This approach supports decision-making in material selection based on stress distribution and potential failure points. The relationship among material properties is often represented mathematically, using equations such as Hooke's Law for elastic materials: \[ \sigma = E \cdot \epsilon \] where \( \sigma \,\) is the stress, \( E \,\) is the elastic modulus, and \( \epsilon \,\) represents strain.
Geometrical Considerations
Certain geometric designs can greatly enhance the flexibility of a mechanism:
- Tapered Structures: Helps in distributing stress more evenly.
- Curved Shapes: Allows bending while maintaining the cross-sectional area.
- Thin Walls: Offers flexibility but must be balanced with structural strength.
Simplifying geometry can lead to increased flexibility but may compromise integrity. Use design iterations to find the optimal configuration.
In practice, a common example is the design of compliant hinges used in microelectromechanical systems (MEMS). These hinges rely on geometric characteristics to bend while allowing rotational motion without traditional pivot points. A mathematical representation can be modeled using beam theory, where the bending stress \[ \tau = \frac{M\cdot c}{I} \] is a function of the applied moment \(M\,\text{, }\) the distance to the neutral axis \(c\,\text{, }\) and the moment of inertia \(I\,\text{.}\)
Applications of Flexible Mechanisms
Flexible mechanisms are increasingly important across various industries due to their adaptability and efficiency. By allowing motion through the deformation of materials rather than traditional joints, these mechanisms simplify designs and reduce maintenance needs.
Industrial Automation
In industrial settings, flexible mechanisms are utilized to improve production precision. For instance, in pick-and-place robots, flexible grippers adjust to handle products of different shapes and sizes, enhancing the efficiency of assembly lines.
- Adaptive control for variability in product lines.
- Reduction of maintenance needs due to fewer mechanical parts.
- Lower energy consumption thanks to lightweight materials.
Robotic arms equipped with compliant joints are an example where flexibility is crucial. These joints can mimic human arm movements, offering precise and gentle interaction with delicate items during manufacturing processes.
Aerospace Engineering
The aerospace industry benefits immensely from flexible mechanisms, particularly in reducing aircraft weight and improving aerodynamic efficiency. Flexing wing designs allow aircraft to:
- Adapt to varying flight conditions with minimal energy expenditure.
- Ensure optimal lift-to-drag ratios, crucial for fuel efficiency.
NASA and other research agencies are deeply exploring the potential of morphing wing technologies for future-generation aircraft.
Medical Devices and Healthcare
In the field of healthcare, flexible mechanisms have sparked innovations in the design of medical instruments and devices. Instruments that adjust to a patient's body contours can provide better results. For example, flexible endoscopes allow for non-invasive exploration of internal organs, leading to faster and more precise diagnostics.
Developments in soft robotics have furthered the creation of prosthetic limbs with flexible joints that provide a range of steady, human-like movements. This innovation enhances user comfort and control.Mathematically, the deformation of soft robotic structures can be examined through continuum mechanics using complex models such as the finite element method (FEM). The stress-strain relationship for these materials is typically nonlinear, described by equations such as:\[ \tau = C_1 (\frac{\theta}{m}) + C_2 \theta^2\] where \(C_1\) and \(C_2\) are material constants, \(\theta\) is the angle of deformation, and \(m\) represents mass per unit length.
Flexible Mechanical Elements in Practice
Flexible mechanical elements are employed widely in today's industries for their ability to adjust to different functions without the need for traditional articulate systems. These elements provide a foundation for advancing technology through adaptable design principles.
Flexible Mechanical Joints: Basics and Functions
Flexible joints replace conventional hinged joints and are crucial in a variety of machines. They act as approximately linear elastic connections that can rotate within certain limits.Key functions of these joints include:
- Improved Motion Transfer: Ensures smooth transmission of movement between parts.
- Increased Durability: Fewer mechanical stresses lead to longer lifespan.
- Versatility: Suitable for applications requiring various movement ranges.
In applications like microelectromechanical systems (MEMS), flexible joints help miniaturize complex mechanical systems. This miniaturization enables advanced functionalities in a compact form, which is crucial for sensitive applications such as biomedical implants and consumer electronics.
Flexible Mechanical Couplings: Types and Uses
Flexible mechanical couplings connect rotating shafts while accommodating misalignment and shaft movements. They play a key role in ensuring machine efficiency.Common types include:
- Jaw Couplings: For general-purpose applications requiring poor alignment compensation.
- Oldham Couplings: Useful in scenarios demanding angular misalignment support.
- Bellows Couplings: Ideal for precise torque transmission without backlash.
A practical use case of bellows couplings is seen in precision linear motion applications, such as CNC machines, where their capacity to provide flexible alignment ensures high positional accuracy.
Choosing the right type of flexible coupling depends not only on alignment needs but also on load capacity and environmental conditions.
Innovative Flexible Mechanical Elements
Innovation within flexible mechanical elements has vastly expanded their application scope. Materials such as shape-memory alloys and biomaterials enable these elements to perform in dynamic conditions.The integration of smart materials has led to the development of mechanisms that can self-adjust based on external stimuli such as temperature or pressure, providing real-time adaptive performance.
A shape-memory alloy is a material that “remembers” its original shape and, after being deformed, can return to its pre-deformed shape when heated.
The field of bio-inspired flexible mechanisms has seen exciting developments, modeling elements after biological systems to improve adaptability and efficiency. Examples include robotic limbs that mimic cephalopod tentacles, allowing for highly flexible and versatile applications across various environments.
flexible mechanisms - Key takeaways
- Flexible Mechanisms: Systems in engineering capable of changing shape or function without traditional joints, leading to simpler, lighter designs.
- Flexibility Mechanism Definition: A component that can perform multiple functions by changing configuration without extra articulation methods.
- Applications of Flexible Mechanisms: Used in robotics for flexible grippers, aerospace for adaptive wings, medical devices for body-adaptive instruments.
- Flexible Mechanical Joints: Replace hinged joints; offer improved motion transfer, durability, and versatility in machines.
- Flexible Mechanical Couplings: Connect rotating shafts, accommodate misalignment; types include jaw, Oldham, and bellows couplings.
- Techniques for Designing Flexible Mechanisms: Involves selecting materials like elastomers and metals; geometric considerations like tapered, curved shapes enhance flexibility.
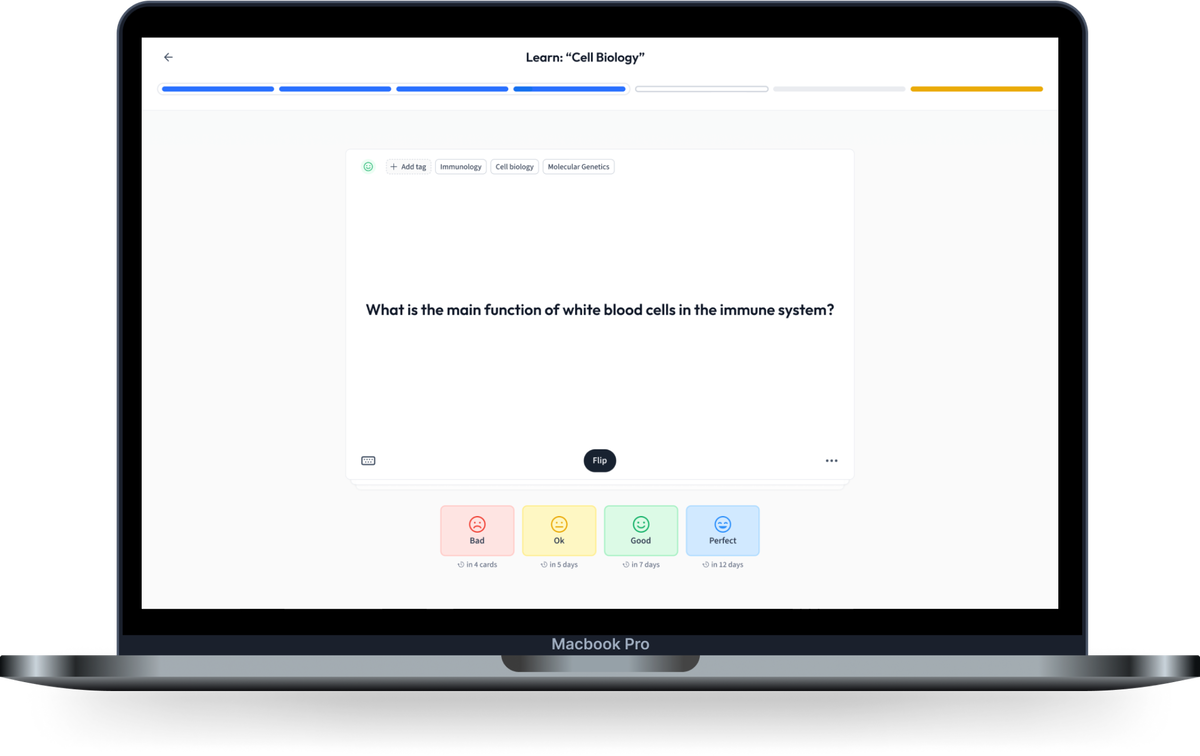
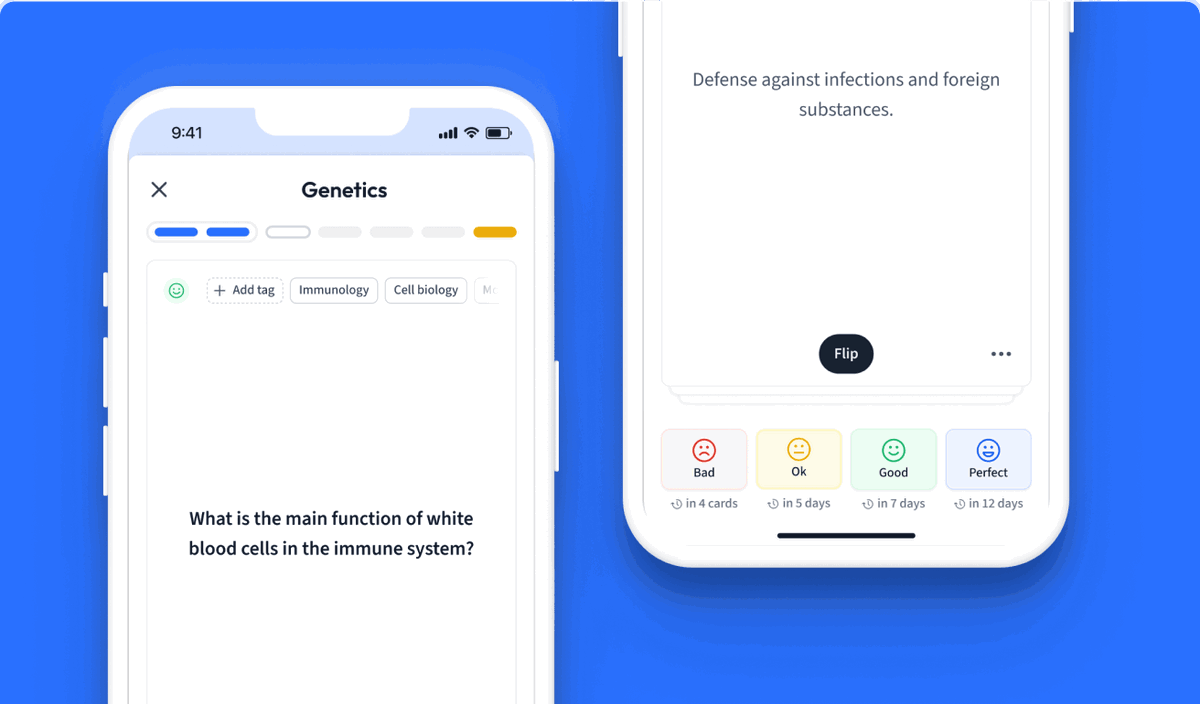
Learn with 12 flexible mechanisms flashcards in the free StudySmarter app
Already have an account? Log in
Frequently Asked Questions about flexible mechanisms
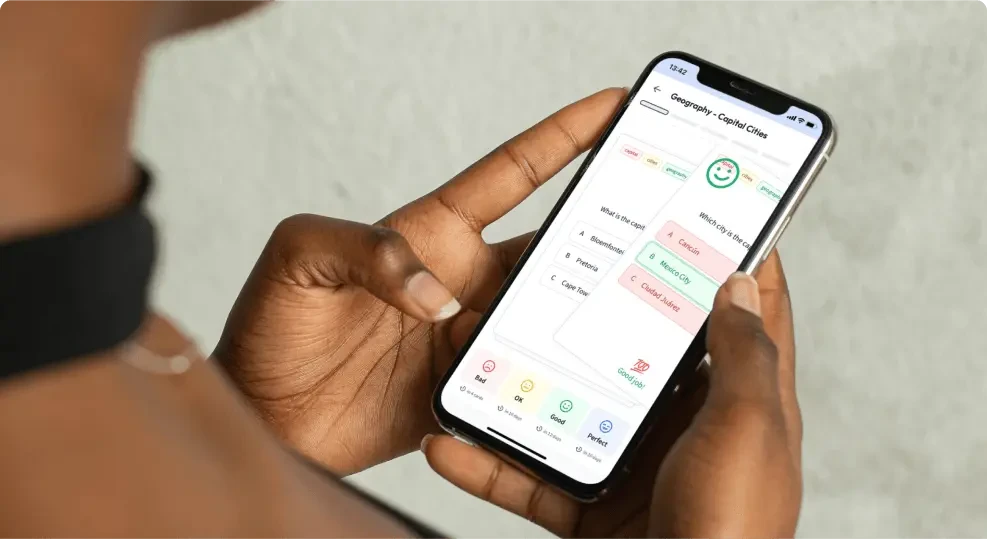
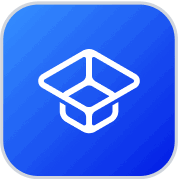
About StudySmarter
StudySmarter is a globally recognized educational technology company, offering a holistic learning platform designed for students of all ages and educational levels. Our platform provides learning support for a wide range of subjects, including STEM, Social Sciences, and Languages and also helps students to successfully master various tests and exams worldwide, such as GCSE, A Level, SAT, ACT, Abitur, and more. We offer an extensive library of learning materials, including interactive flashcards, comprehensive textbook solutions, and detailed explanations. The cutting-edge technology and tools we provide help students create their own learning materials. StudySmarter’s content is not only expert-verified but also regularly updated to ensure accuracy and relevance.
Learn more